一种多波束可旋转的金属波导太赫兹阵列天线
1 引言
为了提高频谱资源的利用率,增加信道容量,能够同时产生多个独立波束的多波束天线被越来越多地应用在各个领域[1-4],如多目标天线雷达[5-7]、卫星通信[8-10]以及无人驾驶设备[11]等。太赫兹(THz)波具有丰富的频谱资源,可以大幅度提高通信带宽,实现超高速无线通信,可以满足通讯信息量迅速上涨的需求,相比于传统天线,THz天线具有频率更高、带宽更宽、波束更窄的特点,这些特点赋予了THz天线巨大的应用潜力[12]。涡旋波束因为携带了轨道角动量而在天线通信系统信道容量、提升信号传输速率方面有广泛的应用前景[13-16],利用涡旋波束的干涉特性实现多波束旋转为完成对目标方位角的测量提供了新的思路。
利用亚波长尺度单元阵列构成的调控器件如超表面[17-18]等,可实现对THz波的灵活操纵和调控。本文所用的金属矩形波导是波导系统中一种常见的波导结构,其波导壁由金属材料制成,具有规则的矩形截面、腔体内可填充电介质(一般是空气)的特征[19]。它能像传输相位型超表面一样通过电磁波在传输过程中产生的光程差来实现相位调控,根据矩形波导理论,每个矩形孔支持两个正交偏振态的基模(TE01模和TE10模),因此与电介质超表面相比,这种金属波导单元对电磁波的控制主要依赖于垂直于偏振方向孔的尺寸,单个矩形孔可以实现两个正交偏振波的独立控制[20]。更重要的是,相位延迟与空穴尺寸之间的解析关系可以清晰地表达出来,更方便我们控制单元的选择。此外,由于异常透射效应[21]的存在,该亚波长金属矩形孔有着异常高的透射率,便于器件的设计与实际应用。
在本文中,我们提出了一种基于金属波导阵列的多波束可旋转THz天线。通过设计单元的结构尺寸,可以同时且独立调控其所传导的两种正交模式在0~2π范围内的相位延迟。进而通过设置一系列金属波导单元的排布,可将入射波两正交偏振分量转化为阶数相反的涡旋波束。由于这两束波的偏振方向相互正交,因而无法直接干涉,但其在其它偏振方向如45°方位角上的投影分量可以发生干涉。而干涉增强(或减弱)的方位角与入射波两正交分量的相位差成正比,因此可通过改变该相位差,使得干涉波束发生旋转,从而实现利用两正交分量的相位差对旋转波束方位角的调控。所设计拓扑阶数为m = ± 1的涡旋阵列天线可产生双波束远场辐射图样,其远场增益为31 dBi,其3 dB带宽高达61 GHz (114~175 GHz,相对带宽42.2%)。通过采用更高拓扑阶数的涡旋波束进行干涉,还可以产生更多的波束。所提出的多波束旋转可控的THz阵列天线可为雷达天线的方位角测量提供新的思路,对丰富THz频段的阵列天线设计具有重要意义。
2 原理与理论模拟
2.1 设计原理
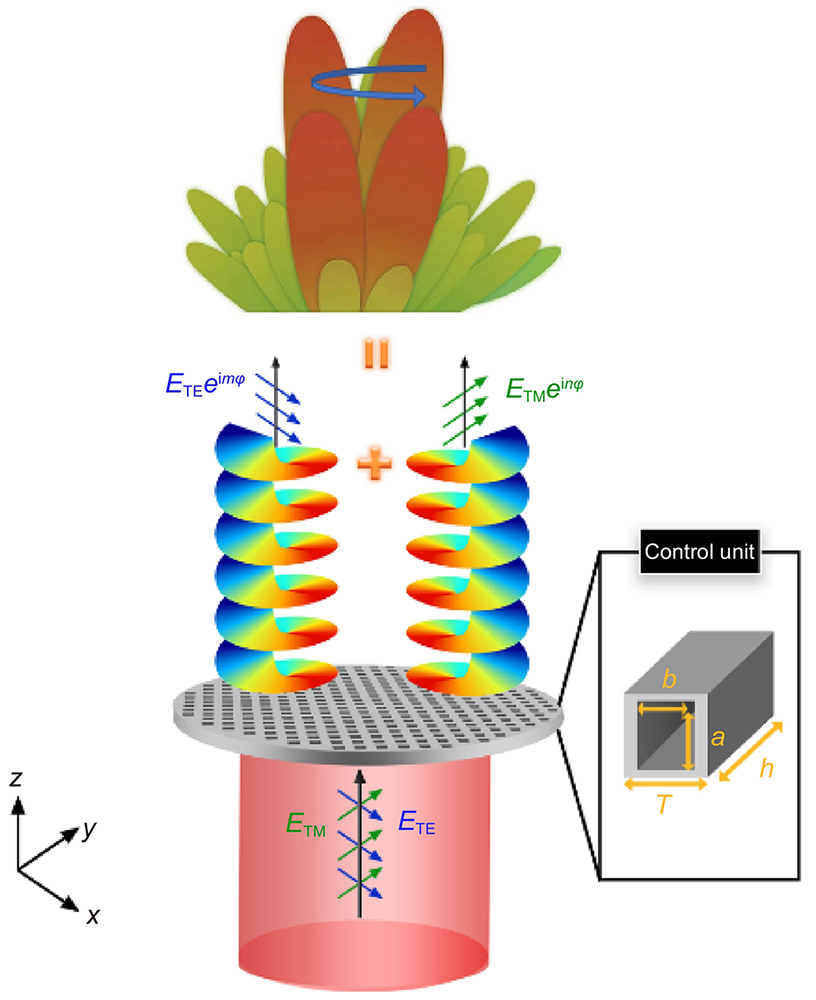
图 1. 基于金属波导的多波束可旋转THz阵列天线示意图
Fig. 1. Schematic diagram of a multibeam rotatable THz array antenna based on metallic waveguides
其中:θd为干涉增强的方位角,m为涡旋波束的拓扑荷数,当m = 1且n = 1时,式(1)可以写为:
