一种光学通道开放且适合构建晶格的静电阱
下载: 565次
1 引言
近年来, 越来越多的研究小组对冷极性分子的操控产生浓厚兴趣。由于具有固有电偶极矩的极性分子在非均匀电场中受到偶极梯度力的作用,所以可以用多种方式, 如利用静电反射镜[1]、静电导引[2-6]、静电分束器[7-8]、静电Stark减速[9-12]、静电存储环[13-16]和三维静电阱[10,17-23]等操控极性分子束。光学通道开放的静电阱可以方便激光探测和操控被囚禁的分子,甚至可以用于冷分子的光学势蒸发冷却[22]。2007年,Schnell等[23]提出了一种针对冷极性分子的半开放交流阱方案。2013年,Wang 等[22]提出了一种光学通道开放的静电阱方案,但考虑到冷分子的装载需要增加两个平板,这样会破坏其光学通道开放的性能。尽管该研究小组又提出了两种改进方案[24-25],但阱中心高度不可灵活操控,而且很难将这些方案移植到芯片表面,尤其不适合用于形成静电晶格。光学晶格可广泛用于研究Sisyphus冷却、偏振梯度冷却与囚禁动力学[26-27]、Raman和绝热冷却[28-29]、拉比振动和布洛赫振荡[30-31]、量子传输和量子隧道效应[32-33]以及通过原子光学晶格的Bragg衍射[34]等。类似地,原子磁晶格也广泛应用于各种磁囚禁行为,波包动力学、量子传输与隧道效应研究以及采用冷原子的量子计算[35],甚至可用于制备一维、二维和三维光子晶体等[36-39]。而关于冷极性分子的静电晶格却几近无人问津。光晶格存在光子散射效应,磁晶格存在导线的冷却问题,相比而言,静电晶格有着自己的优势和巨大的潜在应用价值。
