正交再加热双脉冲激光诱导黄连等离子体的光谱特性
下载: 859次
1 引言
目前,对中药材中微量元素如Fe、Ca、Al、Zn等[1]的传统检测分析方法有电感耦合等离子体发射光谱(ICP-OES)法、原子吸收光谱(AAS)法、电感耦合等离子体质谱(ICP-MS)法 等[2-4]。这些方法探测灵敏度高,但样品预处理过程复杂,不能进行实时检测。
激光诱导击穿光谱(LIBS)技术具有多元素在线分析功能,且样品制备过程简单,已经被应用于中药材的元素检测分析中[5-6]。王彩虹等[7]使用LIBS技术快速检测蔬菜中的Ca元素,结果表明,利用LIBS技术能清晰探测出样品中浓度高的元素,但是对于浓度低的重金属,检测灵敏度不足。Yi等[8]采用基于小波变换算法的背景去除的标准加入法,提高了粉末样品中元素的LIBS探测灵敏度,样品中Pb的预测浓度标准偏差提高到97.76%。郭连波等[9]使用LIBS结合空间约束的方法检测钢铁样品中低浓度元素V、Cr、Mn,结果证明该方法可以提高LIBS的探测灵敏度。杨宇翔等[10]采用激光诱导击穿光谱-激光诱导荧光联用技术,将液体样品转换为固体样品,实现了水中重金属元素Pb的超灵敏快速检测。
双脉冲(DP)LIBS技术不仅具有单脉冲(SP) LIBS的优点,还能增强光谱强度,提高LIBS的探测灵敏度[11-12],主要用于分析金属样品[13-18]。目前关于DP-LIBS技术在中药材元素检测中的研究鲜有报道,对于金属样品和有机样品,它们的烧蚀机制也不同[19]。
本文采用正交再加热双脉冲装置,对中药材黄连中的元素进行了检测,分析了探测延时、激光能量值组合、脉冲间隔等参数对再加热双脉冲激光诱导击穿光谱(RDP-LIBS) [20]检测灵敏度的影响。在最优的实验条件下,获得了4条特征谱线Fe I (358.17 nm)、Al I (396.15 nm)、Ca II (393.37 nm)、CN (388.34 nm)的光谱强度增强倍数。对比了SP-LIBS和RDP-LIBS检测中,等离子体电子激发温度和电子数密度随探测延时的变化关系。
2 实验及理论
2.1 实验装置
搭建的正交RDP-LIBS系统如
2.2 实验样品
实验所用的黄连购自重庆中药材市场,如
3 结果与分析
3.1 探测延时对光谱强度的影响
在激光等离子体形成初期,特征谱线的光谱强度和背景噪声都很强,但背景噪声的衰减速度远大于特征谱线的,故可以通过调节ICCD探测延时来
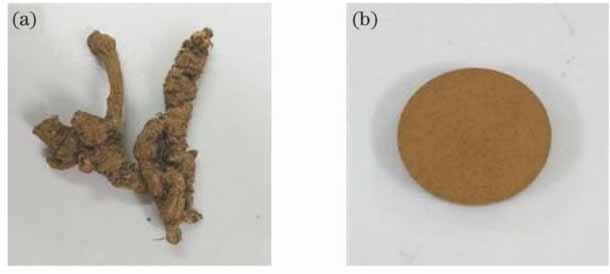
图 2. 黄连。(a) 采购的实物;(b) 制备后的样品
Fig. 2. Coptis Chinensis. (a) Purchased real objects; (b) samples after preparation