由式(2)可知,干涉增强的方位角θd与入射波两正交偏振分量的相位差Δφ存在线性关系,这意味着可通过改变相位差Δφ实现45°偏振方向上干涉图样的方位角θd的变化,即干涉图样的旋转。
涡旋波束是一种具有螺旋相位波前的特殊光场,相位分布中含有exp(imθ) 项,θ为螺旋形相位的方位角,在传输过程中每绕光轴转一周,波前的相位就改变2mπ,其携带的相位可以表示为
2.2 调控单元的选择
所采用的工作频率为0.14 THz,调控单元中所用金属为Al,其相对介电常数可通过Drude模型计算得到[23]。铝孔中的电介质是空气,其折射率n0 = 1,所设计调控单元的周期T = 2 mm,厚度h = 6 mm。在工作频率下,但金属为完美电导体时,x偏振的THz的传播常数可以写为[20]
同理,y偏振的THz的传播常数可以写为
其中k0 = 2π/λ为波数,n0为金属波导单元中介质的折射率,a为金属波导单元在x方向上的尺寸,b为在y方向上的尺寸。当THz波通过厚度为h的金属波导单元后,累积的相位延迟分别为Φx = kxh和Φy = kyh,因此可以知道金属波导单元对电磁波的调控主要依赖于垂直于偏振方向上孔的尺寸,其可实现对THz波两个正交偏振分量的独立控制。式(4)与式(5)明确了相位延迟与尺寸(a,b)的解析关系。通过商用软件COMSOL模拟,也同时可以计算得出相位延迟与结构参数的关系,与解析关系较为吻合。我们选取了64个金属波导调控单元,用于完整覆盖x偏振分量与y偏振分量0~2π的相位延迟,且相位变化间隔均为π/4。由于异常透射效应的存在,这64个调控单元中的绝大多数透射率都大于90%,这为实现高增益提供了有利的前提条件,其具体尺寸如
表 1. 64个金属矩形孔的尺寸(a, b)
Table 1. Dimensions (a, b) of 64 selected sub-wavelength metallic holes
|
3 天线仿真结果
通过式(3)可以获得涡旋阵列天线所需实现的相位延迟,再根据此相位延迟对金属波导单元进行排布,从而得到偏振复用高增益涡旋透射阵列天线。当入射波沿方位角为45°的方向偏振时,该阵列天线可将入射波的两个正交偏振分量分别转化为 ±1阶高增益涡旋波束,这两束波的振幅相等,涡旋相位变化相等。虽然这两束正交偏振波无法直接干涉,但二者在45°方位角方向上的投影分量可以相互干涉。当入射波两正交分量的相位差分别为0、90°、180°和270°时,由商业软件COMSOL模拟所得的三维远场辐射图样如

图 2. 入射波两正交偏振分量的相位差为(a) 0°,(b) 90°,(c) 180°,和(d) 270°时的三维辐射图样
Fig. 2. Three-dimensional radiation patterns under the phase difference of two orthogonal polarization components being (a) 0°, (b) 90°, (c) 180°, and (d) 270°, respectively

图 3. 干涉增强方位角θd与两偏振分量的相位差Δφ的关系
