钛及钛合金激光选区熔化技术的研究进展
下载: 2439次
1 引言
钛及钛合金具有密度低、比强度高、耐腐蚀性好、高温力学性能好、无毒无磁性、耐热性好、抗低温脆性好、可焊性好等优点,在航空航天、船舶、石油、化工、医疗等行业得到广泛应用[1-4]。近年来,钛及钛合金的发展非常迅速,其使用量大约以每年8%的速度增长,20世纪60年代,钛合金在军机中的使用量迅速增加到20%~25%。先进钛合金可大幅度减轻结构重量,提高安全性,已成为现代飞机和发动机不可缺少的结构材料之一。21世纪以来,世界航空工业对钛合金的需求迅猛增长[5-6]。然而,钛合金热导率低、硬度高、弹性变形大等缺点,使得传统锻造等加工方式已无法满足设计与整体化制造的要求[7-10]。
基于逐层累加成形思想的金属增材制造技术为钛合金复杂结构一体化的设计与制造提供了一种新思路。根据粉体材料输送方式的不同,金属增材制造技术主要分为以下两类。1)基于送粉和送丝的增材制造技术,以激光立体成形(LSF)、金属直接沉积(DMD)为代表,材料以粉末或丝状被直接送入高温熔池,熔化后逐层沉积。该技术只能成形出毛坯,后续需要依靠数控加工才能达到其净尺寸[11-12]。2)基于粉床的金属增材制造技术,如金属直接激光烧结(DMLS)、激光选区熔化(SLM)、电子束选区熔化(SEBM)等,粉体材料预先铺展在基板区域,其层厚一般为20~100 μm,利用高能束能量源根据预先规划的扫描路径逐层熔化金属粉末,直接净成形出零件,其表面仅需部分处理即可满足要求[13-15]。国内外针对金属增材制造技术开展了广泛研究并取得了诸多成果[16-20]。SLM具有激光光斑小、粉末粒径小、制造精度高,可实现薄壁及精密复杂结构的直接净成形,甚至无需后续处理,在中小型模具、生物结构材料等复杂构件的直接制造方面的应用备受关注[21]。2013年8月,NASA对SLM制造的J-2X发动机喷注器样件进行热试车,结果表明其完全满足设计使用要求[22]。德国BEGO牙科设备公司将SLM技术应用于牙冠和假牙的制造中;MCP公司应用SLM技术制造出精密金属模具插件及薄壁零件等,其尺寸精度及力学性能优良[23]。利用SLM技术制备具有随形冷却流道的刀具和模具,冷却效果更好,冷却时间更短,生产效率和产品质量均得到提高。采用SLM技术制备的具有交叉流道的微散热器,流道直径可达0.5 mm,表面粗糙度可达8.5 μm[24]。总体来看,SLM技术在复杂结构的制备方面具有广阔的应用前景。
本文从材料体系、成形工艺、显微组织及性能、应用等方面,系统概述了钛及钛合金SLM成形技术的进展,并总结了存在的问题及对今后的发展趋势进行了展望。
2 SLM技术原理及设备进展
SLM技术是一种金属增材制造技术,最早由德国Fraunhofer研究所于1995年提出[25],采用高能激光束逐层熔化堆积金属粉末直至成形,其基本原理如
SLM技术发展至今,设备发展较成熟,目前主要有EOS、Realizer、Concept Laser、SLM solutions、3D systems、Renishaw PLC、Phenix systems。国内由于三维打印市场发展缓慢,加上 SLM技术力量主要集中在西北工业大学、华南理工大学、华中科技大学、南京航空航天大学、中北大学、西安交通大学等高校,我国SLM技术的市场化还未取得突出成绩。近年来,西安铂力特公司、江苏永年激光成形技术有限公司、广东汉邦激光科技有限公司、西通公司等相继开发出SLM设备,但总体来看,国内SLM成形商用化设备市场几乎被国外企业垄断。因此,深入研究SLM成形技术,促进具有自主知识产权的商用SLM成形设备的产业化,对真正实现国内自主研发的SLM成形设备的大规模商业化应用具有重要意义。
3 钛及钛合金材料的SLM技术研究
目前,钛及钛合金SLM成形研究及应用主要集中在纯钛(CP-Ti)、Ti6Al4V及其他钛合金。国内外学者关于钛及钛合金SLM成形的研究主要集中在工艺参数及后处理等对构件致密度、组织及性能的影响方面。
3.1 CP-Ti的SLM成形及显微组织性能研究