观测特征谱线的时间演化特性。设定激光脉冲频率、ICCD探测门宽和脉冲间隔分别为4 Hz, 0.2 μs和3.6 μs,主脉冲激光能量值和再加热脉冲激光能量值都设为25 mJ, 将ICCD探测延时从0 μs逐步增加到6.5 μs,间隔为0.5 μs。中药材样品中的4条特征谱线Fe I (358.17 nm)、Al I (396.15 nm)、 Ca II (393.37 nm)、CN (388.34 nm)的光谱强度和信背比(SBR)随探测延时的变化情况如
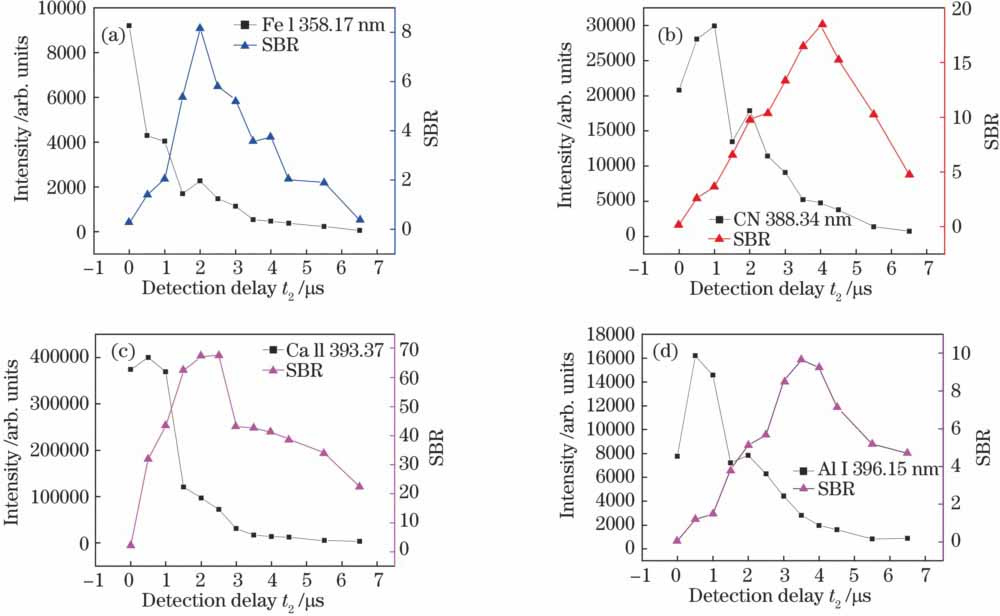
图 3. 4条特征谱线的光谱强度和信背比随探测延时的演化。(a) Fe I; (b) CN; (c) Ca II; (d) Al I
Fig. 3. Spectral intensities and SBRs of four characteristic spectral lines versus detection delay. (a) Fe I; (b) CN; (c) Ca II; (d) Al I
由
3.2 双脉冲的激光能量对光谱强度的影响
前后两束激光的能量值组合和脉冲间隔时间
由文献[
18,21-23]可知,第一束激光能量值会影响样品中等离子体的电子数和离子数密度,从而改变第二束激光作用于等离子体的环境,故对于不同的样品,获得最强光谱信号的双脉冲激光能量取值组合是不同的。此外,样品的表面粗糙度和热特性也会影响激光诱导样品等离子体的烧蚀过程。
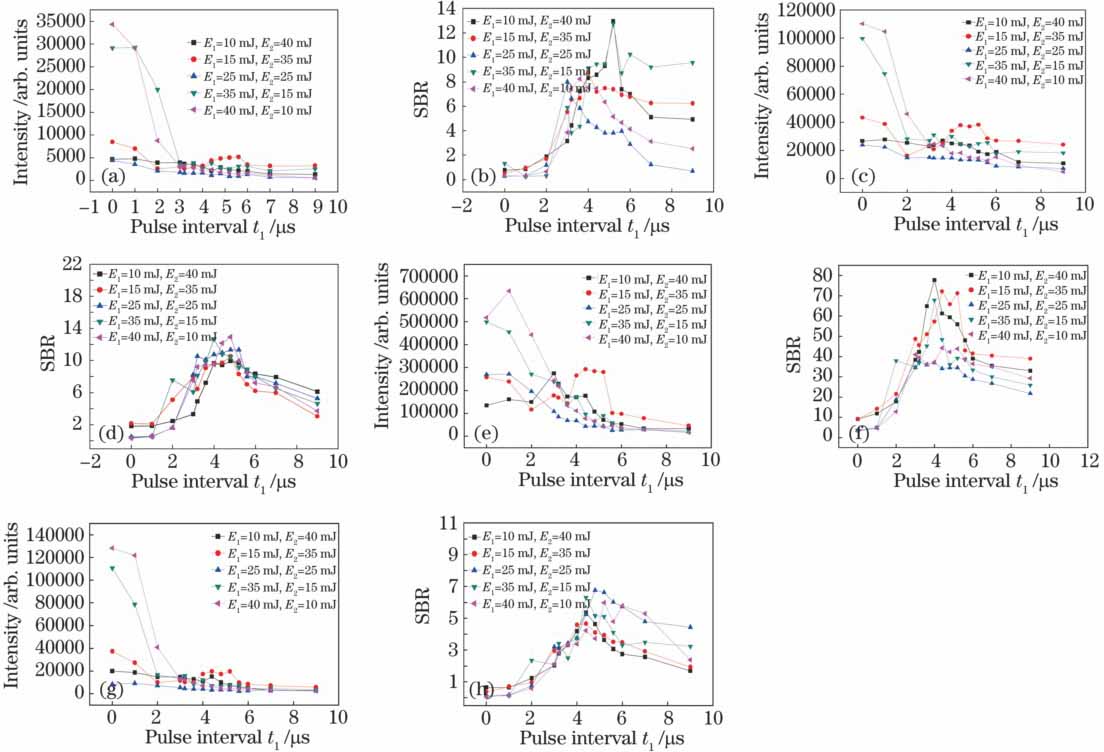
图 4. 不同激光能量组下4条特征谱线的光谱强度和SBR随脉冲间隔的变化。(a)(b) Fe I; (c)(d) CN; (e)(f) Ca II; (g)(h) Al I
Fig. 4. Spectral intensities and SBRs of four characteristic spectral lines versus pulse interval under different energy groups. (a)(b) Fe I; (c)(d) CN; (e)(f) Ca II; (g)(h) Al I
3.3 脉冲间隔对光谱强度的影响
脉冲间隔是影响双脉冲LIBS信号强度的主要因素[24]。设置主脉冲激光能量为15 mJ,再加热激光能量为35 mJ,探测延时为1.5 μs,4条特征谱线Fe I (358.17 nm)、Al I (396.15 nm)、Ca II (393.37 nm)、CN (388.34 nm)的光谱强度和信背比随脉冲间隔的变化规律基本一致,如
3.4 光谱增强程度
