基体取向对激光熔覆修复区域杂晶形成的影响
下载: 893次
Objective Renovating surface defects of Ni-based single crystal superalloy blades is the key to prolong their manufacturing life, and repair mechanism is also a hot research topic in material physics and chemistry. In this study, laser melting method combined with theoretical model calculation is used, and the self-developed DD series Ni-based superalloys in China is taken as the research object. On the basis of previous studies, the substrate orientation has rotated 45°around the direction of [010] during laser melting of single crystal alloys, then rotate different angles ξ along the direction of [001], the distribution and variation of different crystal orientation regions with the increase of ξ are studied. It is found that with the increase of ξ, the distribution of single crystal domain increases on the side of molten pool. Substrate orientation 112/[201ˉ] with single crystal region on one side of molten pool is obtained. Repairing operation on its substrate, because of the disappearance of [010] domain in the molten pool of epitaxial growth repair zone, the grain boundary [001]/[010] also disappears, the ability of stray grain formation is greatly reduced. The substrate orientation suitable for laser cladding repair is obtained, and the mechanism of the effect of changing substrate orientation on the formation ability of stray grain in the epitaxial growth structure is demonstrated.
Methods In our previous research, the mechanism of substrate orientation for the formation of equiaxed crystals has been elaborated in detail. It is defined that when the laser scans along the [100] direction on the crystal plane, that is, the substrate orientation relationship is the initial substrate orientation. When the initial matrix is rotated 45° around the crystal direction (i.e., the y-axis), the growth of heterocrysts can be effectively suppressed. On this basis, this study continues to take the crystal direction as the rotation axis. Through the second rotation of different ξ and laser melting technology, the mechanism of inhibiting the growth of heterocrystals is revealed, and the optimal substrate orientation is obtained to effectively control the single crystal nature of the repaired area.
Results and Discussions According to the experimental results, for the initial substrate crystal orientation (001)/[100], the value of the single-layer repair height is limited by the position of the [001]/[100] crystal zone boundary in the laser cladding epitaxial growth structure, and the position of the [001]/[100] crystal zone boundary in the weld pool is related to the initial substrate crystal orientation. For the matrix (101)/[101]ˉ crystal orientation rotated 45° around the y-axis, as shown in Fig. 2 (a) and Fig. 2 (b). In this case, because there is no crystal zone boundary in the epitaxial growth structure by laser cladding, the height can be effectively raised in the single-layer repair. However, the monocrystal property of the repaired structure is affected by the existence of the sum zone on the left and right sides. However, when the substrate with ξ=45° is used (as shown in Fig. 10), due to the disappearance of the [010] crystal zone in the weld pool of the epitaxial growth repair structure, the intersection line of the [001]/[010] crystal zone on one side of the corresponding molten pool is generated, and all the crystal regions are [001] crystal regions on one side of the molten pool, so the substrate orientation with only a single crystal zone can be obtained. Based on the above matrix orientation combined with multi-channel and multi-layer laser cladding experiment, the single crystal structure of laser cladding repair can be obtained completely, which plays a great role in improving the single crystal property of laser cladding epitaxial growth structure.
Conclusions In this paper, we propose a laser cladding repair method for the secondary rotating matrix, and obtain the substrate orientation suitable for the growth of single dendrite direction. The specific conclusions are as follows:
1. In the experiment of laser cladding epitaxial growth and repair, the choice of the initial substrate orientation can affect the ability of heterocrystal formation in cladding epitaxial growth structure under the condition of other repair parameters such as laser parameters, scanning speed and so on.
2. When the initial crystal direction rotates 45° around the y-axis, the crystal direction is rotated by the rotation axis (secondary rotation). When ξ=45°, the laser cladding repair process is carried out along the crystal direction on the surface. The single-layer repair height increases and the single-layer dendrite growth direction is ensured on one side of the weld pool, so as to ensure the monocrystalline of the laser cladding epitaxial growth structure.
3. The internal mechanism of this situation is that after changing the initial crystal substrate orientation, the crystal area in the laser melting pool expands and occupies the area where the original crystal zone is located, and the boundary of the crystal zone disappears in the molten pool. Therefore, a large single crystal area can be adopted, which ensures the repair efficiency and single crystal property of the laser cladding epitaxial growth structure.
