Compressed sensing in synthetic aperture photoacoustic tomography based on a linear-array ultrasound transducer
Download: 1053次
Photoacoustic (PA) imaging is a noninvasive imaging modality based on the thermoelastic expansion effect in biological tissues. This imaging tool could provide anatomical, functional, metabolic, molecular, and genetic contrasts from organelles to organs[1–
One of the main concerns in the linear-array transducer based PAT is that this modality suffers from the limited-view challenge[8–
The limited-view problem could be compensated if the synthetic aperture detection configuration is established to encircle the target. The original incomplete detection geometry of the single-array transducer would be effectively extended based on the spatial compounding method[21,22]. However, the synthetic aperture setup would inevitably increase the burden of DAQ hardware. As the number of transducer elements increases, the operation and reconstruction in the ultrasound receiving system become more challenging and costly[23]. Compressed sensing (CS) could recover compressible signals from under-sampled measurements, and thus it is suitable for PA image reconstruction from insufficient measurements[24–
In this Letter, a feasible synthetic aperture PAT configuration based on the CS reconstruction algorithm is proposed. This approach combined the ultrasound spatial compounding method to extend the effective aperture size and the CS technique to reduce the measurement dataset. The theoretical model of the limited-view issue in the linear-array transducer based PAT was first introduced, then the corresponding simulation and phantom studies were conducted to verify the synthetic aperture setup and the proposed CS reconstruction method.
The generation of acoustic waves is resulted from the PA effect in the biological tissue. Through the instant excitation of a short-pulsed laser, a certain proportion of the absorbed energy would be converted into heat, generating a wideband acoustic signal due to the transient thermos-elastic expansion. The mathematical model for the forward problem of PAT in an acoustically homogeneous tissue in the case of thermal confinement is
The pressure
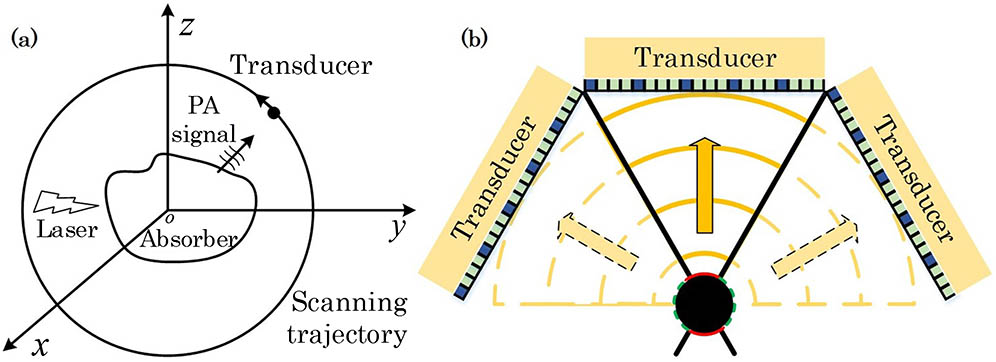
Fig. 1. Theoretical model of the synthetic aperture PAT. (a) Ideal PA signal generation and detection. (b) Synthetic aperture configuration with 1/4 DAQ channels.
Both the PA signal detection and PA image reconstruction assume that the detection trajectory should be an enclosed surface with infinitely small point detectors. This is contradictory to the practical application, where the spatial sampling interval always exists in a single element or an array transducer. An infinitely wide array is demanded to entirely cover the target if linear array is considered. However, in practice, the aperture size of the probe and the penetrated depth of the PA wave are finite and restricted. As a result, the synthetic aperture configuration composed by a single linear-array transducer was proposed and the concept model to detect the artificial blood vessels is illustrated in Fig.
It can be observed that the generated PA signals will only be detected at two opposite orientations of the omnidirectional propagation. So the target boundary located at the real red part can be detected by the transducer, while the green dashed part presents the missing information of the target. The finite aperture size could not collect the entire acoustic signal, leading to the loss of structure information, limited-view artifacts, and image resolution degradation. Here, the tilted angle of the extended aperture is assigned as 60°, considering the target structure, imaging depth, and geometrical symmetry of the field of view (FOV). Thus the three linear-array transducers would form the semicircle cross-sectional geometry to approximate enclosed detection surface and then the multiview data will be collected. However, a multi-element ultrasound transducer and the associated multichannel DAQ electronics will be required to receive the PA signals. Therefore, to reduce the hardware cost and improve data acquisition speed, the CS technique has been applied here to reconstruct an image with fewer measurements. The selected receiving channels in CS reconstruction are shown as the dark blue element in Fig.