本文提出在双球电极静电阱方案[22]的基础上,通过增加第三个球电极成功解决分子束的装载问题。相比双球电极静电阱方案,新方案继续保持光学通道开放的特性,且阱中心的高度也可灵活操控。此方案具有微型化、集成化、方便移植到芯片表面的特点,可构建一维和二维静电晶格。本文给出了方案模型,推导了电场的解析解,用有限元软件给出了空间电场的数值解;用经典的蒙特卡罗方法模拟了冷极性分子被装载和囚禁的动力学过程,以证实方案的可行性;讨论了此方案在静电表面囚禁方面的应用,如单阱、一维和二维静电晶格。
2 静电阱方案
2.1 公式推导
如
如
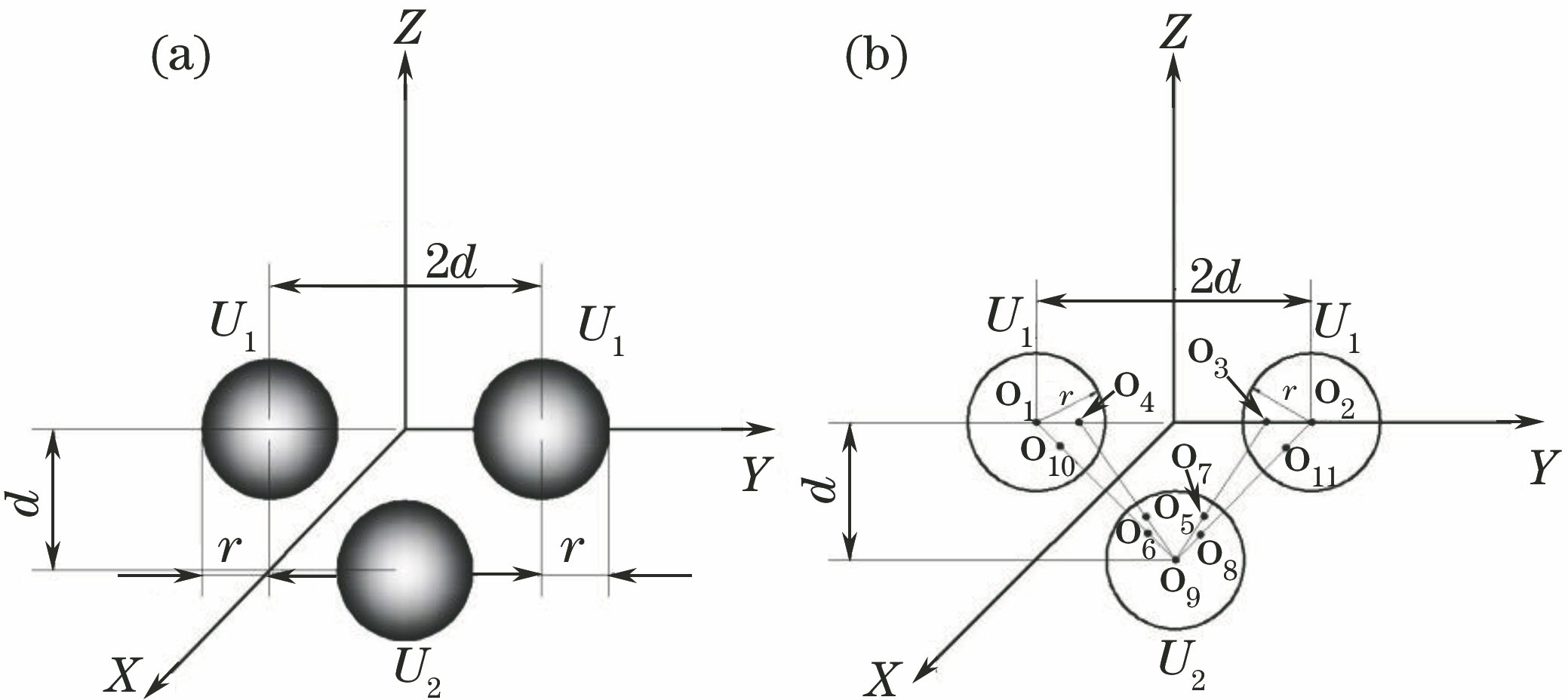
图 1. (a) 三个带电球电极囚禁分子的方案图; (b) 镜像法的原理图
Fig. 1. (a) Schematic for trapping molecules using three charged spherical electrodes; (b) schematic of the image method
表 1. 电荷量和坐标
Table 1. Charges and coordinates
|
由
这里令
式中
2
通过(1)

图 2. 囚禁时YOZ、XOZ、XOY平面的电场等高线分布的解析解和数值解。(a)(d) YOZ平面; (b)(e) XOZ平面; (c)(f) XOY平面
Fig. 2. Analytical solutions and numerical solutions of contour distributions of trapping field at YOZ, XOZ and XOY planes. (a)(d) YOZ plane; (b)(e) XOZ plane; (c)(f) XOY plane