1 引言
镍基单晶高温合金具有优异的高温力学性能,是制造航空发动机涡轮叶片的主要材料。随着航空发动机的发展,涡轮叶片的服役环境更加严峻,叶片直接受到高载荷交变应力以及高温(1000 ℃)高压(1 MPa)且具有腐蚀性气流的冲击作用,使用过程中不可避免地会产生磨损和裂纹等表面缺陷,极大缩短了叶片的使用寿命。由于单晶叶片造价昂贵,直接更换成本较高,因此发展有效的叶片修复技术成为国内外广泛研究的课题。激光粉末沉积技术具有修复精度高、对基体的热影响小、修复性能优异等优势,可定点控制柱状晶外延生长,有望广泛应用于高质量单晶叶片的修复工艺中。但修复过程中可能出现的杂晶会促进凝固裂纹的形成,导致修复失败,因此揭示杂晶的形成机理,精准控制其形成过程是发展激光单晶修复技术的关键[1-4]。
激光熔池内杂晶的形成主要受枝晶生长速度和生长方向上温度梯度分量的影响,改变单晶基体取向会改变外延柱状枝晶的生长方向,进而影响杂晶的形成。David等[5-9]在单晶高温合金激光焊接方面进行了大量的研究,Park等[7]设计了一个巧妙的实验有效说明了基体取向对杂晶形成的影响。激光熔池内的温度场以及凝固条件分布关于熔池中心纵截面对称,因此,选择关于中心纵截面不对称的单晶基体取向进行激光焊接实验,结果表明,杂晶更容易出现在熔池的一侧。原因是基体取向不对称使熔池内一侧枝晶偏离温度梯度的角度高于另一侧,在合适的激光加工参数下,角度较大的一侧超过了柱状晶向等轴晶转变(CET)的临界值,产生杂晶;而角度较小的一侧还未达到CET的临界值,保持了柱状晶形态,验证了基体取向会明显影响熔池中的CET。
Anderson等[10-11]结合三维热传递和液流运动模型,在(100)晶面上选择不同的晶向改变基体取向,更细致地研究了单晶高温合金激光熔凝过程。结果表明,在几个晶区的交界处枝晶角度偏离温度梯度方向最大,温度梯度在这些区域更小,表明CET更容易发生在几个晶区的交界处,熔池整体的转变能力则取决于这些晶区交界的位置和数目。Liu等[12]在单晶基体上采用激光沉积Rene5粉末,分别在熔池纵截面和横截面上改变基体取向。结果表明,在横截面上改变基体取向只会影响柱状晶的竞争生长和生长模式,在纵截面上改变基体取向会影响CET的位置。已有研究通常在某一晶面内改变激光扫描方向或在不同晶面内沿某一固定晶向进行修复,对取向的改变方式研究较少,难以全面描述基体晶向对杂晶形成的作用机制。
针对上述问题,本文提出了一种通过二次旋转基体改变初始单晶基体取向的方法,采用理论计算和实验分析相结合的方式,系统研究了基体取向对激光熔池内杂晶形成的影响;阐述了单晶取向的作用机制,并将基体取向效应扩展到激光粉末沉积过程中,证明了其在单晶修复中应用的可行性。
2 实验设计
2.1 基体取向作用
基体取向对等轴晶形成的作用机制[13-14]如
为了确保修复层与基底具有相同晶体取向且保持良好的单晶性,需避免CET的发生[15]。关于CET的研究,主要控制参数为液固界面前沿的温度梯度G和生长速度V。在实际高温瞬态激光熔凝过程中,G和V不是沿某一特定方向,其值随时间和空间不断变化,因此,在激光熔池内,CET会存在于若干不同晶向但属于单一晶粒的区域,如[001]、[100]、[010]和[010]方向对应的区域。