致密度是表征构件内部缺陷的直接参量之一,对构件的性能影响显著。党新安等[26]采用单道、单层实验对CP-Ti粉SLM成形特性进行了初步研究,确立了较为优化的工艺参数范围,但样块最高致密度不超过80%。Abe等[27]通过改变工艺参数,获得了致密度大于92%的钛构件,验证了SLM成形高致密CP-Ti构件的可行性。Gu等[28]在合适的工艺参数下获得了致密度达99.5%的样件,认为不合理的工艺控制会引起微裂纹及孔隙,如

图 2. 不同扫描速度下的SLM成形钛构件横截面。 (a) 100 mm·s-1;(b) 200 mm·s-1;(c) 300 mm·s-1;(d) 400 mm·Fig. 2. Cross-sectional images of Ti components formed by SLM under different scanning speeds. (a) 100 mm·s-1; (b) 200 mm·s-1; (c) 300 mm·s-1; (d) 400 mm·
Fig. 2. Cross-sectional images of Ti components formed by SLM under different scanning speeds. (a) 100 mm·s-1; (b) 200 mm·s-1; (c) 300 mm·s-1; (d) 400 mm·
![不同衍射角下的 SLM钛构件的XRD谱。(a) 2θ=38.45°;(b) 2θ=40.18°[28]](/richHtml/lop/2018/55/1/011403/img_3.jpg)
图 3. 不同衍射角下的 SLM钛构件的XRD谱。(a) 2θ=38.45°;(b) 2θ=40.18°[28]
Fig. 3. XRD spectra of Ti components formed by SLM under different diffraction angles. (a) 2θ=38.45°; (b) 2θ=40.18°[28]
Li等[30]从激光功率角度对CP-Ti 的SLM成形样件的织构及力学性能进行了研究,发现其力学性能在高功率作用下呈现各向同性且呈现弱织构,而在低功率作用下呈现各向异性且具有强织构现象。Kang等[31]首次在CP-Ti 的SLM成形过程中施加静磁场(SMF),发现SMF能够细化和均匀化CP-Ti显微组织,消除织构,改善力学性能,如
SLM成形CP-Ti构件的力学性能见
总的来说,SLM成形技术可获得高性能的CP-Ti构件,但成形质量受到工艺参数、成形环境、粉末状态及设备的影响。目前,研究主要集中在激光功率、扫描速度等主要工艺参数对成形构件的影响方面,而对其他影响因素如成形环境、粉体材料、粉末层厚、扫描间距及扫描填充方式等的研究鲜有报道。另外,不同的SLM设备会引起成形构件力学性能的不同,这对工业化应用具有明显的影响方面。因此,需要进一步开展CP-Ti 的SLM成形研究,加速CP-Ti构件SLM成形标准的制定。
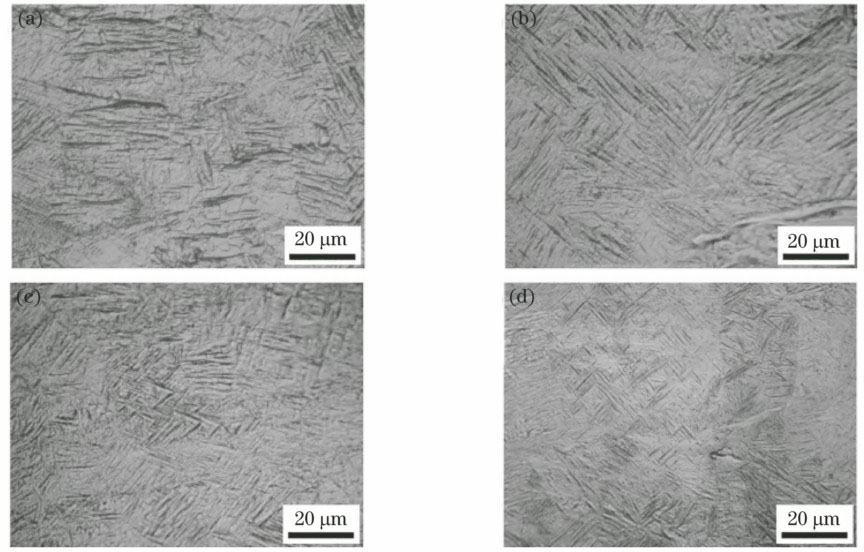
图 4. 不同扫描速度下的SLM成形钛构件显微结构。(a) 100 mm·s-1;(b) 200 mm·s-1;(c) 300 mm·s-1;(d) 400 mm·Fig. 4. Microstructures of Ti components formed by SLM under different scanning speeds. (a) 100 mm·s-1; (b) 200 mm·s-1; (c) 300 mm·s-1; (d) 400 mm·
Fig. 4. Microstructures of Ti components formed by SLM under different scanning speeds. (a) 100 mm·s-1; (b) 200 mm·s-1; (c) 300 mm·s-1; (d) 400 mm·

图 5. (a)(b)无SMF和(c)(d)有SMF下的CP-Ti的SLM样品显微结构
Fig. 5. Microstructures of CP-Ti samples formed by SLM (a)(b) without and (c)(d) with SMF