2.3 阱的灵活操控性
通过改变电压
2.4 ND3 分子的装载和囚禁
选择量子态为
式中
如
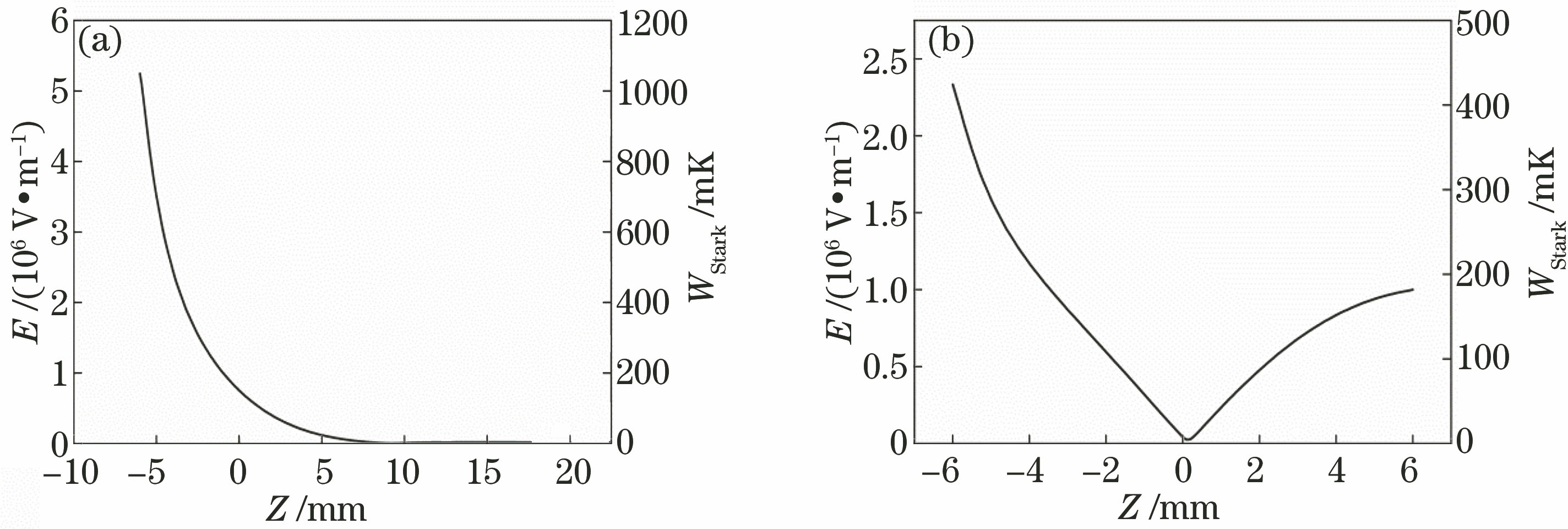
图 4. 沿Z方向的电场分布及对处于量子态|J,K,M>=|1,1,-1>的ND3分子的Stark势能。 (a)装载; (b)囚禁
Fig. 4. Electric field distributions in Z direction and Stark potential for ND3 molecules in state (|J,K,M>=|1,1,-1>). (a) Loading; (b) trapping
2
采用经典的蒙特卡罗法进行模拟。先通过随机数选取得到所需数目且初始位置和初始速度分布均满足高斯分布的初始分子束。在模拟中,所用的分子数目为105。入射ND3 分子束的位置分布和速度分布均为高斯分布,
式中
如
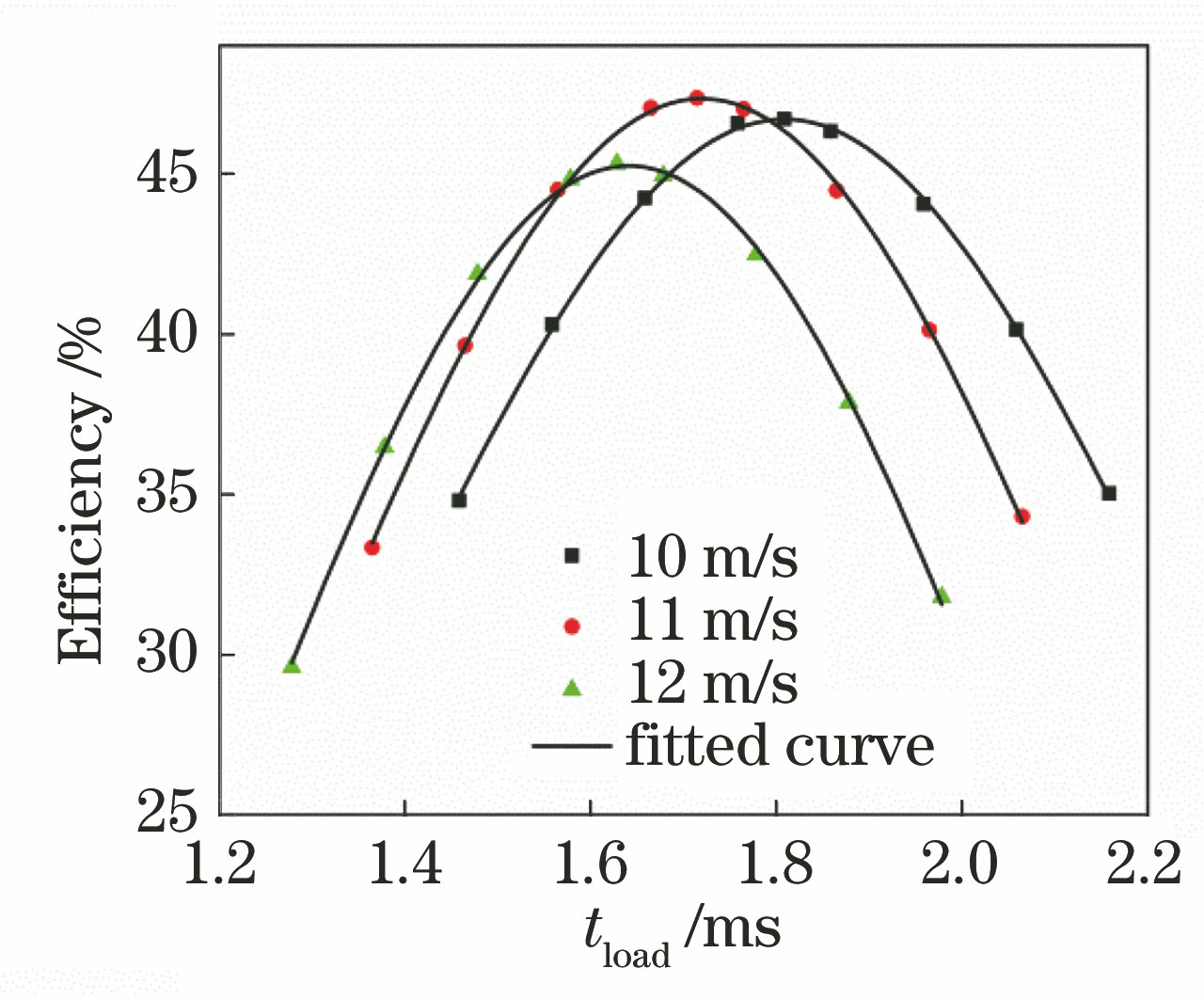
图 5. 在三个不同分子束入射速度下,装载效率与装载时间的关系
Fig. 5. Relationship between loading efficiency and loading time under different velocities of incident molecular beam
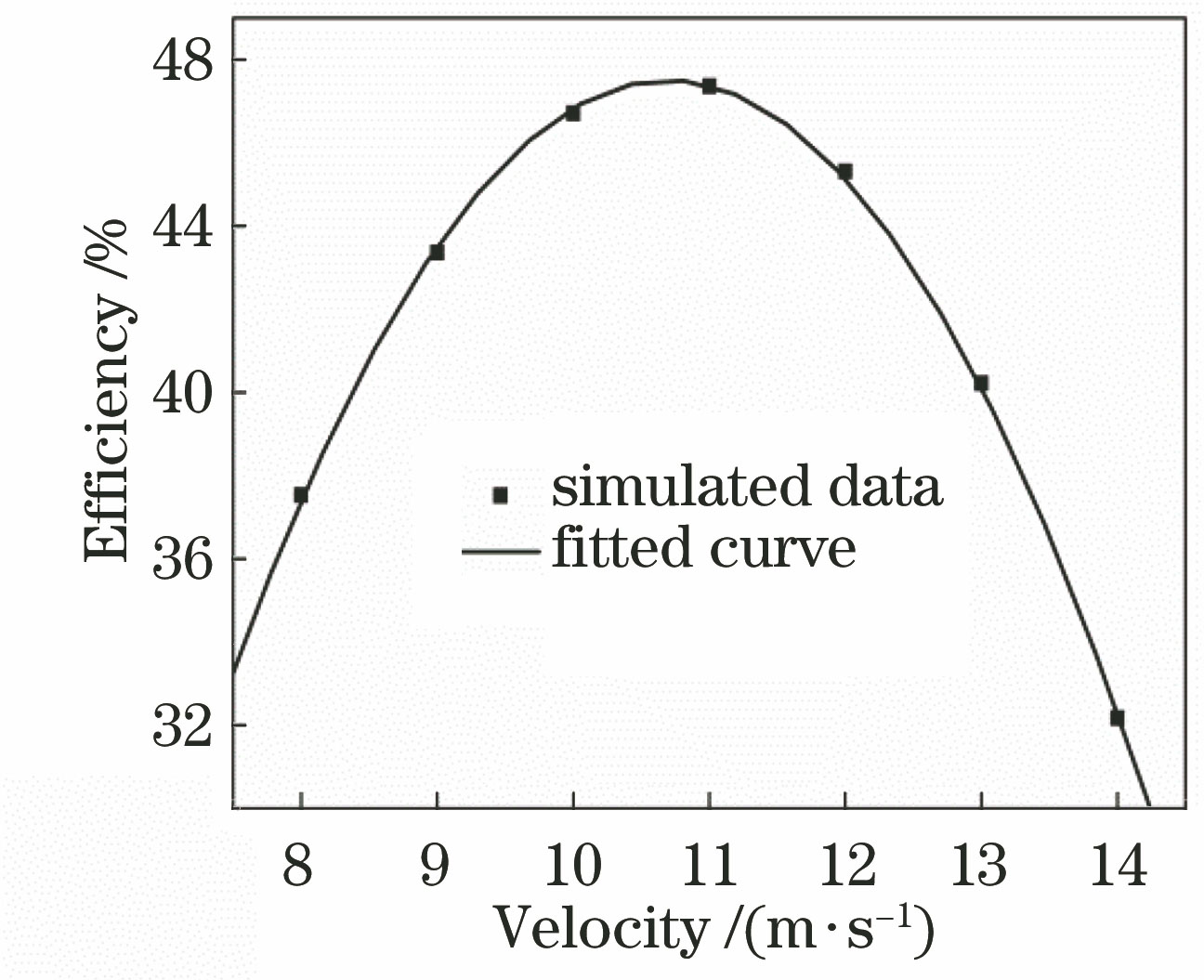
图 6. 装载效率与入射分子束中心速度的关系