SP-LIBS的激光能量设为50 mJ,探测延时设为1.5 μs;RDP-LIBS的主脉冲激光能量设为15 mJ,再加热激光能量设为35 mJ,脉冲间隔和探测延时分别为4.4 μs和1.5 μs,获得的4条特征谱线Fe I (358.17 nm)、Al I (396.15 nm)、Ca II (393.37 nm)、CN (388.34 nm)的光谱对比图如
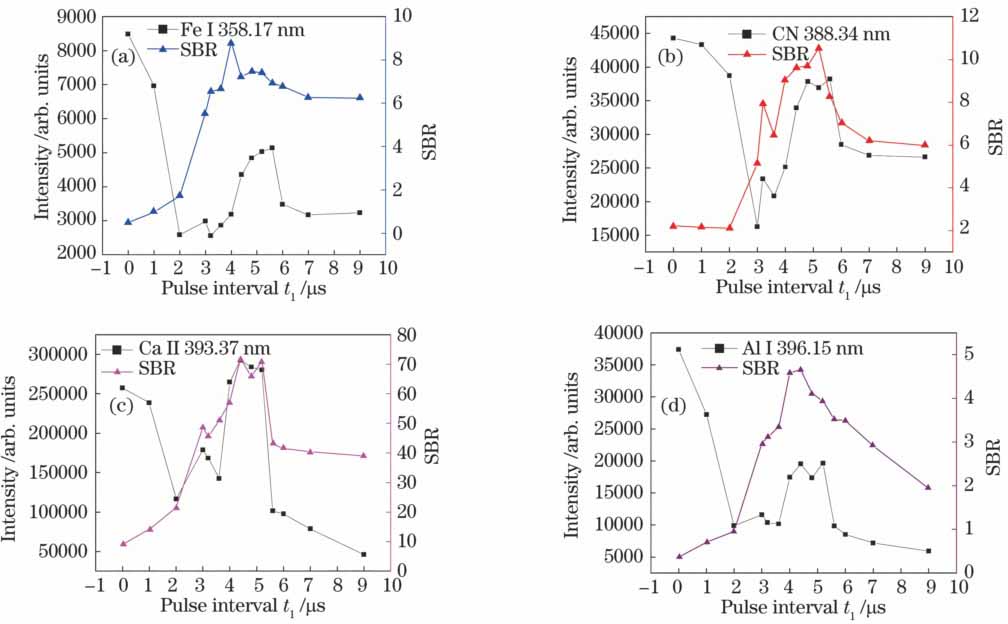
图 5. 4条特征谱线的光谱强度和信背比随脉冲间隔的变化规律(E1=15 mJ,E2=35 mJ,T1=1.5 μs)。(a) Fe I; (b) CN; (c) Ca II; (d) Al I
Fig. 5. Spectral intensities and SBRs of four characteristic spectral lines versus pulse interval (E1=15 mJ, E2=35 mJ, T1=1.5 μs). (a) Fe I; (b) CN; (c) Ca II; (d) Al I

图 6. SP-LIBS与RDP-LIBS的信号强度对比
Fig. 6. Signal intensity comparison for SP-LIBS and RDP-LIBS
9.3%,说明RDP-LIBS所采集的光谱信号的稳定性要高于SP-LIBS的。
3.5 等离子体的电子激发温度和电子数密度
研究了等离子体电子激发温度和电子数密度随探测延时的变化规律,并与单脉冲LIBS的结果进行对比。选择Ca II (317.93 nm)、Ca II (370.60 nm)、Ca II (373.69 nm)、Ca II (393.37 nm) 和Ca II (396.85 nm) 5条谱线,利用Boltzmann平面法计算等离子体电子激发温度,对Ca II (393.37 nm)进行Lorentz拟合,从而计算电子数密度。
表 1. 4条特征谱线的光谱增强倍数和相对标准偏差
Table 1. Enhancement factor of spectral intensity and RSD for four characteristic spectral lines
|
表 2. Boltzmann拟合所使用的Ca II谱线的光谱参数
Table 2. Spectral parameters of Ca II spectral line used for Boltzmann fitting
|
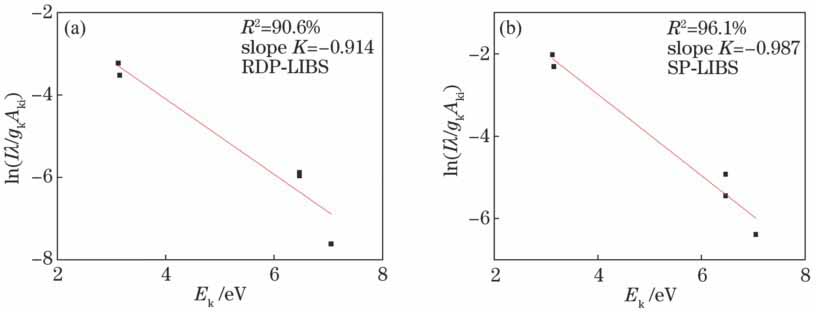
图 7. 黄连样品Ca II谱线的Boltzmann线性拟合。(a) RDP-LIBS;(b) SP-LIBS
