Charge distribution into illuminated dye-doped surface stabilized ferroelectric liquid crystal cell
Download: 836次
The mechanisms of the switching of the surface stabilized antiferroelectric liquid crystal (SSAFLC) structure[1] and surface stabilized ferroelectric liquid crystal (SSFLC) structure[2] driven by an optically induced effective electric field
Opposite to the SSAFLC, which exhibits three-state switching, the SSFLC can adopt only two optical states, see Fig.
![Molecular director at the SSFLC can adopt two synclinic structures where the tilt angle θ is defined by an angle between the n⃗ and k⃗ versors. These states are metastable and can exist without any external electric fields as a result of the minimum energy condition for the SSFLC structure at particular boundary conditions. These states [(a) and (b)] are characterized by the different orientation of the optical axes; polarized light passing through the SSFLC can be modulated by switching between these states. When the external field reaches +Esat, the SSFLC layer will be fully switched to the state shown in (b), and the application of the negative field of value −Esat drives the liquid crystal (LC) layer to the state shown in (a).](/richHtml/col/2016/14/10/102302/img_001.jpg)
Fig. 1. Molecular director at the SSFLC can adopt two synclinic structures where the tilt angle is defined by an angle between the and versors. These states are metastable and can exist without any external electric fields as a result of the minimum energy condition for the SSFLC structure at particular boundary conditions. These states [(a) and (b)] are characterized by the different orientation of the optical axes; polarized light passing through the SSFLC can be modulated by switching between these states. When the external field reaches , the SSFLC layer will be fully switched to the state shown in (b), and the application of the negative field of value drives the liquid crystal (LC) layer to the state shown in (a).
In Fig.
Although the standard applications of SSFLC structures demand driving by an electric field, the alternative solutions are possible[2,9
When the superposition of the external electric field (
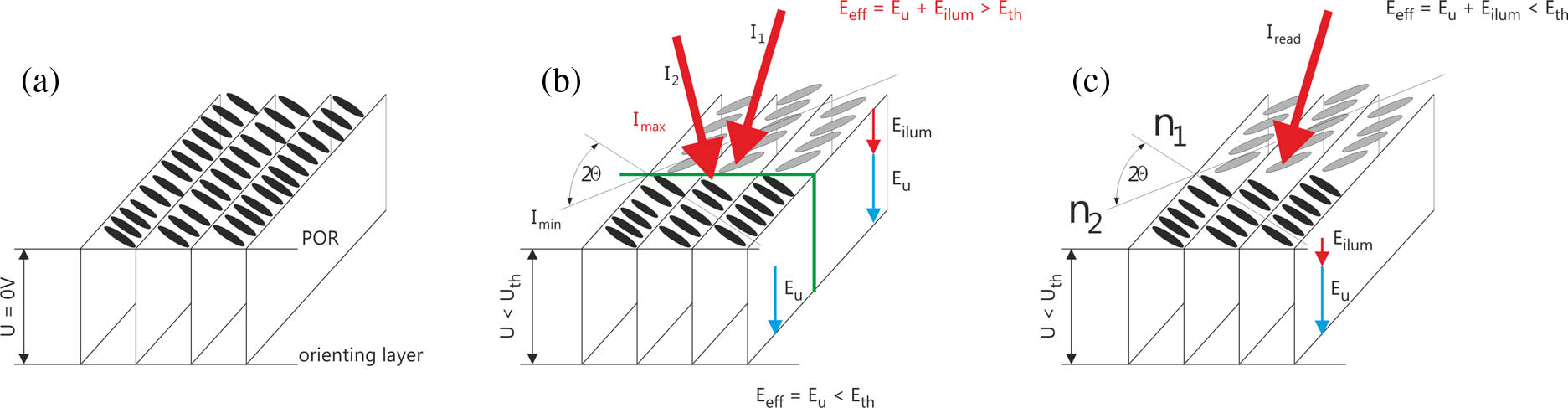
Fig. 2. (a) Creation of the interference gratings onto the SSFLC slab. Initial state of the LC layer in the cell with one of the orienting layer doped by dye (POR). (b) and are the interfering laser beams, The interference pattern consists of neighboring areas with a minimum and maximum intensity of the illumination ( and , respectively), the green line is for show purposes only and roughly denotes a border between these areas. The illumination locally affects the effective electric field ( ) that is superposed with the applied electric field ( ) which exceeds the threshold level ( ) and drives the director locally to an opposite state. (c) The reading laser beam illuminates the SSFLC slab uniformly and when the reading beam intensity ( ), hence , is superposed with does not reach the level, the grating should be ready for optical reconstruction.