Fig. 6. Relationship between loading efficiency and center velocity of incident molecular beam
取入射分子束
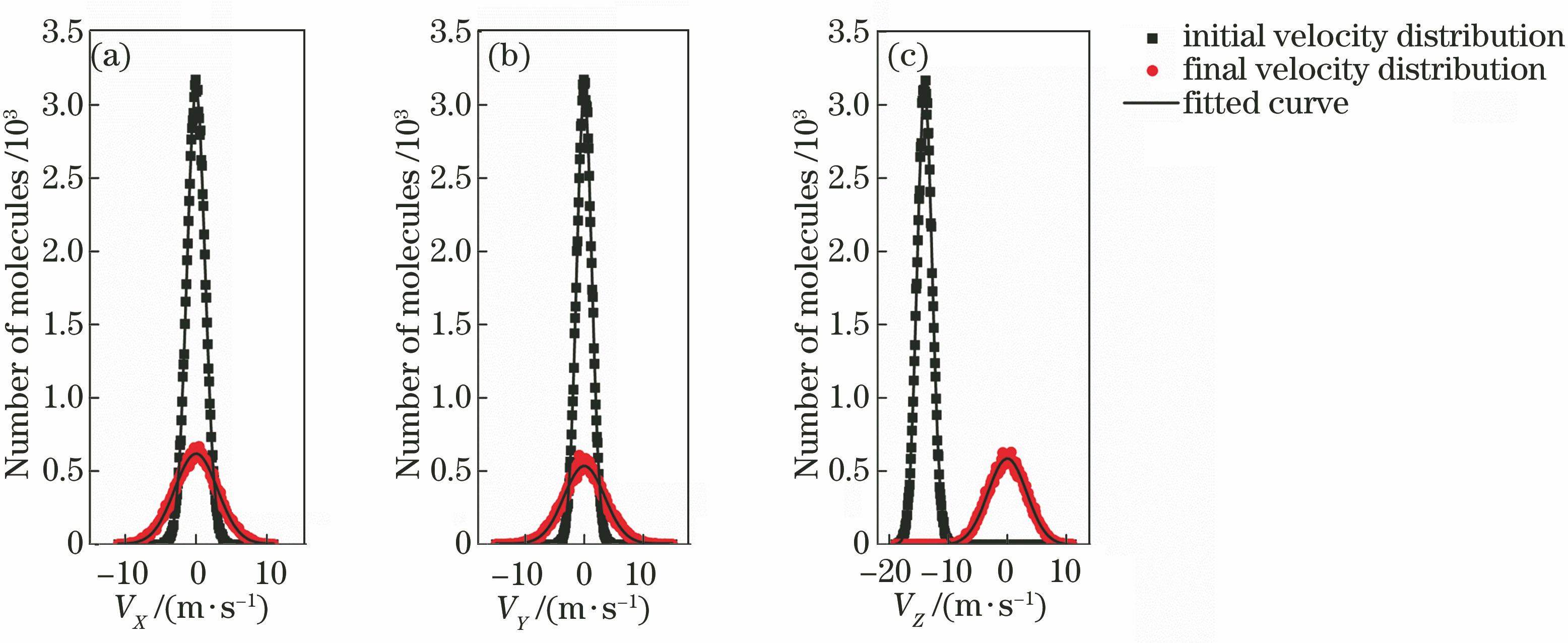
图 7. 入射分子束的初始速度分布及囚禁在阱中的分子速度(VX、VY、VZ)分布。 (a) VX; (b) VY; (c) VZ
Fig. 7. Velocity distributions of incident molecular beam before loading process and the trapped cold molecules. (a) VX; (b) VY; (c) VZ
3 潜在应用与讨论
此方案可延伸性强,下面将三球静电阱方案微型化,并将其移植到芯片表面,以形成静电表面单阱、一维和二维静电晶格等。
3
如

图 8. 用三个带电球电极构建静电表面阱方案图
Fig. 8. Schematic for trapping molecules on a chip with three charged spherical electrodes
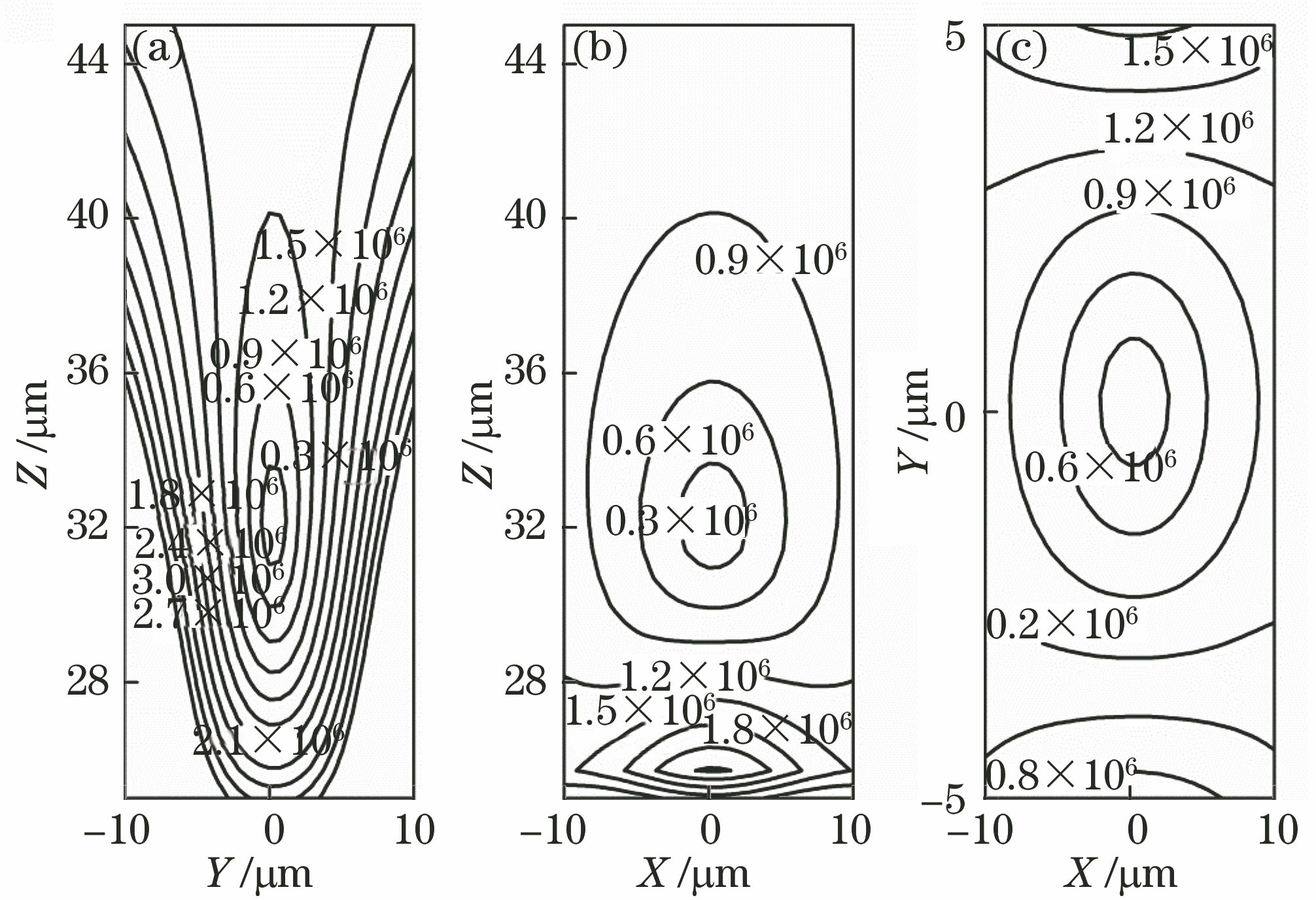
图 9. 囚禁电场的等高线分布。 (a) YOZ平面; (b) XOZ 平面; (c) XOY平面
Fig. 9. Contour distributions of trapping field. (a) YOZ plane; (b) XOZ plane; (c) XOY plane
3.2 一维静电晶格
如果沿着