CS is suitable for sparse reconstruction from incoherent measurements. The images in PAT are speckle-free and sensitive to the boundaries of the absorbers[29,30]. So the image
In order to approximate the enclosed detection trajectory by only one single-aperture linear-array transducer, the conceptual model of the synthetic aperture PAT was proposed and verified numerically. The K-wave toolbox was chosen for simulation of the forward PA wave propagation and detection. The corresponding PA images were reconstructed by the CS method, with the traditional universal BP as a standard for comparison. The conceptual model of synthetic aperture configuration for imaging the point targets and the artificial blood vessels are illustrated in Figs.
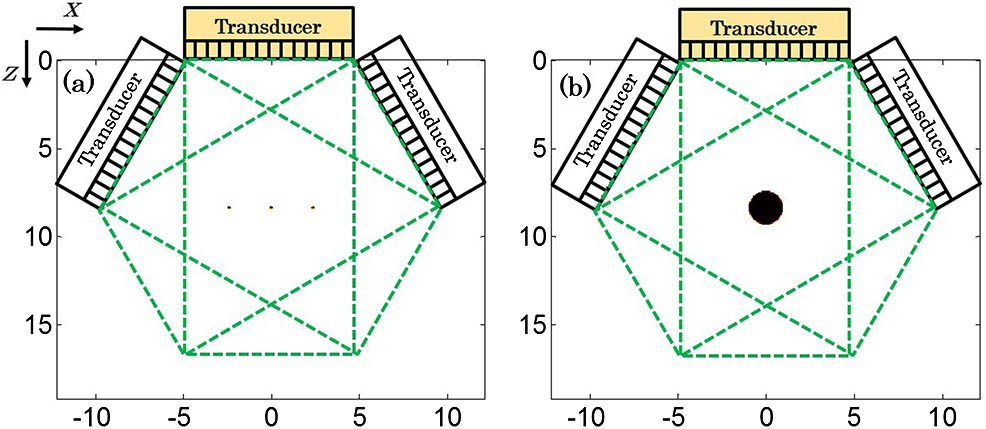
Fig. 2. Conceptual model of synthetic aperture PAT. (a) Image resolution simulation. (b) Limited-view simulation.
The synthetic aperture configuration consists of three linear-array transducers to acquire the PA signals simultaneously. It has 96 elements in total, each single linear array containing 32 directional elements. The pitch in each transducer is 0.304 mm and the center frequency is 7.5 MHz. The pitch size and the element width were maintained the same with the parameter of the transducer used in the following experiment. The properties of the acoustic source in the medium were defined as a spatially smoothed initial pressure distribution, assuming the radiation light was illuminated uniformly at the target.
