Near-field holography enhanced with antireflection coatings—an improved method for fabricating diffraction gratings
Download: 1251次
Soft x-ray diffraction gratings with constant or varied line spacing play key roles in the fields of synchrotron radiation[1,2] and plasma diagnosis[35" target="_self" style="display: inline;">–
Near-field holography (NFH) at either normal[15,16] or Littrow[17] incidence has a strong potential as a method to realize soft x-ray gratings. Compared to conventional holographic lithography, with its complex experimental setups[7], in this method only a fused silica phase mask as a beam splitter is necessary to generate grating patterns. Hence, the NFH setup is simple and resistant to environmental influences. An application of phase masks realized by electron beam lithography (EBL)[17] in NFH provides the appealing possibility of combining NFH’s robustness and efficiency with EBL’s immense flexibility regarding the spatial distributions of line densities. At present, NFH has been widely used in the fabrication of Bragg gratings at infrared wavelengths. Furthermore, most of the NFH investigations so far have focused on the optimization of masks at normal incidence[15,16]. To the best of our knowledge, little work has been done on the fabrication of soft x-ray gratings by NFH. A big problem with NFH is how to suppress the interface reflections from each optical element of the exposure setup. Such interface reflections may lead to a serious degradation of resist patterns after exposure and development. Hence, suppressing interface reflections is a promising approach to improving the quality of resist patterns. Recently, Amako
With the integration of appropriate optical antireflection coatings (ARCs) it is possible to suppress interface reflections. In this Letter, we demonstrate the feasibility of surface reflection reduced fused silica masks by using ARCs for applications in NFH. The corresponding method shall be called NFH with ARCs. We also compare the patterns of far field diffraction of resist gratings using NFH with either ARCs or refractive index liquid. Finally, soft x-ray gratings with a density of 2400 lines/mm are demonstrated using NFH with ARCs and ion beam etching.
For NFH at normal incidence, the 0th-order beam of a fused silica mask is difficult to suppress and can seriously degrade fringe visibility[15,16]. The disturbance from the undesired diffraction of the 0th order can be automatically avoided by oblique illumination. Hence, NFH at the Littrow incidence was investigated in this research. The general principle of NFH is introduced in the Littrow configuration, as schematically shown in Fig.
According to the geometry of NFH, there are two cases that we denote as Layouts I and II. In Layout I, the structured side of the mask faces the resist on the grating substrate [Fig.
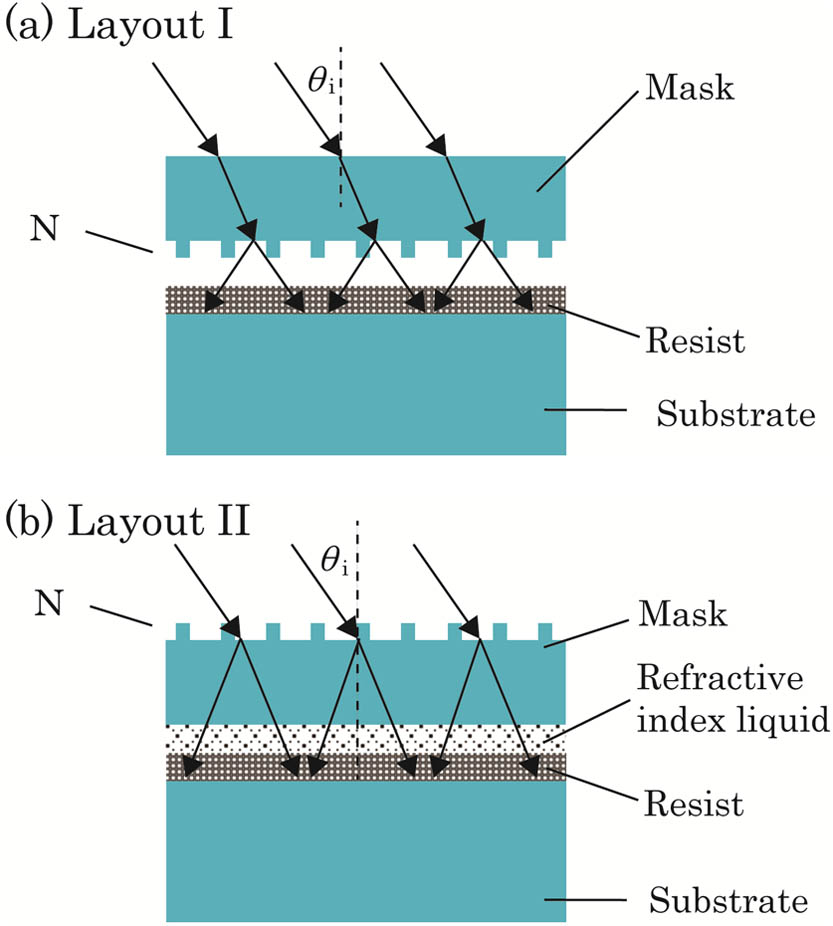
Fig. 1. Principles for fabricating a grating with a constant line spacing by NFH. (a) Layout I. (b) Layout II.