![不同基体取向下的熔凝结果。(a)取向为(001)/[100];(b)取向为(101)/[101ˉ]](/richHtml/zgjg/2021/48/6/0602110/img_2.jpg)
图 2. 不同基体取向下的熔凝结果。(a)取向为(001)/[100];(b)取向为(101)/[10 ]
Fig. 2. Fusion results under different substrate orientations. (a) Substrate orientation is(001)/[100]; (b) substrate orientation is(101)/[10 ]
![基体取向为(001)/[100]时的激光熔覆结果](/richHtml/zgjg/2021/48/6/0602110/img_3.jpg)
图 3. 基体取向为(001)/[100]时的激光熔覆结果
Fig. 3. Laser fusion result when the substrate orientation is (001)/[100]
2.2 优化基体实验
实验使用的国产第二代镍基单晶高温合金材料DD6具有高温强度高、综合性能好、组织稳定、铸造工艺性能好、生产成本相对较低等优点。将稀有贵金属Re加入DD6单晶,同时改变DD6单晶中其他元素的质量分数,使单晶的力学性得到了一定的提升。DD6镍基单晶高温合金的力学性能已达标且具有良好的组织稳定性和单晶铸造工艺性能,已应用于我国航空发动机。实验主要研究了激光熔覆外延生长过程中通过改变基体取向改善组织单晶性的可行性,因此采用常见的高温合金GH738粉末作为激光熔覆实验的粉末,具体化学成分如
表 1. DD6和GH738中元素的质量分数
Table 1. Mass fraction of the elements in DD6 and GH738 unit: %
|
实验使用的基体样品从DD6单晶棒状材料上切取,底面直径φ=15 mm。定义激光在(001)晶面上沿[100]方向扫描即(001)/[100]基体取向关系为初始取向,将初始基体绕[010]晶体方向旋转45°,再将[001]晶体方向作为旋转轴,分别旋转角度ξ=0°,15°,30°和45°并进行切片后将样片作为基底,如
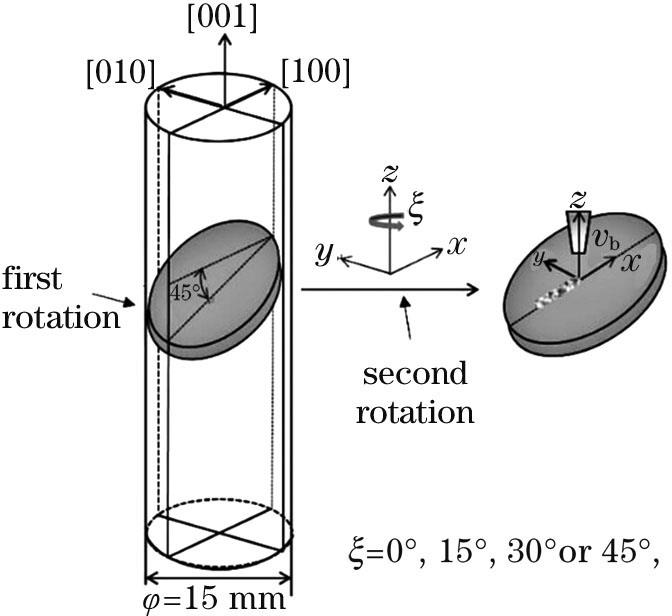
图 4. 二次旋转得到的基体取向示意图
Fig. 4. Schematic diagram of the substrate orientation obtained by the second rotation
实验使用最大功率为1 kW的Nd∶YAG连续固体激光器,激光功率为200 W,扫描速度为5 mm/s,熔覆层粉末的颗粒度为300目。送粉器为单筒侧向送粉装置,送粉速率为8 g/min。单层提升高度为200 μm,横向叠加率为50%,送粉气体为惰性气体Ar,具体装置如
3 分析与讨论
3.1 实验结果
在初始基体取向(001)/[100]的基础上,将基体绕y轴旋转45°且ξ=0°时的激光熔覆熔池结果如
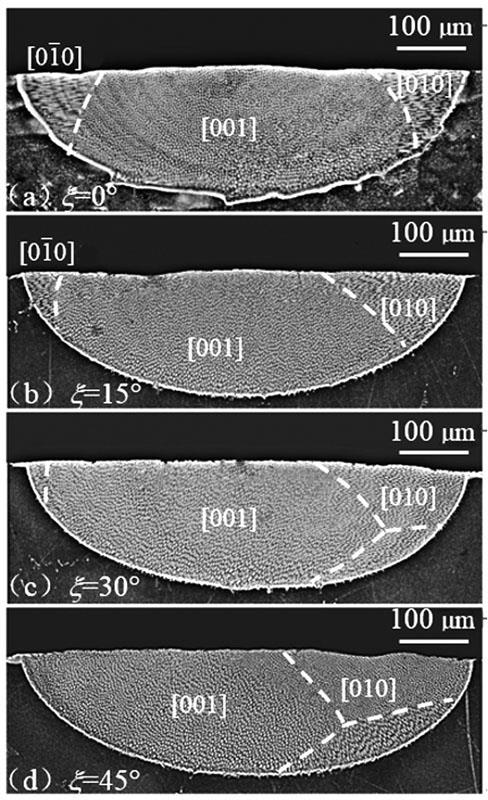
图 6. 扫描速度5 mm/s时得到的激光熔凝熔池结果。(a) ξ=0°;(b) ξ=15°;(c) ξ=30°;(d) ξ=45°