In the first simulation, a series of point targets was placed to quantitatively measure the resolution determined by the different reconstruction methods. Distribution of the point sources in the synthetic aperture setup allowed for analysis of the beam profile along the lateral axis of the array. The recovered PA images are separately shown in Fig.
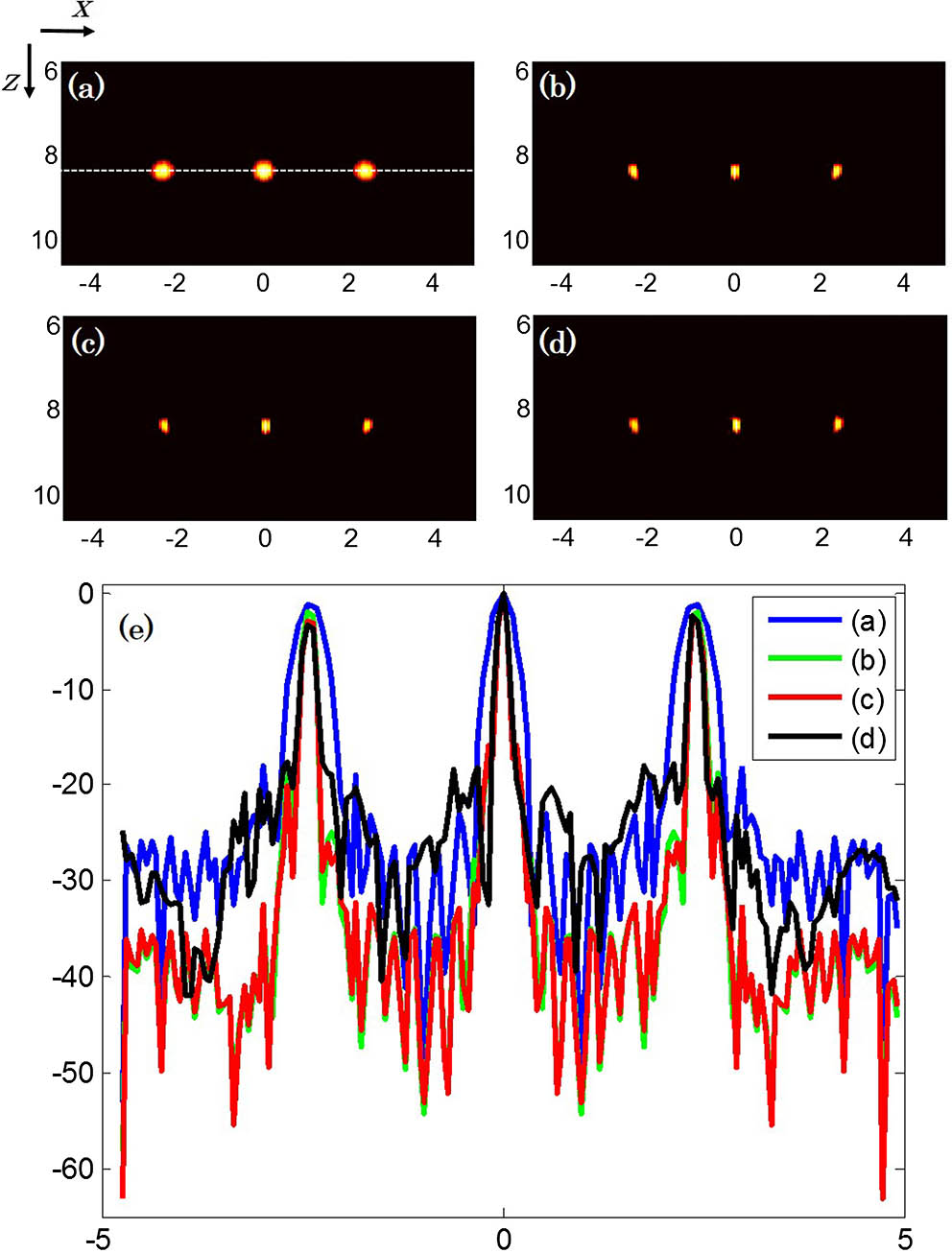
Fig. 3. (Color online) Enhanced resolution simulation results. The PA images reconstructed by (a) BP with center aperture, (b) BP with synthetic aperture, (c) CS with synthetic aperture, and (d) CS with 1/4 synthetic aperture. (e) The profile along the center line on (a)–(d).
It could be obviously seen that the image resolution from the synthetic aperture configuration was enhanced. Compare Fig.
Figures
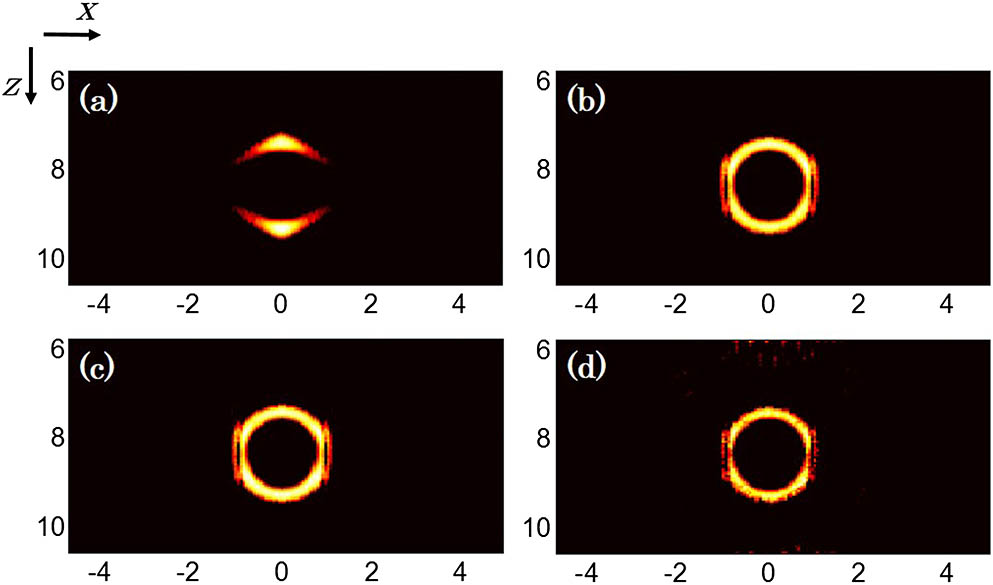
Fig. 4. Limited-view reconstruction results. (a) BP with center aperture, (b) BP with synthetic aperture, (c) CS with synthetic aperture, and (d) CS with 1/4 synthetic aperture.
Compare Fig.
Phantom studies were conducted to validate the feasibility of proposed method subsequently. To geometrically match the configuration in the simulation, the corresponding synthetic aperture setup was formed by rotating a single linear-array transducer, as shown in Fig.