Fig. 7. Boltzmann linear fitting of Ca II spectral line of Coptis Chinensis samples. (a) RDP-LIBS; (b) SP-LIBS
3.5.1 脉冲间隔对等离子体电子激发温度的影响
设置RDP-LIBS的主脉冲激光能量
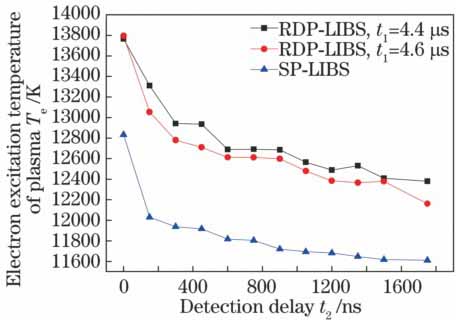
图 8. 等离子体电子激发温度随探测延时的变化曲线
Fig. 8. Electron excitation temperature of plasma versus detection delay
3.5.2 探测延时对等离子体电子数密度的影响
黄连样品中的等离子体电子数密度为
式中Δ
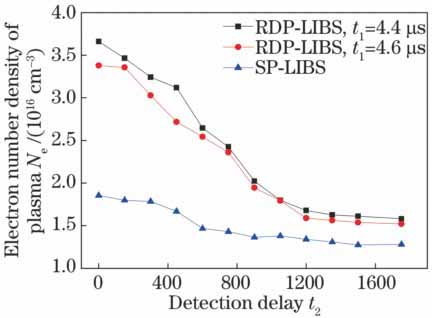
图 9. 等离子体电子数密度随探测延时的变化曲线
Fig. 9. Electron number density of plasma versus detection delay
3.5.3 局部热平衡验证
由于所采集的谱线均未发现明显的自吸收现象,因此在计算等离子体参数时可假设其满足局部热动平衡(LTE),一般通过McWhirter准则来验证:
式中Δ
4 结论
选用4条特征谱线Fe I (358.17 nm)、Al I (396.15 nm)、 Ca II (393.37 nm)、CN (388.34 nm)对RDP-LIBS实验参数进行优化,当探测延时为1.5 μs,主脉冲能量为15 mJ,再加热脉冲能量为35 mJ,脉冲间隔为4.4 μs时,RDP-LIBS获得最大的光谱信号增强。与SP-LIBS结果相比,4条特征谱线的增强倍数分别为4.0,5.5,10.0和3.5。同时研究了LIBS实验中等离子体的电子激发温度和电子数密度随探测延时的演化规律,取最优脉冲间隔4.4 μs时,RDP-LIBS的黄连样品等离子体电子激发温度和电子数密度平均值分别为12783 K与2.41×1016 cm-3,与SP-LIBS实验结果相比,等离子体电子激发温度提高了937 K,电子数密度增大了0.91×1016 cm-3,表明RDP-LIBS可以提高中药材的光谱特性。
[6] Wang J M, Liao X Y, Zheng P C, et al. Classification of Chinese herbal medicine by laser induced breakdown spectroscopy with principal component analysis and artificial neural network[J]. Analytical Letters, 2018, 51(4): 575-586.