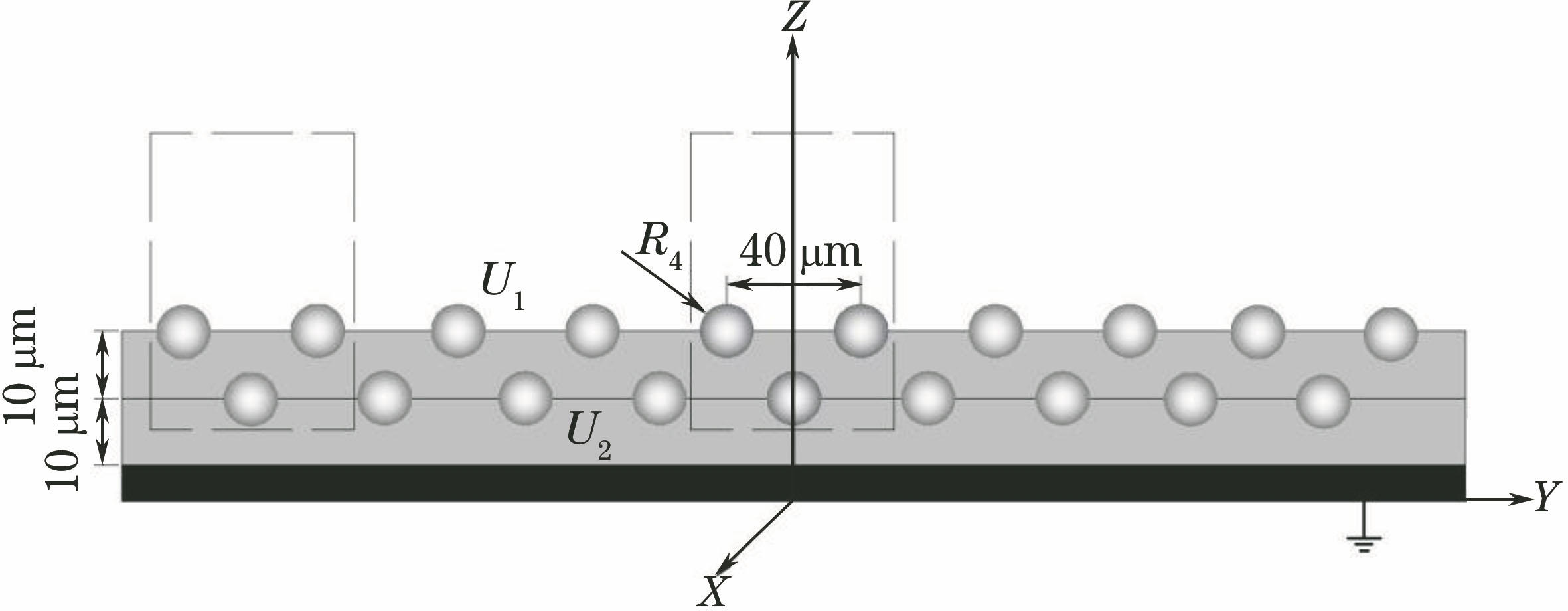
图 10. 一系列三球电极实现在芯片表面对冷分子静电囚禁(一维静电晶格)
Fig. 10. Schematic for trapping molecules on a chip with a series of three charged spherical electrodes (one-dimensional electrostatic lattice)
![在两个不同位置处[图9(a)虚框位置]电场的等高线分布。 (a)(d) XOZ平面; (b)(e) XOY平面; (c)(f) YOZ平面](/richHtml/gxxb/2017/37/12/1202001/img_11.jpg)
图 11. 在两个不同位置处[图9(a)虚框位置]电场的等高线分布。 (a)(d) XOZ平面; (b)(e) XOY平面; (c)(f) YOZ平面
Fig. 11. Contour distributions of trapping field at two different locations [marked with dashed box in Fig. 9(a)]. (a)(d) XOZ plane; (b)(e) XOY plane; (c)(f) YOZ plane
分别为阵列边缘处和中心处三个平面(
3.3 二维静电晶格
同样地,如果将一维静电晶格沿着
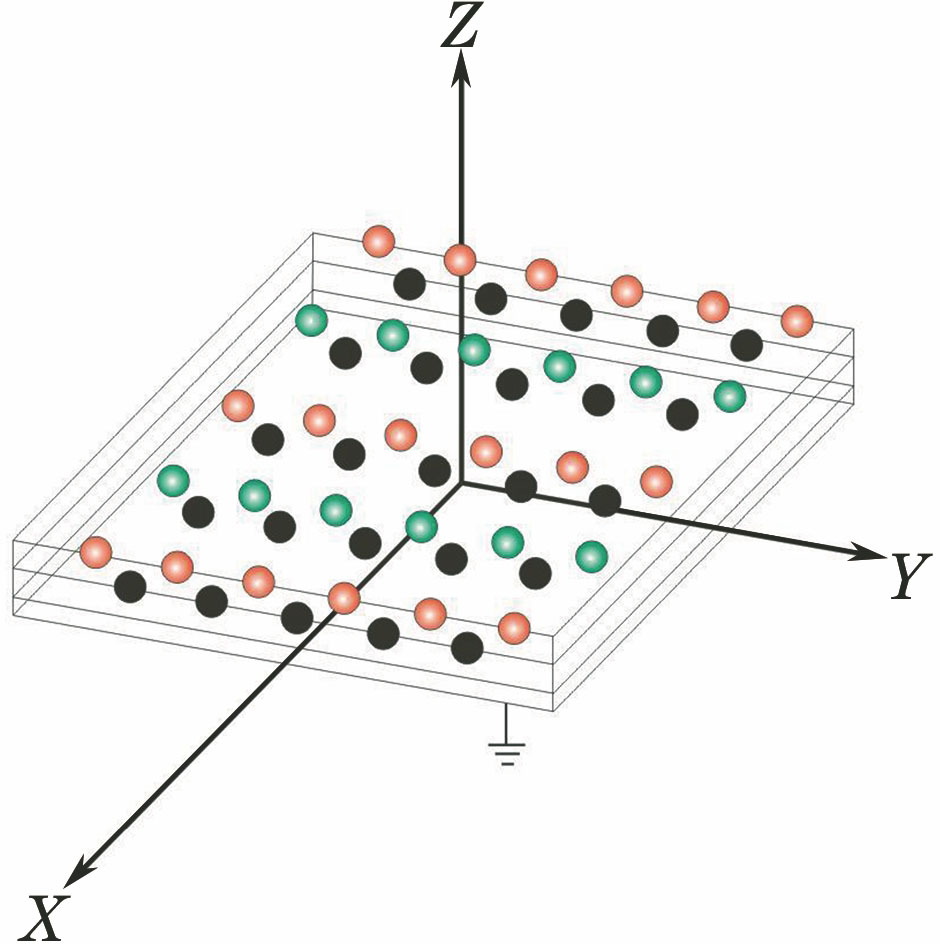
图 12. 利用一系列三球电极在芯片表面实现对冷分子的静电囚禁(二维静电晶格)