Fig. 6. Laser melting pool results obtained at a scanning speed of 5 mm/s. (a)ξ=0°; (b) ξ=15°; (c) ξ=30°; (d) ξ=45°
3.2 激光熔池内CET的发生机制
激光熔凝过程中,除了在熔池底部和熔池边缘很小的区域内凝固速度接近0 m/s外,熔池中的凝固速度大约为10-3~10-1 m/s,液固界面温度梯度大约为105~107 K/m。研究表明,G/V的值对CET起着关键作用。当某一区域发生变化时,与其对应界面的生长速度及温度梯度都会发生相应的变化。为了全面阐明基体晶向对杂晶形成的作用机制,得到单晶性良好的外延生长组织,必须分析相应区域的CET性能。
为了预测CET随外界处理条件的变化,Hunt[21]依据经典的凝固理论提出CET的确定性模型,假设熔体液相成分不变的情况下,组织发生CET时温度梯度G满足
式中,N0为单位体积的形核数,ΔTn为形核过冷度,Ttip为枝晶尖端过冷度,与凝固速度的关系为V=ΔTtipn/a,a和n为与合金成分有关的常数。将ΔTtipn代入(1)式,得到
Hunt[21]首先用解析模型描述稳态柱状晶和等轴晶生长,定性揭示了合金成分、形核密度和冷却速率对CET的影响,
根据Rappaz等[24-33]提出的几何模型,以晶体择优方向为坐标轴建立坐标系(晶向坐标系),以x、y、z轴方向作为坐标轴建立坐标系(熔池坐标系),两个坐标系相对的取向关系可通过相应的旋转矩阵描述,该旋转矩阵也可以转换为矢量在不同坐标系内的表达形式,其对应的三维旋转矩阵可分解为三个基元旋转矩阵的组合形式,可表示为
式中,ξx、ξy、ξz分别为绕x轴、y轴、z轴方向旋转的角度,对应的旋转矩阵可表示为
择优生长方向[hkl] 是激光扫描方向对应的晶向指数,其单位矢量在熔池坐标系中可表示为
通过坐标变换矩阵,
结合基体取向和局域凝固速度,得到不同择优取向方向上的枝晶生长速度为
式中, Vhkl为择优生长方向的速度,根据最小生长速度准则,几个不同生长方向柱状晶相互竞争,总是选择生长速度最小的柱状晶,枝晶的生长速度Vd可表示为
确定枝晶生长方向后,温度梯度沿枝晶方向的分量Gd为
式中,Gsl为固液界面的温度梯度。在将基底绕y轴旋转45°的基础上,以z轴为旋转轴转动角度ξ,考虑ξ增大时枝晶的生长速度及不同晶向区域分布,对于某一固定的ξ,θ和ψ两个方向对应熔池内的各个点,分别为熔池上表面的对应位置和熔池底面熔化线对应的角度位置,根据|Vhkl|/Vb,就能得到理想熔池中枝晶生长速度的分布及枝晶生长的不同晶向区域。ξ为0°、15°、30°和45°时的计算结果如

图 8. 不同基体取向下的熔池分布。(a) ξ=0°;(b) ξ=15°;(c) ξ=30°;(d) ξ=45°
Fig. 8. Melt pool distribution under different substrate orientations. (a) ξ=0°; (b) ξ=15°; (c) ξ=30°; (d) ξ=45°
由于晶区的交点和交线是杂晶形成的敏感区域,基体取向的变化会影响熔池整体平均的杂晶形成能力,当基体取向绕x或z轴旋转时,总是一个交点靠近熔池中心,另一个靠近熔池边界,导致熔池内一侧杂晶形成能力增强而另一侧减弱,两侧的变化相互抵消,熔池整体的杂晶形成能力基本不变;而绕y轴旋转取向时,熔池内的两个交点会同时往熔池顶部或者底部移动,两侧的杂晶形成能力同时增加或者减少,不会相互抵消,如