表 1. 不同SLM成形设备所得的CP-Ti的力学性能
Table 1. Mechanical properties of CP-Ti fabricated by different SLM equipments
|
![不同条件下的断口截面的(a)(b)光学显微图、(c)(d)扫描电镜图、(e)(f)取向图和(g)(h)反极图[31]](/richHtml/lop/2018/55/1/011403/img_6.jpg)
图 6. 不同条件下的断口截面的(a)(b)光学显微图、(c)(d)扫描电镜图、(e)(f)取向图和(g)(h)反极图[31]
Fig. 6. (a)(b) Optical microscopy images, (c)(d)scanning electron microscopy images, (e)(f) orientation maps and (g)(h) inverse pole figures of fracture section under different conditions[31]
3.2 Ti6Al4V的SLM技术研究
Ti6Al4V是一种α+β双相钛合金,具有良好的综合性能,被广泛应用于航空航天、汽车、飞机等领域。因此,SLM成形Ti6Al4V构件得到了各国学者的关注,相关研究较为全面和深入。
3.2.1 Ti6Al4V的SLM成形粉末研究
SLM成形技术通常采用粒径为几十微米的金属粉末,如
不同Ti6Al4V粉末材料特性对成形构件质量的影响。结果表明:无论是用流动性好的粉末还是用流动性差的粉末,制备出的构件的力学性能均满足要求,为确保SLM成形质量,还需考虑粉末生产流程中的其他因素,建立其他因素与粉末特性及制件质量之间的关系。金属粉末除了本身具有可塑性之外,还必须满足粒径细小、粒度分布窄、球形度高、流动性高以及松装密度高等要求[35]。目前,钛合金粉末生产成本普遍较高,故钛合金SLM成形技术的应用受到限制[36-37]。因此,需要进一步开发钛合金粉末生产工艺,提高生产效率和质量,实现低成本钛合金SLM成形,推动SLM技术的快速发展及广泛应用。

图 7. Ti6Al4V粉末形貌。(a) 500×;(b) 3000×
Fig. 7. Morphologies of Ti6Al4V powder. (a) 500×; (b) 3000×
3.2.2 Ti6Al4V的SLM成形致密化研究
构件的致密度直接影响其性能,这也是Ti6Al4V的SLM成形技术首先需要研究的问题。Gong等[38]开展了基于熔池形貌的单道工艺实验,根据不同孔隙类型将工艺参数进行分类,讨论了缺陷生成机制,认为高能量密度引起的熔池内部蒸发以及熔化过程中的传质现象造成大颗粒残留在每层上表面,导致出现缺陷[39-40]。因此,应该寻求合适的工艺参数,减少高能量密度下的飞溅现象[41-43],以改善成形构件的致密度,如
综上所述,在多种因素的共同作用下,Ti6Al4V SLM成形构件通常出现微孔、裂纹等缺陷,但通过调整工艺参数能够减少甚至消除孔隙等缺陷,实现Ti6Al4V的近致密甚至全致密成形。
![不同激光扫描速度下的熔化凝固过程[43]。(a) 5 mm·s-1;(b)10 mm·s-1; (c) 25 mm·s-1;(d) 50 mm·s-1;(e) 100 mm·s-1](/richHtml/lop/2018/55/1/011403/img_8.jpg)
图 8. 不同激光扫描速度下的熔化凝固过程[43]。(a) 5 mm·s-1;(b)10 mm·s-1; (c) 25 mm·s-1;(d) 50 mm·s-1;(e) 100 mm·s-1
Fig. 8. Melting and solidification processes under different laser scanning speeds[43]. (a) 5 mm·s-1; (b) 10 mm·s-1; (c) 25 mm·s-1; (d) 50 mm·s-1; (e) 100 mm·s-1
3.2.3 Ti6Al4V显微组织及性能研究