A key issue of NFH is the reduction of as much interface reflection in the setup as possible. The more parasitic reflections are suppressed, the higher the quality of the resist patterns.
Considering the structured side of the fused silica mask, besides the directly transmitted 0th and
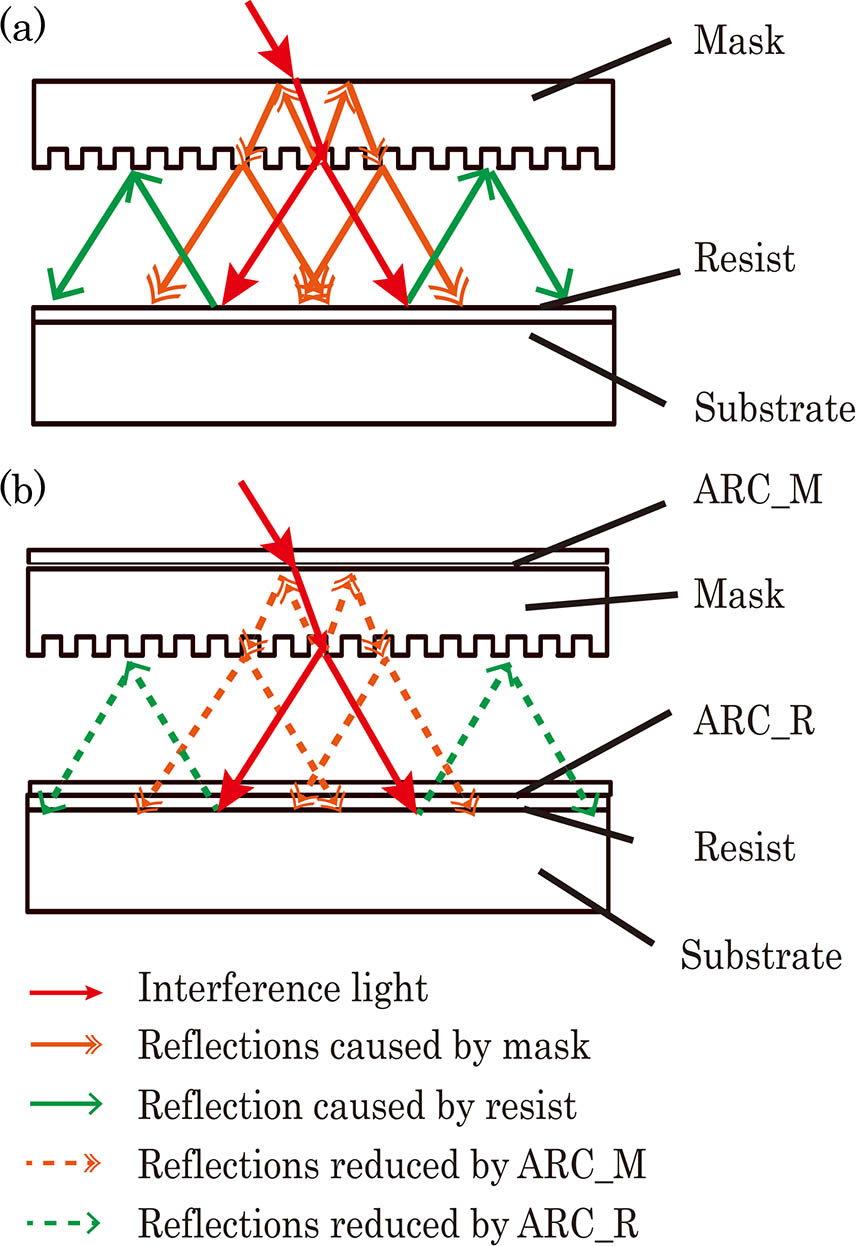
Fig. 2. Sketch of interface reflections and their suppressions with ARCs during NFH. (a) Setup without ARCs. (b) Setup with ARC_ M and ARC_R.