采用激光熔凝实验验证该基体取向效应,然后将该取向效应应用到单晶激光熔覆修复中,探讨如何利用基体取向效应提高单晶修复的成功率和效率。
单晶激光熔池内由于竞争生长会形成几个晶区,在晶区的交点,枝晶偏离热流方向最大,枝晶生长方向上的温度梯度分量较小,导致晶区的交点和交线处杂晶体积分数较高,成为杂晶形成的敏感区域。采用ξ=45°基体取向旋转可以控制熔池内的晶区交点和交线的位置,达到定向控制杂晶形成区域的作用。
激光熔凝实验得到的结果与理论分析的结果一致,在绕[010]轴旋转改变杂晶形成能力的基础上,再将[001]晶体方向作为旋转轴,分别旋转不同角度ξ。以ξ=45°基体取向作为基底,对应的熔池内一侧没有产生[001]/[010]晶区的交线,该侧全部为[001]晶区,可作为完美的激光熔覆修复基体。
对于具有不同初始晶向的基体,在相同激光修复参数条件下的激光熔覆外延生长组织中,ξ=45°基体的杂晶形成能力远远小于初始基体,这表明相对于初始基体,旋转过后的基体晶向更适用于激光熔覆外延生长修复操作。通常情况下,镍基单晶高温合金叶片是沿[001]轴线生长铸造的,因此修复工作一般是在(001)晶面进行。如果一个单晶叶片的表面出现缺陷,那么通常是对缺陷部位进行整体切除,留下一个平整的(001)晶面,这种情况就是初始展示的基体晶面(001)以及扫描方向[100],然后在该平面上展开激光熔覆修复操作。这种情况下,杂晶容易在[001]/[010]晶区交界线处出现,因此要求新一层的激光熔覆外延生长组织必须把前面一层熔覆外延生长组织内存在的[001]/[010]晶区交界线全部重熔掉,以保证外延生长组织的单晶性。
3.3 基体取向对激光熔覆外延组织生长过程中杂晶形成的控制机理

图 10. 基体取向ξ=45°时的激光熔覆实验。(a)实验结果;(b) EBSD测试图
Fig. 10. Laser cladding experiment when the substrate orientation ξ=45°. (a) Experimental results; (b) EBSD test chart
综上所述,对于采用(001)/[100]晶向的初始基体,其单层修复高度受激光熔覆外延生长组织内部[001]/[100]晶区交界线位置的限制,而熔池内部[001]/[100]晶区交界线的位置与基体初始晶向有关。对于晶向绕y轴旋转45°得到(101)/[10
4 结论
提出了一种二次旋转基体的激光表面熔覆修复方法,获得了适合生长单一枝晶方向的激光熔覆修复操作基体取向并揭示了基体晶向改变对外延生长组织内部杂晶形成能力的影响机制。在激光熔覆外延生长修复实验中,在激光功率、扫描速度vb等其他修复参数受限的情况下,基体初始晶向的选择可以影响熔覆外延生长组织内部的杂晶形成能力。当初始晶向绕y轴旋转45°,再将[001]晶体方向作为旋转轴旋转角度ξ(二次旋转),当ξ=45°时激光熔覆修复进程在
[1] BöllinghausT, HeroldH. Hot cracking phenomena in welds[M]. Berlin/Heidelberg: Springer-Verlag, 2005.