构件的性能直接取决于其显微组织,SLM成形是一种微小熔池的极度非平衡的多重热循环过程,其最终形成的显微组织与传统方法得到的不同[52]。显微组织与温度、冷却速率等密切相关,而SLM成形工艺条件直接影响温度及冷却速率。因此,各国学者研究了SLM成形条件对Ti6Al4V显微组织的影响。Thijs等[53]发现扫描策略的不同会引起显微组织形貌的不同,如
除了从工艺参数、成形过程设计等方面调整马氏体显微组织之外,后处理对显微组织特征及性能的影响也较为明显。
综上所述,Ti6Al4V 的SLM技术在工艺实验方面的研究较为深入,但SLM成形过程涉及复杂的物理化学过程,其显微组织形成演化机制仍需进一步阐释;同时,适用于特定Ti6Al4V SLM成形件的热处理工艺需要进一步探索和深入研究。需要深入研究和理解工艺、显微组织、性能三者间的关系,制定合理的Ti6Al4V SLM成形构件的标准规范。除此之外,Ti6Al4V SLM成形构件的其他性能如摩擦性能、抗疲劳性能及力学性能有待深入研究。同时,通过调整SLM成形工艺条件来实现显微组织的定制化有待于进一步研究。
![扫描策略对显微结构的影响[53]。(a)(b)(c)(d)单向扫描;(e)(f)(g)(h)交叉扫描](/richHtml/lop/2018/55/1/011403/img_9.jpg)
图 9. 扫描策略对显微结构的影响[53]。(a)(b)(c)(d)单向扫描;(e)(f)(g)(h)交叉扫描
Fig. 9. Influence of scanning strategy on microstructures[53]. (a)(b)(c)(d) Unidirectional scanning; (e)(f)(g)(h) cross scanning
![激光能量输入为(a) 0.5E0、(b) E0、(c) 2E0时,Ti6Al4V的SLM成形样件显微结构[54]](/richHtml/lop/2018/55/1/011403/img_10.jpg)
图 10. 激光能量输入为(a) 0.5E0、(b) E0、(c) 2E0时,Ti6Al4V的SLM成形样件显微结构[54]
Fig. 10. Microstructures of Ti6Al4V specimens formed by SLM with laser energy input of (a) 0.5E0, (b) E0 and (c) 2
![(a)支撑结构示意图;(b) As/Ap=0.25时的支撑结构图[63]](/richHtml/lop/2018/55/1/011403/img_11.jpg)
图 11. (a)支撑结构示意图;(b) As/Ap=0.25时的支撑结构图[63]
Fig. 11. (a) Schematic of support structure; (b) schematic of support structure with As/Ap=0.25[63]
![Ti6Al4V 的SLM成形构件的显微结构[63]。(a) As/Ap=0.125;(b) As/Ap=0.25;(c) As/Ap=0.4;(d) As/Ap=1](/richHtml/lop/2018/55/1/011403/img_12.jpg)
图 12. Ti6Al4V 的SLM成形构件的显微结构[63]。(a) As/Ap=0.125;(b) As/Ap=0.25;(c) As/Ap=0.4;(d) As/Ap=1
Fig. 12. Microstructures of Ti6Al4V components formed by SLM [63]. (a) As/Ap=0.125; (b) As/Ap=0.25; (c) As/Ap=0.4; (d) As/Ap=1
表 2. 不同加工条件下Ti6Al4V的SLM成形构件的力学性能
Table 2. Mechanical properties of Ti6Al4V components formed by SLM under different process conditions
|
3.2.4 Ti6Al4V的SLM成形件残余应力及变形研究