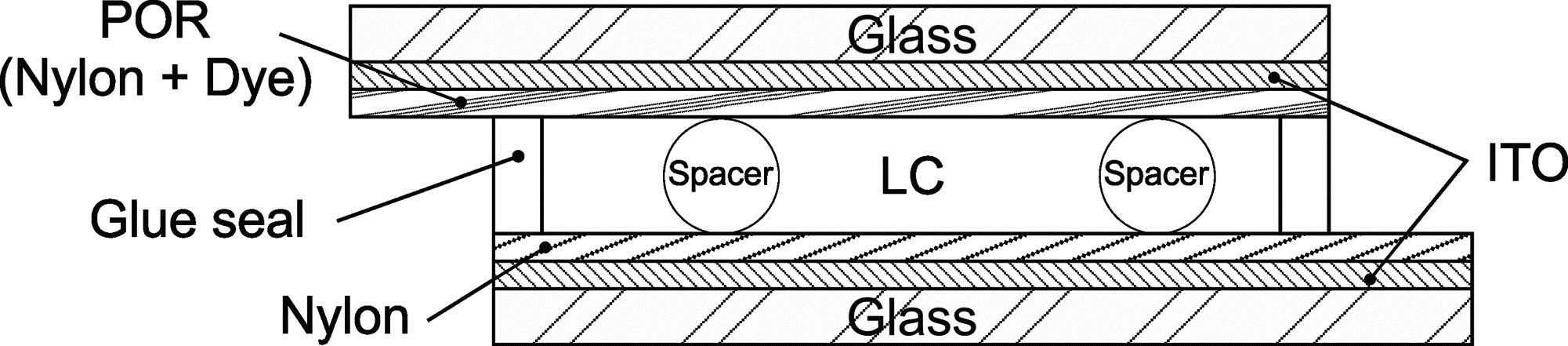
Fig. 3. LC cell with the POR prepared as a mixture of the basic solution (nylon 6/6) and dye-dopant (explanation in text).
The cell has a standard construction with one of the polyimide orienting layers replaced by dye-doped one (POR), shown in Fig.
The cells were prepared using polished soda lime float glass substrates (thickness
The POR was obtained with the surface spin coating of the substrate with the mixture of the basic solution (nylon 6/6) doped with the anthraquinone dye (blue 2590, with a maximum absorption of
Substrate glasses spin coated with basic and dye-doped surface agents were dried and uniformly rubbed according to the standard procedure. The ready-made POR layer thickness was between 85 and 130 nm, according to the measurements done with the use of the ellipsometric method (UV-VIS-IR spectroscopic ellipsometer SE850 by Sentech). Cells with a single photosensitive layer were assembled using one glass substrate with a basic nylon orienting layer and the other with a dye-doped nylon 6/6 one (POR). Both substrates forming a measuring cell were treated with antiparallel rubbing at a controlled regime. To avoid the induction of a two domain structure, an extra cross-rubbing has been applied to the surface of a pure nylon 6/6 orienting layer, according to a custom procedure elaborated at several “try and check” tests. The cells were assembled using spherical glass spacers of a diameter of
The cells have been filled by capillary action with a smectogenic mixture W212-2E at the isotropic state within the vacuum chamber. The mixture of W212-2E used was prepared at the Institute of Chemistry MUT. The uniform orientation of the SSFLC structure was obtained during the slow cooling (
The optical uniformity of the LC structure was checked using of Biolar PI polarizing microscope at room temperature (see Fig.