Wang J M, Liao X Y, Zheng P C, et al. Classification of Chinese herbal medicine by laser induced breakdown spectroscopy with principal component analysis and artificial neural network[J]. Analytical Letters, 2018, 51(4): 575-586.
[7] 王彩虹, 黄林, 杨晖, 等. 蔬菜中Ca含量的LIBS快速检测研究[J]. 江西农业大学学报, 2016, 38(3): 483-487.
王彩虹, 黄林, 杨晖, 等. 蔬菜中Ca含量的LIBS快速检测研究[J]. 江西农业大学学报, 2016, 38(3): 483-487.
Wang C H, Huang L, Yang H, et al. Rapid detection of Ca in vegetables by LIBS[J]. Acta Agriculturae Universitatis Jiangxiensis, 2016, 38(3): 483-487.
[10] 杨宇翔, 康娟, 王亚蕊, 等. 水中铅元素的激光诱导击穿光谱-激光诱导荧光超灵敏检测[J]. 光学学报, 2017, 37(11): 1130001.
杨宇翔, 康娟, 王亚蕊, 等. 水中铅元素的激光诱导击穿光谱-激光诱导荧光超灵敏检测[J]. 光学学报, 2017, 37(11): 1130001.
[11] Babushok V I. De Lucia F C, Gottfried J L, et al. Double pulse laser ablation and plasma: Laser induced breakdown spectroscopy signal enhancement[J]. Spectrochimica Acta Part B: Atomic Spectroscopy, 2006, 61(9): 999-1014.
Babushok V I. De Lucia F C, Gottfried J L, et al. Double pulse laser ablation and plasma: Laser induced breakdown spectroscopy signal enhancement[J]. Spectrochimica Acta Part B: Atomic Spectroscopy, 2006, 61(9): 999-1014.
[20] 王琦, 董凤忠, 梁云仙, 等. 再加热双脉冲与单脉冲激光诱导Fe等离子体发射光谱实验对比研究[J]. 光学学报, 2011, 31(10): 1030002.
王琦, 董凤忠, 梁云仙, 等. 再加热双脉冲与单脉冲激光诱导Fe等离子体发射光谱实验对比研究[J]. 光学学报, 2011, 31(10): 1030002.
Wang Q, Dong F Z, Liang Y X, et al. Experimental comparison investigate on emission spectra of reheating double and single laser-induced Fe plasmas[J]. Acata Optical Sinica, 2011, 31(10): 1030002.
[25] Griem HR. Spectral line broadening by plasma[M]. New York: Academic Press, 1974: 342.
Griem HR. Spectral line broadening by plasma[M]. New York: Academic Press, 1974: 342.
Article Outline
王金梅, 郑慧娟, 郑培超, 谭癸宁. 正交再加热双脉冲激光诱导黄连等离子体的光谱特性[J]. 中国激光, 2018, 45(7): 0702006. Jinmei Wang, Huijuan Zheng, Peichao Zheng, Guining Tan. Spectral Characteristics of Coptis Chinensis Plasma Induced by Orthogonal Re-Heating Double-Pulse Laser[J]. Chinese Journal of Lasers, 2018, 45(7): 0702006.