在SLM成形过程中,高能激光束逐点逐线作用于金属粉末,其温度梯度大、冷却速度快,导致成形件内部存在较大的残余应力,造成构件的翘曲变形,进而影响构件的尺寸精度和性能[72]。如何有效提高构件的尺寸精度和性能是SLM成形技术工业化应用的挑战之一。SLM成形过程中的残余应力研究受到了国内外学者的关注[62,73-77]。Yadroitsev等[78]研究发现,基板预热及扫描策略有利于减少Ti6Al4V的SLM成形构件残余应力。Parry等[79]通过热力耦合仿真分析了扫描策略对残余应力的影响。Mohanty等[80-81]研究了蜂窝扫描策略及分区扫描策略,证实了扫描策略对残余应力的控制是可行的。张升等[82]研究发现,工艺参数的调控可以削弱残余应力,达到减少或消除裂纹的目的。Mishurova等[83]通过改变扫描速度,研究了不同能量密度对Ti6Al4V的SLM成形构件残余应力的影响,结果表明,在构件上表面存在大的拉伸应变,较高的激光能量密度能够降低晶格应变,而热处理能够消除晶格应变。Vastola等[84]针对Ti6Al4V电子束选区熔化成形单道残余应力建立了仿真计算模型,结果表明,光斑尺寸、光源能量密度、扫描速度及粉床温度对残余应力的大小及分布均有影响,通过调节这些参数可以实现对残余应力的控制,且较高的粉床温度能够有效降低残余应力。
综上所述,残余应力在SLM成形构件中不可避免,如何控制甚至消除残余应力成为研究者们关注的问题。另外,如何从支撑设计角度控制成形过程中的应力变形及建立更为有效的仿真计算模型还有待进一步研究。
3.3 其他钛合金SLM技术研究
相较于研究较为成熟及应用较为广泛的Ti6Al4V SLM成形粉体材料,其他适用于SLM技术的钛合金及其复合材料的研究并不多见[85-86]。Marcu等[87-88]通过对Ti6Al7Nb粉末施加不同的热处理温度,研究了适用于SLM成形的Ti6Al7Nb粉末流动性,结果表明,经500 ℃处理后的Ti6Al7Nb粉末更加适用于SLM且成形构件的显微组织主要为柱状α'马氏体;同时,研究发现添加羟基磷灰石可以提高构件的硬度,且随着羟基磷灰石体积分数的增加,构件硬度不断增大。Chlebus等[89]开展了Ti6Al7Nb的SLM成形构件显微组织及力学性能的研究,结果与Ti6Al4V的类似,均具有高强度低韧性的特点,显微组织为均匀分布的分散相β-AlNbTi2强化的α'马氏体。Sercombe等[90]研究了热处理对Ti6Al7Nb 的SLM成形构件的影响。结果表明,固溶温度在1050 ℃时可获得均匀的显微组织,但该研究未进行力学性能测试。Zhang等[91-93]对生物医用合金TiNb24Zr4Sn8的SLM成形开展了研究,获得了近全致密试件,并探讨了不同因素对构件力学性能的影响,结果表明,随着扫描速度的降低,其显微硬度不断增大;当能量密度足够高时,激光斑点间的距离对致密度及显微硬度无明显影响,而当能量密度低时,其对构件性能是有害的。相比于传统的加工方法,SLM制备TiNb24Zr4Sn8表现出类似的力学特性且无明显的超弹性变形。进一步利用SLM技术制备TiNb24Zr4Sn8支架,其表现出优异的力学性能。
另外,TiAl合金由于具有密度低、弹性模量高、综合性能指标优于传统高温合金的特性,受到研究者们的广泛关注[94-95]。Li等[96-100]从工艺、显微组织及性能方面对TiAl45Cr2Nb5的SLM成形开展了较为深入的研究,并在此基础上对TiAl/TiB2复合材料的SLM技术进行了研究。
除了以上几种钛基材料,对其他钛基材料的SLM成形研究也已开展[102-109]。SLM成形钛基材料取得了较为理想的结果,但正如前面所述,钛基材料种类尚不多见,有待进一步深入揭示不同种类钛基材料SLM成形的机理。
4 钛合金SLM成形技术的应用研究
SLM成形技术可实现复杂结构件的一体化成形,较少甚至无需后续处理,大大提高了构件的制造效率,降低了加工成本。不少国内外学者应用SLM技术制备钛及钛合金复杂构件,取得了诸多成果,相关研究主要集中于医疗、工程等领域。
4.1 在医学领域上的应用
Sing等[110]利用SLM成形设备成功制备了CP-Ti和TiTa生物组织工程结构支架,如