Fig. 4. Photomicrograph of the SSFLC cell with the POR filled with W212-2E ferroelectric LC observed under the polarizing microscope BIOLAR PI (by PZO Poland) within the birefractive setup. The cross denotes the polarizer and analyzer orientation. (a) Left area is the view of the SSFLC between the ITO electrodes while the electric field is applied. (b) The same cell while the electric field is applied.
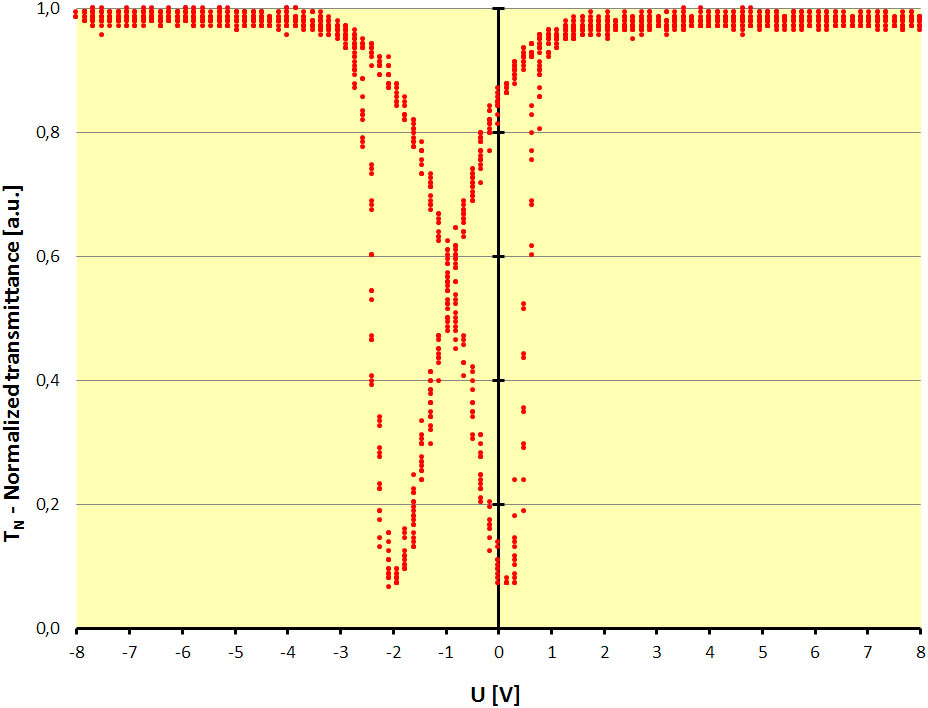
Fig. 5. Electro-optical response of the cell with SSFLC structure (W212-2E mixture) with the orienting layer (nylon 6/6) and the POR (Nylon 6/6 + dye—blue 2590). Measurements were performed for , , and . The cell shows a W-shaped behavior.
The aim of the study was to observe the influence of illumination on the switching process inside the SSFLC structure and the registration of the light generated charge and its distribution. The last one phenomenon is one of the most important at the generation of the phase grating and optical pattern recording within the SSFLC cells[1]. Since a current flow within the cell is proportional to a charge existing and moving through the cell, the last one can be measured by the observation of the current at the time domain. The assumption is that the cell with a photosensitive POR layer can be treated as a complex capacitor with two plate electrodes (ITO and ITO + POR) and dielectric material in between (LC layer), see Fig.