Fig. 3. The dependence of the interference-enhanced azimuth angle θd on the different phase differences Δφ between x- and y-polarized components
当阵列天线直径为52 mm (≈24.2λ),两正交偏振分量的相位差为0时,xz平面的二维远场方向图如
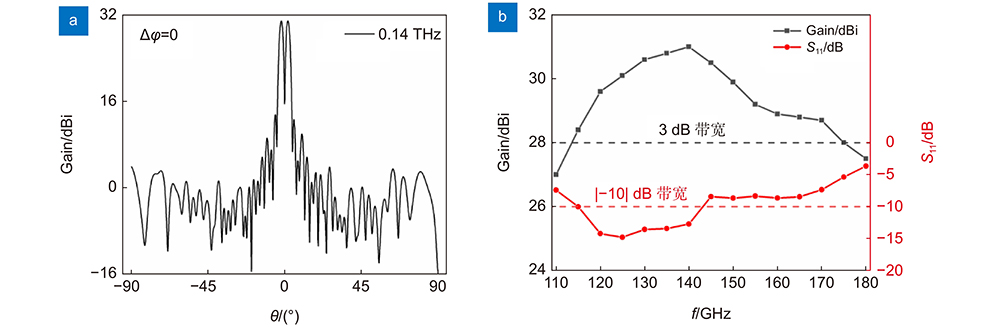
图 4. (a)当两偏振分量相位差为0时在xz平面的二维远场方向图;(b)增益和反射系数S11随频率的变化曲线
Fig. 4. (a) Two-dimensional far-field radiating pattern in the xz plane for Δφ = 0; (b) Dependences of the gain and reflection coefficient S11 frequency
4 结论
我们提出了一种基于金属波导的多波束可旋转THz阵列天线。通过设置一系列金属波导单元的排布,可将入射波两正交偏振分量转化为阶数相反的涡旋波束。这两束波在其它偏振方向如45°方位角上的投影分量可以发生干涉,而干涉增强(或减弱)的方位角与入射波两正交分量的相位差成正比,因此可以利用两正交分量的相位差对旋转波束方位角的调控。该阵列天线的远场增益为31.0 dBi,其3 dB带宽高达61 GHz (114 ~ 175 GHz,相对带宽为42.2%),插入损耗仅为1.4 dB。所提出的多波束旋转可控的THz阵列天线可丰富THz频段的阵列天线设计,能够为雷达天线的方位角测量提供新的思路。
Overview: With the high-speed development of mobile communication and the increasingly complex communication environment, multibeam terahertz (THz) antennas with the characteristics of high frequency, wide bandwidth and narrow beam have great application potential in 6G wireless communication. The vortex beam carrying orbital angular momentum has a broad application prospect in enhancing the channel capacity of the communication system and improving the signal transmission rate. Using the interference of vortex beams to realize multibeam rotation can provide a new way for accomplishing the azimuth measurement of the target.