Wang等[111]针对个性化骨板,应用SLM技术成功制备了该复杂结构,如
椎间融合器主要用于治疗背痛和蜕变性疾病,然而高刚度融合装置会出现应力集中现象,Pan等[112]利用SLM技术制备了Ti6Al4V多孔结构,如
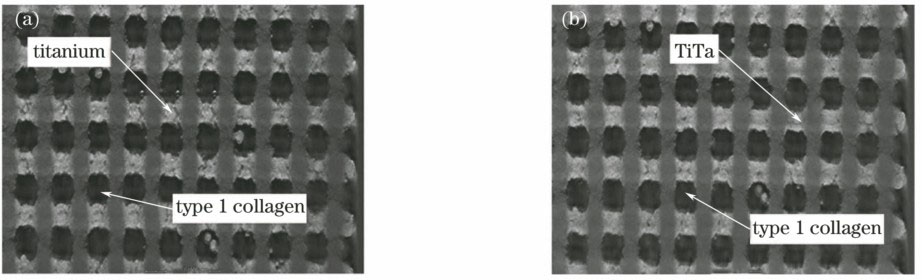
图 13. SLM制备的结构件。(a) CP-Ti;(b) TiTa
Fig. 13. Structural components fabricated by SLM. (a) CP-Ti; (b) TiTa
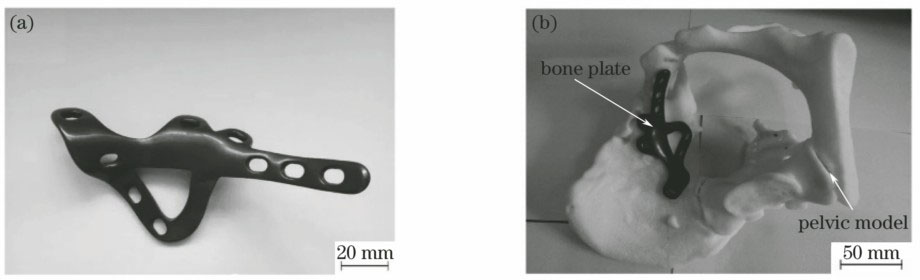
图 14. (a) 表面处理后的个性化骨板;(b) 骨板和骨盆模型的匹配
Fig. 14. (a) Individual bone plate after surface treatment; (b) matching between bone plate and pelvic model

图 15. 梯度融合器的(a)成形件和(b)模型
Fig. 15. (a) Formed part and (b) model of gradient fusion cage section
4.2 在工程领域上的应用
Cheng等[113]设计了两种吸声器结构,并利用SLM技术成功完成了该结构的制造,如
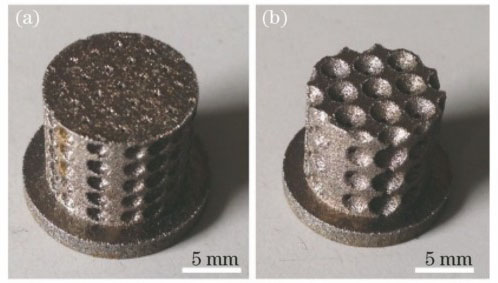
图 16. SLM成形制备的Ti6Al4V吸声器。(a)样品A;(b)样品B
Fig. 16. Ti6Al4V sound absorbers fabricated by SLM. (a) Sample A; (b) sample B
目前,钛合金SLM成形构件主要应用于医学领域,可修复骨骼、牙齿等结构。而在航空航天、汽车、船舶等领域的应用报道较少,主要原因可能是技术保密、成本高等[116]。
5 结束语
SLM技术经过二十多年的发展,在设备、工艺、性能及显微组织等方面取得了诸多成果,其商业化设备层出不穷,多种材料体系尤其是钛及钛合金的SLM成形技术日臻完善,但仍存在以下问题:1) SLM成形设备有待进一步完善,急需开发具有我国自主知识产权的商业化SLM成形设备,推动国产设备的大规模商业化使用;2) 适用于SLM成形的钛合金粉末体系有待健全,急需扩展专用钛合金材料体系,开发低成本钛合金粉末;3) 钛合金SLM成形工艺、显微组织及性能间的关系有待进一步研究,揭示其相互作用机制有助于实现钛合金SLM成形构件性能的稳定化以及标准规范的制定;4) 适用于钛合金SLM成形的热处理及其他后处理有待进一步完善;5) 对钛合金SLM成形构件应力变形的控制有待进一步研究;6) 有待开发大尺度钛合金SLM成形构件,以实现精密复杂大尺度钛合金构件的高精度一体化成形。
[1] LeyensC, PetersM. Titanium and titanium alloys:Fundamentals and applications[M]. New York: John Wiley & Sons, 2003: 3.
[2] 付艳艳, 宋月清, 惠松骁, 等. 航空用钛合金的研究与应用进展[J]. 稀有金属, 2006, 30(6): 850-856.
[3] 李俐群, 王建东, 吴潮潮, 等. Ti6Al4V激光熔化沉积熔池温度场与微观组织特性[J]. 中国激光, 2017, 44(3): 0302009.
[4] 王祥, 周建忠, 黄舒, 等. 激光喷丸对 TC4 钛合金抗氢脆性能的影响[J]. 光学学报, 2017, 37(9): 0914006.
[5] 赵永庆, 陈永楠, 张学敏, 等. 钛合金相变及热处理[M]. 长沙: 中南大学出版社, 2012.
Zhao YQ, Chen YN, Zhang XM, et al.Phase transformation and heat treatment of titanium alloys[M]. Changsha: Zhongnan University Press, 2012.