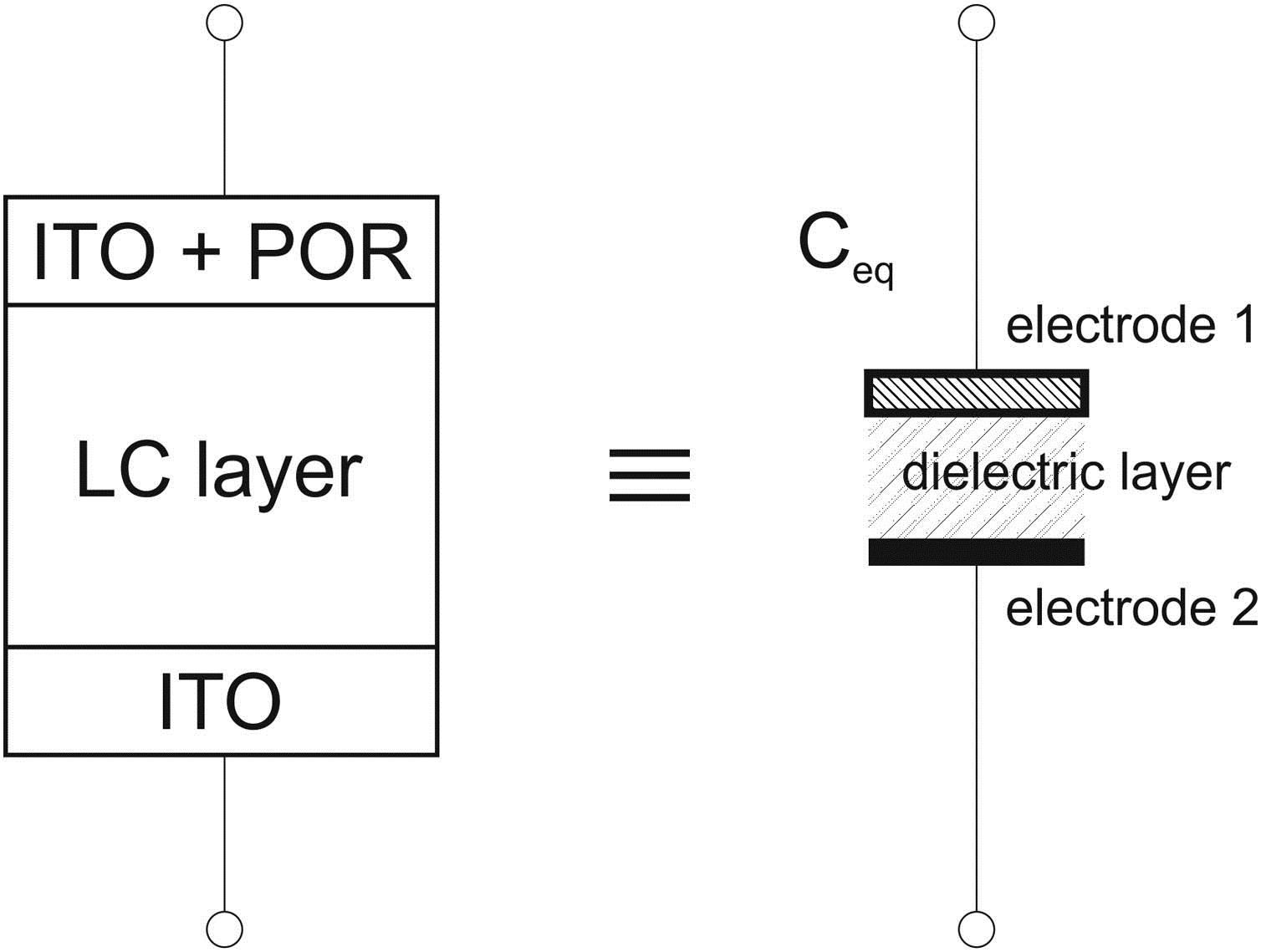
Fig. 6. Equivalent electrical circuit of the photosensitive cell. For clarity, the influence of the electrical properties of the electrical wires and assembled cell (including aligning layers) were omitted and treated as a constant in reference to the assumed conditions.
In the conventional way, the SSFLC structure can be switched as an effect of applied voltage connected to the capacitor’s electrodes. In this case, both plate electrodes can be treated as plates with identical but opposite charges [see Fig.