[2] Reed RC. The Superalloys: fundamentals and applications[M]. Cambridge: Cambridge university press, 2008.
[3] 陈炳贻. 航空发动机材料的发展[J]. 航空科学技术, 1998, 9(2): 13-15.
[4] Sims CT, Stoloff NS, Hagel WC. Superalloys II[M]. New York: John Wiley & Sons, 1987.
[6] Vitek J M, David S A, Boatner L A. Microstructural development in single crystal nickel base superalloy welds[J]. Science and Technology of Welding and Joining, 1997, 2(3): 109-118.
[10] Anderson T D. DuPont J N, DebRoy T. Stray grain formation in welds of single-crystal Ni-base superalloy CMSX-4[J]. Metallurgical and Materials Transactions A, 2009, 41(1): 181-193.
[11] Anderson T D. DuPont J N, DebRoy T. Origin of stray grain formation in single-crystal superalloy weld pools from heat transfer and fluid flow modeling[J]. Acta Materialia, 2010, 58(4): 1441-1454.
[15] 郭文渊, 王东生, 王茂才. 镍基超合金的Nd-YAG激光熔敷涂层行为研究[J]. 应用激光, 2002, 22(2): 101-104, 154.
[16] 唐林峰, 王楠, 管强, 等. 单晶合金激光熔凝过程中晶向对单晶完整性的影响[J]. 物理学报, 2010, 59(11): 7941-7948.
[17] Rong P, Wang N, Wang L, et al. The influence of grain boundary angle on the hot cracking of single crystal superalloy DD6[J]. Journal of Alloys and Compounds, 2016, 676: 181-186.
[20] Li JR, Zhong ZG, Liu SZ, et al. A low-cost second generation single crystal superalloy DD6[C] //Superalloys 2000 (Ninth International Symposium), September 17-21, 2000. Warrendale, PA: TMS, 2000: 777- 783.
[22] Gäumann M, Trivedi R, Kurz W. Nucleation ahead of the advancing interface in directional solidification[J]. Materials Science and Engineering:A, 1997, 226/227/228: 763-769.
[24] Rappaz M, David S A, Vitek J M, et al. Development of microstructures in Fe-15Ni-15Cr single crystal electron beam welds[J]. Metallurgical Transactions A, 1989, 20(6): 1125-1138.
[27] Mokadem S. Bezen{c}on C, Hauert A, et al. Laser repair of superalloy single crystals with varying substrate orientations[J]. Metallurgical and Materials Transactions A, 2007, 38(7): 1500-1510.
[28] Wei Y H, Liu R P, Dong Z J, et al. Stress/strain distributions for weld metal solidification crack in stainless steels[J]. China Welding, 2000, 9(1): 36-41.
[29] Wei Y H, Liu R P, Dong Z J. Development of the model for simulating weld metal solidification cracking in stainless steel[J]. China Welding, 1999, 8(2): 3-5.
[30] Dong H B, Lee P D. Simulation of the columnar-to-equiaxed transition in directionally solidified Al-Cu alloys[J]. Acta Materialia, 2005, 53(3): 659-668.
[31] 李祚, 隋尚, 袁子豪, 等. 高沉积率激光熔覆沉积GH4169合金的微观组织与拉伸性能[J]. 中国激光, 2019, 46(1): 0102004.
[32] 周显新, 辛博, 巩亚东, 等. 扫描方向对变厚度熔覆成形件组织与力学性能的影响[J]. 中国激光, 2019, 46(8): 0802003.
[33] 张金智, 张安峰, 王宏, 等. 微锻造激光熔覆沉积高性能TC4组织与各向异性[J]. 中国激光, 2019, 46(4): 0402009.
Article Outline
荣鹏, 郭嘉琛. 基体取向对激光熔覆修复区域杂晶形成的影响[J]. 中国激光, 2021, 48(6): 0602110. Peng Rong, Jiachen Guo. Effect of Substrate Orientation on Formation of Heterocrystals in Laser Cladding Zone[J]. Chinese Journal of Lasers, 2021, 48(6): 0602110.