[6] 朱知寿. 我国航空用钛合金技术研究现状及发展[J]. 航空材料学报, 2014, 34(4): 44-50.
[7] 李富长, 宋祖铭, 杨典军. 钛合金加工工艺技术研究[J]. 新技术新工艺, 2010, 5: 66-69.
[8] 巩水利, 锁红波, 李怀学. 金属增材制造技术在航空领域的发展与应用[J]. 航空制造技术, 2013, 433(13): 66-71.
[9] 王华明. 高性能金属构件增材制造技术开启国防制造新篇章[J]. 国防制造技术, 2013, 3: 5-7.
[10] 邓贤辉, 杨治军. 钛合金增材制造技术研究现状及展望[J]. 材料开发与应用, 2014, 29(5): 113-120.
[11] 李静, 林鑫, 钱远宏, 等. 激光立体成形TC4钛合金组织和力学性能研究[J]. 中国激光, 2014, 41(11): 1103010.
[12] 李怀学, 巩水利, 孙帆, 等. 金属零件激光增材制造技术的发展及应用[J]. 航空制造技术, 2012, 416(20): 26-31.
[13] 潘爱琼, 张辉, 王泽敏. 选区激光熔化镍基单晶高温合金的熔池显微组织[J]. 激光与光电子学进展, 2017, 54(7): 041702.
[14] 侯慧鹏, 梁永朝, 何艳丽, 等. 选区激光熔化Hastelloy-X合金组织演变及拉伸性能[J]. 中国激光, 2017, 44(2): 0202007.
[15] 杨全占, 魏彦鹏, 高鹏, 等. 金属增材制造技术及其专用材料研究进展[J]. 材料导报, 2016( s1): 107- 111.
Yang QZ, Wei YP, GaoP, et al. Research progress of metal additive manufacturing technologies and related materials[J]. Materials Review, 2016( s1): 107- 111.
[18] 王霄, 王东生, 高雪松, 等. 轻合金构件激光增材制造研究现状及其发展[J]. 应用激光, 2016, 36(4): 478-483.
[19] 王华明, 张述泉, 汤海波, 等. 大型钛合金结构激光快速成形技术研究进展[J]. 航空精密制造技术, 2008, 44(6): 28-30.
[20] 冯颖芳. 西工大用3D打印制造3米长C919飞机钛合金部件[J]. 工艺技术研究, 2013, 1: 24.
[21] 董鹏, 李忠华, 严振宇, 等. 铝合金激光选区熔化成形技术研究现状[J]. 应用激光, 2015, 5: 607-611.
[22] 董鹏, 陈济轮. 国外选区激光熔化成形技术在航空航天领域应用现状[J]. 航天制造技术, 2014, 1: 1-5.
[23] 杨永强, 吴伟辉, 来克娴, 等. 金属零件选区激光熔化直接快速成形工艺及最新进展[J]. 航空制造技术, 2006, 2: 73-76.
[24] 尹华, 白培康, 刘斌, 等. 金属粉末选区激光熔化技术的研究现状及其发展趋势[J]. 热加工工艺, 2010, 39(1): 140-144.
[25] 顾冬冬, 沈以赴. 基于选区激光融化的金属材料零件快速成形现状与技术展望[J]. 航空制造技术, 2012, 8: 32-37.
[26] 党新安, 张晓博, 杨立军, 等. 钛粉激光选区熔化成型特性的研究[J]. 陕西科技大学学报, 2014, 1: 68-73.
Dang X A, Zhang X B, Yang L J, et al. Researching forming property of titanium powder in selective laser melting[J]. Journal of Shaanxi University Science & Technology, 2014, 1: 68-73.
[33] 梁永仁, 吴引江. 3D打印用钛及钛合金球形粉末制备技术[J]. 世界有色金属, 2016, 12: 150-151.
[35] 姚妮娜, 彭雄厚. 3D打印金属粉末的制备方法[J]. 四川有色金属, 2013, 4: 48-51.
[36] 赵霄昊, 左振博, 韩志宇, 等. 粉末钛合金3D打印技术研究进展[J]. 材料导报, 2016, 30(23): 120-126.
[37] 姚文静. Iluka追加投资研发低成本钛金属粉末[J]. 中国钛业, 2016, 2: 50.
[38] GongH, GuH, ZengK, et al. Melt pool characterization for selective laser melting of Ti-6Al-4V pre-alloyed powder[C]. Solid Freeform Fabrication Symposium, 2014: 256- 267.