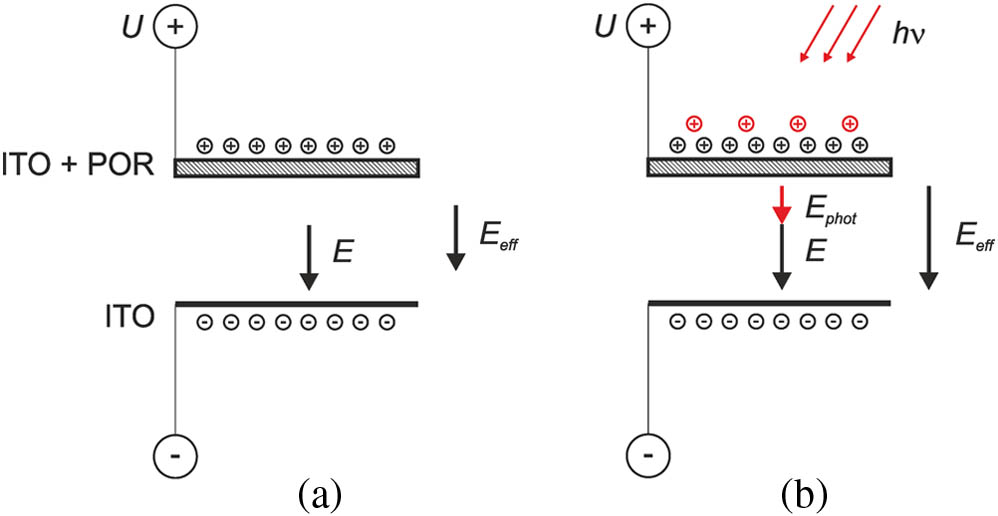
Fig. 7. Charge analysis in the complex capacitor with voltage applied; cases without and with the illuminating photon beam are shown as (a) and (b), respectively.
Within an illuminated cell some additional charges are generated (photo induced carriers) in the photosensitive layer; thus, there exists two different charge densities (
As a consequence, the current flow increases when the cell is illuminated with the photon beam fitted to the absorption bandwidth of the dye used in the POR. Experimental measurements should confirm these assumptions.
The examined SSFLC cell (with the W212-2E mixture) under study was connected to a sensitive electrometer (Keithley 617) and initially polarized with use of a high impedance output of the waveform generator (Agilent 33220A). All the measurements were done in a remote mode by a dedicated application, working under the NI LabView environment. The schematic diagram of the measurement setup is shown in Fig.
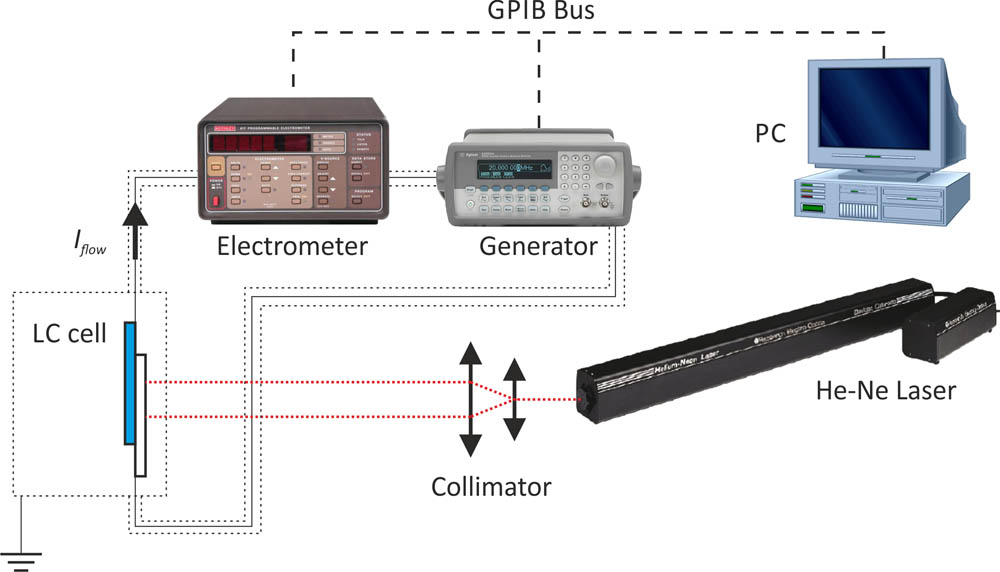
Fig. 8. Measuring setup. A collimated laser beam is applied as an option. All wires and an LC cell under investigation were very precisely shielded by the ground.