An additional source of interface reflections is the resist of the substrate to be patterned (green dashed lines with single arrowhead in Fig.
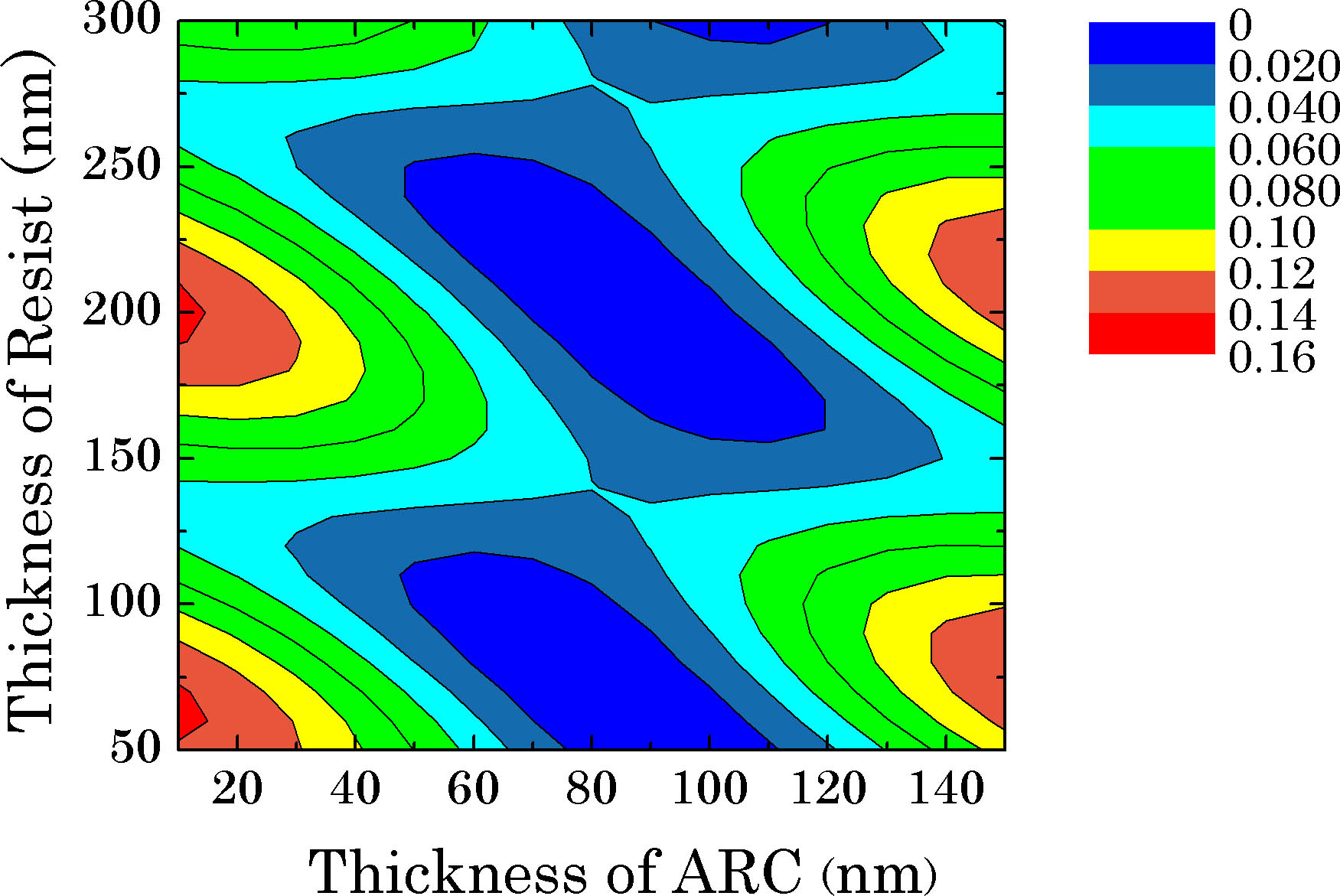
Fig. 3. Simulated reflectance of the substrate to be patterned as a function of the thickness of the resist and of the ARC_R at a wavelength of 441.6 nm and an incidence angle of 32°.