[39] Gong HJ, RafiK, Gu HF, et al. Analysis of defect generation in Ti-6Al-4V parts made using powder bed fusion additive manufacturing processes[J]. Additive Manufacturing, 2014, 1/2/3/4: 87- 98.
[40] 孙健峰, 杨永强, 杨洲. 基于粉末特性的选区激光熔化Ti6Al4V表面粗糙度研究[J]. 中国激光, 2016, 43(7): 0702004.
[43] SatoY, TsukamotoM, YamashitaY, et al. Effect on beam profile of Ti alloy plate fabrication from powder by sputter-less selective laser melting[C]. SPIE, 2017, 10095: 100950Z.
[47] 叶梓恒. Ti6Al4V胫骨植入体个性化设计及其激光选区熔化制造工艺研究[D]. 广州: 华南理工大学, 2014.
Ye ZH. The personalized design and process research of selective laser melting manufacturing of Ti6Al4V tibial implant[D]. Guangzhou: South China University of Technology, 2014.
[48] 王小龙. 钛合金激光选区熔化工艺优化与性能研究[D]. 广州: 华南理工大学, 2016.
Wang XL. Study on process optimization and property of titanium alloy manufactured by selective laser melting[D]. Guangzhou: South China University of Technology, 2016.
[49] 王建宏, 白培康. Ti6Al4V粉末选区激光熔化单层扫描工艺研究[J]. 热加工工艺, 2013, 42(15): 13-15.
[50] 张升. 医用合金粉末激光选区熔化成形工艺与性能研究[D]. 武汉: 华中科技大学, 2014.
ZhangS. Research on the forming processes and properties in selective laser melting of medical alloy powders[D]. Wuhan: Huang Zhong University of Science and Technology, 2014.
[56] SimonelliM, Tse YY, TuckC. Further understanding of Ti-6Al-4V selective laser melting using texture analysis[C]// Proceedings of 23rd Annual International Solid Freeform Fabrication Symposium, 2012: 480- 491.
[68] 梁晓康, 董鹏, 陈济轮, 等. 激光选区熔化成形Ti-6Al-4V钛合金的显微组织及性能[J]. 应用激光, 2014, 34(2): 101-104.
[72] 张凯, 刘婷婷, 张长东, 等. 基于熔池数据分析的激光选区熔化成形件翘曲变形行为研究[J]. 中国激光, 2015, 42(9): 0903007.
[80] MohantyS, Hattel JH. Reducing residual stresses and deformations in selective laser melting through multilevel multiscale optimization of cellular scanning strategy[C]. SPIE, 2016, 9738: 97380Z.
[81] 邓诗诗, 杨永强, 李阳, 等. 分区扫描路径规划及其对SLM成型件残余应力分布的影响[J]. 中国激光, 2016, 43(12): 1202003.
[82] 张升, 桂睿智, 魏青松, 等. 选择性激光熔化成形TC4钛合金开裂行为及其机理研究[J]. 机械工程学报, 2013, 49(23): 21-27.
[91] BolzoniL, Ruiz-Navas E M, Gordo E. Influence of HIP parameters on the microstructure and mechanical properties of elemental titanium and Ti-6Al-7Nb alloy[C]. European Powder Metallurgy, 2012.
[95] 党兴. 我国钛铝合金领域专利技术发展综述[J]. 新材料产业, 2017, 3: 30-34.
[111] Sing S L, Wang S, Agarwala S, et al. Fabrication of titanium based biphasic scaffold using selective laser melting and collagen immersion[J]. International Journal of Bioprinting, 2017, 3(1): 1-7.
[113] Pan C T, Lin C H, Huang Y S, et al. Design of interbody fusion cages of Ti6Al4V with gradient porosity using a selective laser melting process for spinal fusion arthroplasty[J]. Journal of Laser Micro/Nanoengineering, 2017, 12(1): 34-44.
[116] 赵志国, 柏林, 李黎, 等. 激光选区熔化成形技术的发展现状及研究进展[J]. 航空制造技术, 2014, 463(19): 46-49.
[117] 丁红瑜, 孙中刚, 初铭强, 等. 选区激光熔化技术发展现状及在民用飞机上的应用[J]. 航空制造技术, 2015, 473(4): 102-104.
Article Outline
李俊峰, 魏正英, 卢秉恒. 钛及钛合金激光选区熔化技术的研究进展[J]. 激光与光电子学进展, 2018, 55(1): 011403. Li Junfeng, Wei Zhengying, Lu Bingheng. Research Progress on Technology of Selective Laser Melting of Titanium and Titanium Alloys[J]. Laser & Optoelectronics Progress, 2018, 55(1): 011403.