Fig. 12. Schematic for electrostatic trapping of cold molecules on a chip with a series of three charged spherical electrodes (two-dimensional electrostatic lattice)
![三个不同位置[图13中虚框位置]电场的等高线分布。 (a) XOZ平面; (b) XOY平面; (c) YOZ平面](/richHtml/gxxb/2017/37/12/1202001/img_14.jpg)
图 14. 三个不同位置[图13中虚框位置]电场的等高线分布。 (a) XOZ平面; (b) XOY平面; (c) YOZ平面
Fig. 14. Contour distributions of electric field in three different locations (marked with dashed box in Fig. 13) . (a) XOZ plane; (b) XOY plane; (c) YOZ plane
4 结论
提出了用三个带电球电极构建一个光学通道开放、针对弱场搜寻态的冷极性分子静电阱,给出了空间电场分布的解析解和数值解。阱中心的高度是可以灵活操控的。通过经典的蒙特卡罗法进行模拟,探究了影响装载效率的因素。计算结果表明:当分子束的初始速度为11 m/s、相应的装载时刻为1.7147 ms时,装载效率最大可达47.4%。将所提静电阱移植到芯片表面, 不仅可以形成静电单阱,而且可以形成一维和二维静电晶格。 借助于囚禁在静电阱中的冷分子,可以研究处于电子激发态、振动激发态的分子寿命。被囚禁的冷极性分子还可用于研究量子信息处理和冷碰撞等。