The initial measurements were performed on the cell without illumination and no electric polarization. The curve shown in Fig.

Fig. 9. Current flow through the SSFLC structure without the polarizing voltage applied ( ) in darkness. The smoothened curve represents measurements points collected every 0.36 s ( ).
The next step was to determine of a value of the polarizing voltage

Fig. 10. Electro-optical response of the SSFLC structure in a function of the polarizing voltage; the current measurements and results represent the absolute difference between the current flow when the illumination of the cell was switched on or off (“dark–>illum” and “illum–>dark,” respectively). At the level of , a kind of saturation of the observed effect is present (showed by trend lines).
Measurements of the LC cell response under illumination was done for a laser beam power density of approximately
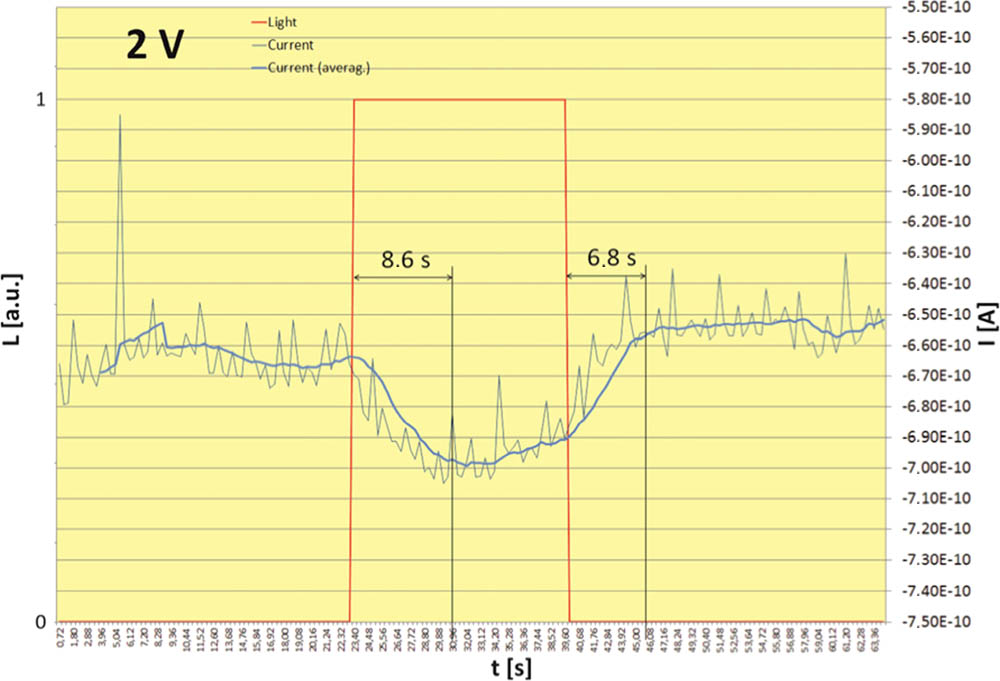
Fig. 11. Measurement of the current flow as an LC cell response for illumination. Measurements were done when was applied to the cell.
To conclude, it can be listed as follows:
[1]
[2]
[3]
[4]
[5]
[7]
[8]
[9]
[10]
[11]
[12]
[13]
[14]
[15]
[16]
Marek Sutkowski, Wiktor Piecek. Charge distribution into illuminated dye-doped surface stabilized ferroelectric liquid crystal cell[J]. Chinese Optics Letters, 2016, 14(10): 102302.