A metallic waveguide can be used as the control unit for full control of the phase, polarization, and amplitude of THz wave. In contrast to the plasmonic and dielectric metasurfaces, the phase delay of the waveguide unit is dependent and independent on the hole dimensions perpendicular and parallel to the polarization direction, respectively. Furthermore, the amplitude and polarization can be completely controlled by tuning the dimension and the orientation angle of metal holes. Notably, the analytical relationship between the phase delay and hole dimensions can be presented explicitly, which greatly simplifies the design process to select the waveguide array for a desired phase distribution. Due to the extraordinary transmission effect, the sub-wavelength metal rectangular hole can attain a very ultra-high transmittance, which is convenient for the device design and practical application. This control unit not only demonstrates a facile scheme to manipulate EM waves but also draws a promising approach to realize multifunctional devices with simplified design and high durability.
In this paper, a multibeam rotatable THz array antenna based on metallic waveguides is proposed. Thanks to the excellent control properties of the metallic waveguides, the phase delay of the two orthogonal polarization modes can be tuned in the range of 0 ~ 2π by designing the cell structure size. By manipulating the phase distribution, the two orthogonal polarization components can be transformed into vortex beams with the same intensity distributions and opposite orders. The polarization directions of the two components are orthogonal to each other, and thus they cannot interfere directly. However, their 45° polarized components can interfere, and the interferometric pattern can be rotated by changing the phase difference between the two components. Moreover, the array antenna also shows the performance of high gain (31.0 dBi) and wide bandwidth (up to 61 GHz). The proposed multi-beam rotatable THz array antenna can provide a new way for the azimuth measurement of radar antennas and is of great significance in enriching the design of array antennas in the THz band.
[1] Chen S Z, Zhao J. The requirements, challenges, and technologies for 5G of terrestrial mobile telecommunication[J]. IEEE Commun Mag, 2014, 52(5): 36-43.
[2] Chowdhury M Z, Shahjalal M, Ahmed S, et al. 6G wireless communication systems: applications, requirements, technologies, challenges, and research directions[J]. IEEE Open J. Commun. Soc., 2020, 1: 957-975.
[3] Dahlman E, Mildh G, Parkvall S, et al. 5G wireless access: requirements and realization[J]. IEEE Commun Mag, 2014, 52(12): 42-47.
[4] Hong W, Jiang Z H, Yu C, et al. Multibeam antenna technologies for 5G wireless communications[J]. IEEE Trans Antennas Propag, 2017, 65(12): 6231-6249.
[5] 程院兵, 顾红, 苏卫民. 一种新的双基地MIMO雷达快速多目标定位算法[J]. 电子与信息学报, 2012, 34(2): 312-317.
Cheng Y B, Gu H, Su W M. A new method for fast multi-target localization in bistatic MIMO radar[J]. J Electron Inf Technol, 2012, 34(2): 312-317.
[6] 柳超, 王月基. 对海探测雷达多目标跟踪技术综述[J]. 雷达学报, 2021, 10(1): 100-115.
Liu C, Wang Y J. Review of multi-target tracking technology for marine radar[J]. J. Radars, 2021, 10(1): 100-115.
[7] Yi W, Zhou T, Ai Y, et al. Suboptimal low complexity joint multi-target detection and localization for non-coherent MIMO radar with widely separated antennas[J]. IEEE Trans Signal Process, 2020, 68: 901-916.
[8] 周乐柱, 李斗, 郭文嘉. 卫星通信多波束天线综述[J]. 电子学报, 2001, 29(6): 824-828.
Zhou L Z, Li D, Guo W J. Review of multiple-beam antennas for satellite communications[J]. Acta Electron Sin, 2001, 29(6): 824-828.