In order to experimentally verify the feasibility of our approach we realized an NFH setup that is illustrated in Fig.
To confirm the effect of ARCs on the quality of resist patterns, we compared the patterns formed with ARCs with those formed by a refractive index liquid. The fused silica masks investigated in this Letter were supplied by the Institute of Applied Physics, Friedrich Schiller University Jena, Germany. Both fused silica masks with nominally the same groove parameters were fabricated by EBL and inductively coupled plasma etching. To gain good contrast during NFH exposure, the profile parameter of the 2400 lines/mm mask was optimized to balance the diffractions at the 0th and
For Layout I, the ARC_M lowered the indirectly transmitted efficiency [orange lines with double arrowheads in Fig.
For Layout II, no additional ARCs was used. The structured side of the grating mask was placed in contact with air. The gap between the mask and the resist layer was filled with refractive index liquid (
Figure
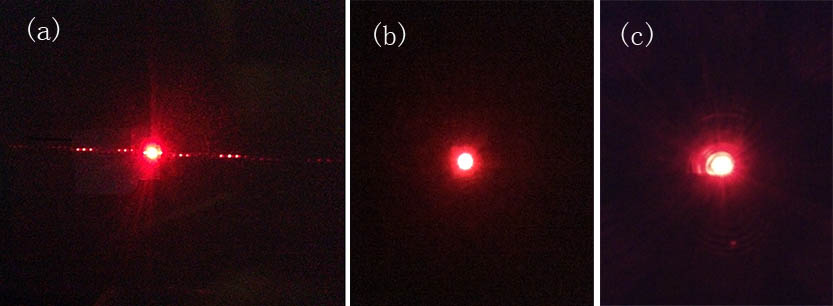
Fig. 5. Comparison of the far field diffraction spots of the resist grating after NFH. (a) Layout I without the suppression of interface reflections, (b) Layout I with ARCs, and (c) Layout II with the refractive index liquid.
The fabricated resist gratings, including the three instances shown in Fig.
Table 1. Measured Parameters of the Grating Samples
|
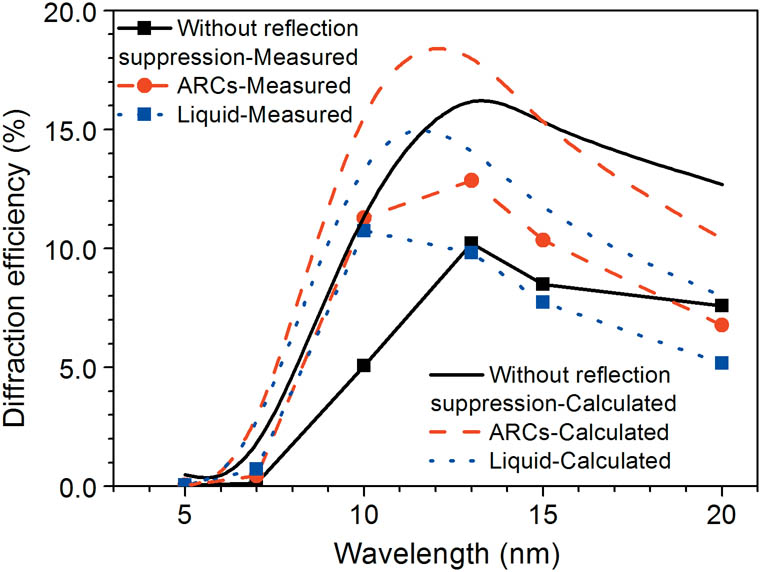
Fig. 6. Measured efficiency at the st order of the gold-coated laminar grating samples as a function of wavelength at an incidence angle of 76.78°. The fabrication differences of the samples are indicated by the legend.