[1] Schulz S A. Bethlem H L, van Veldhoven J, et al. Microstructured switchable mirror for polar molecules[J]. Physical Review Letters, 2004, 93(2): 020406.
Schulz S A. Bethlem H L, van Veldhoven J, et al. Microstructured switchable mirror for polar molecules[J]. Physical Review Letters, 2004, 93(2): 020406.
[9] Bethlem H L, Berden G, Meijer G. Decelerating neutral dipolar molecules[J]. Physical Review Letters, 1999, 83(8): 1558-1561.
Bethlem H L, Berden G, Meijer G. Decelerating neutral dipolar molecules[J]. Physical Review Letters, 1999, 83(8): 1558-1561.
[10] Bethlem H L, Berden G. Crompvoets F M H, et al. Electrostatic trapping of ammonia molecules[J]. Nature, 2000, 406(6795): 491-494.
Bethlem H L, Berden G. Crompvoets F M H, et al. Electrostatic trapping of ammonia molecules[J]. Nature, 2000, 406(6795): 491-494.
[23] Schnell M, Lutzow P. Veldhoven J van, et al. A linear ac trap for polar molecules in their ground state[J]. Journal of Physical Chemistry A, 2007, 111(31): 7411-7419.
Schnell M, Lutzow P. Veldhoven J van, et al. A linear ac trap for polar molecules in their ground state[J]. Journal of Physical Chemistry A, 2007, 111(31): 7411-7419.
李胜强, 张梦芝, 杨亮亮. 一种光学通道开放且适合构建晶格的静电阱[J]. 光学学报, 2017, 37(12): 1202001. Shengqiang Li, Mengzhi Zhang, Liangliang Yang. Electrostatic Trap Suitable for Construction of Lattices with Opened Optical Access[J]. Acta Optica Sinica, 2017, 37(12): 1202001.