Fig. 5. PA experimental setup using a rotate-translate stage to form the synthetic aperture.
The advantage of this single linear-array ultrasound transducer-based synthetic aperture PAT system is that the detection FOV can be easily adjusted. On the other hand, only one linear-array transducer was chosen to save the hardware resources. The system provides a potential solution for multiview imaging the larger and deeper region of interest in biological tissue, such as peripheral vessels, carotid artery. All the PA images were captured with a 780 nm wavelength and the maximum output laser fluence was limited to

Fig. 6. (Color online) Enhanced resolution experiment results. (a) BP with a center aperture, (b) BP with a synthetic aperture, (c) CS with a synthetic aperture, and (d) CS with 1/4 synthetic aperture. (e) The profile along the center line on (a)–(d).
The relative position between the rotated linear-array transducer and reconstruction FOV should be determined first, especially the rotation center and rotation radius. These two parameters were determined by the aperture size of the transducer and controlled by the precise rotation platform. The rotation radius was 32.98 mm and the rotation center was located at the overlapped point of the three different views. The different rotating orientations were
Figures
After the calibration of this synthetic aperture system, a phantom experiment was performed. The aim is to test the feasibility of the synthetic aperture and CS method in tracing the vessel phantom. A polyethylene tube (inner diameter: 3.0 mm) filled with ICG (Indocyanine Green)was designed as the imaging target. This tube was embedded in the agar phantom at about 32.00 mm depth from the surface of the transducer. The light was distributed uniformly anda linear-array transducer was set as the predefined synthetic aperture to collect the PA data. The PA image in Fig.
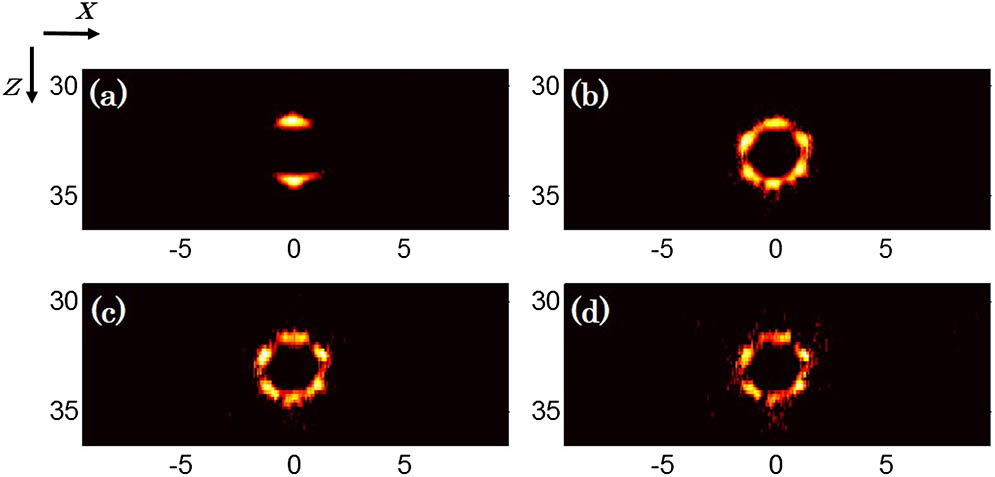
Fig. 7. Limited-view reconstruction results. (a) BP with center aperture, (b) BP with synthetic aperture, (c) CS with synthetic aperture, and (d) CS with 1/4 synthetic aperture.
In conclusion, a synthetic aperture PAT configuration based on the CS reconstruction algorithm is proposed to improve the image resolution and address the limited view problem with fewer number of measurements. The detection coverage is enlarged due to the synthetic aperture and data acquisition channels are reduced due to the CS method. The theoretical analysis, simulation and phantom studies demonstrate that the PA image can be faster reconstructed with fewer channel data to achieve the nearly full-view image quality. In the current study, these off-line data processing methods just focus on recovering the satisfactory PA image quality with reduced measurements. In the future study, real time clinical feasibility for peripheral vessels and human breast imaging will be further investigated.
[1]
[2]
[3]
[4]
[5]
[6]
[7]
[8]
[9]
[10]
[11]
[12]
[13]
[14]
[15]
[16]
[17]
[18]
[19]
[20]
[21]
[22]
[23]
[24]
[25]
[26]
[27]
[28]
[29]
[30]
Xiangwei Lin, Naizhang Feng, Yawei Qu, Deying Chen, Yi Shen, Mingjian Sun. Compressed sensing in synthetic aperture photoacoustic tomography based on a linear-array ultrasound transducer[J]. Chinese Optics Letters, 2017, 15(10): 101102.