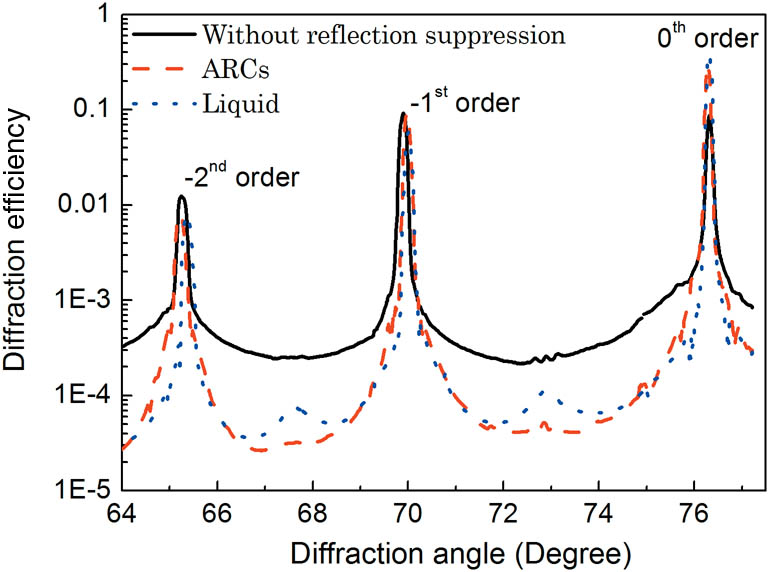
Fig. 7. Diffraction efficiency angular spectrum of the gold-coated laminar grating samples at an incidence angle of 76.78° and a wavelength of 13 nm. The fabrication differences of the samples are indicated by the legend.
These results underscore the necessity of reflection suppression during NFH. For gratings by NFH with ARCs or a refractive liquid, the origin of the small peaks between primary diffractions orders needs future investigation.
In conclusion, we demonstrate the applicability of ARCs in NFH setups for the realization of diffraction gratings with low scattered light. With the fabricated samples (line density 2400 lines/mm) we achieve a comparable optical quality of gratings fabricated with NFH enhanced with ARCs and a setup employing a refractive index liquid. However, in comparison to refractive index liquids, our approach with ARCs enables an easier control of the holographic process.
The presented results illustrate the potential of combining the strengths of NFH and EBL, i.e., high throughput, precise critical dimensions, and a large structure flexibility of reflection-reduced phase masks. The results form the basis for further investigations of the transfer relation of the spatial line density distribution between phase masks and resist gratings. This will open a new avenue for the efficient generation of high-quality resist patterns with even higher line densities and varied line spacings.
[2]
[4]
[5]
[6]
[7]
[8]
[9]
[10]
[11]
[12]
[13]
[14]
[15]
[16]
[17]
[18]
Yuanfang Li, Huoyao Chen, Stefanie Kroker, Thomas Käsebier, Zhengkun Liu, Keqiang Qiu, Ying Liu, Ernst-Bernhard Kley, Xiangdong Xu, Yilin Hong, Shaojun Fu. Near-field holography enhanced with antireflection coatings—an improved method for fabricating diffraction gratings[J]. Chinese Optics Letters, 2016, 14(9): 090501.