[9] Yu L, Wan J X, Zhang K, et al. Spaceborne multibeam phased array antennas for satellite communications[J]. IEEE Aerosp Electron Syst Mag, 2023, 38(3): 28-47.
[10] Martinez-De-Rioja E, Encinar J A, Florencio R, et al. 3-D bifocal design method for dual-reflectarray configurations with application to multibeam satellite antennas in Ka-band[J]. IEEE Trans Antennas Propag, 2019, 67(1): 450-460.
[11] Luo Q, Gao S, Li W T, et al. Multibeam dual-circularly polarized reflectarray for connected and autonomous vehicles[J]. IEEE Trans Veh Technol, 2019, 68(4): 3574-3585.
[12] 王少宏, 许景周, 汪力, 等. THz技术的应用及展望[J]. 物理, 2001, 30(10): 612-615.
Wang S H, Xu J Z, Wang L, et al. Applications and prospects of terahertz technology[J]. Physics, 2001, 30(10): 612-615.
[13] 陆璇辉, 黄慧琴, 赵承良, 等. 涡旋光束和光学涡旋[J]. 激光与光电子学进展, 2008, 45(1): 50-56.
Lu X H, Huang H Q, Zhao C L, et al. Optical vortex beams and optical vortices[J]. Laser Optoelectron Progress, 2008, 45(1): 50-56.
[14] Xu J C, Guo Y X, Yang P Y, et al. Recent progress on RF orbital angular momentum antennas[J]. J Electromagn Waves Appl, 2020, 34(3): 275-300.
[15] Wang H, Li Y F, Han Y J, et al. Vortex beam generated by circular-polarized metasurface reflector antenna[J]. J Phys D:Appl Phys, 2019, 52(25): 255306.
[16] 郭忠义, 龚超凡, 刘洪郡, 等. OAM光通信技术研究进展[J]. 光电工程, 2020, 47(3): 190593.
Guo Z Y, Gong C F, Liu H J, et al. Research advances of orbital angular momentum based optical communication technology[J]. Opto-Electron Eng, 2020, 47(3): 190593.
[17] Cao T, Lian M, Chen X Y, et al. Multi-cycle reconfigurable THz extraordinary optical transmission using chalcogenide metamaterials[J]. Opto-Electron Sci, 2022, 1(1): 210010.
[18] Fan J X, Li Z L, Xue Z Q, et al. Hybrid bound states in the continuum in terahertz metasurfaces[J]. Opto-Electron Sci, 2023, 2(4): 230006.
[19] 刘学观, 郭辉萍. 微波技术与天线[M]. 2版. 西安: 西安电子科技大学出版社, 2001.
LiuX G, GuoH P. Microwave Technology and Antennas[M]. 2nd ed. Xi’an: Xidian University Press, 2001.
[20] Liang H W, Li J Q, Wu Z Y, et al. Metallic waveguide arrays for metasurface-like control with high simplicity in design[J]. Adv Opt Mater, 2020, 8(18): 2000605.
[21] Ebbesen T W, Lezec H J, Ghaemi H F, et al. Extraordinary optical transmission through sub-wavelength hole arrays[J]. Nature, 1998, 391(6668): 667-669.
[22] Jiang Z, Lu J H, Fan J Y, et al. Polarization-multiplexing bessel vortex beams for polarization detection of continuous terahertz waves[J]. Laser Photon Rev, 2023, 17(3): 2200484.
[23] Ordal M A, Bell R J, Alexander R W, et al. Optical properties of fourteen metals in the infrared and far infrared: Al, Co, Cu, Au, Fe, Pb, Mo, Ni, Pd, Pt, Ag, Ti, V, and W[J]. Appl Opt, 1985, 24(24): 4493-4499.
蒋钊, 黄峻堃, 张敏, 苏红, 李玲, 梁华伟. 一种多波束可旋转的金属波导太赫兹阵列天线[J]. 光电工程, 2023, 50(9): 230140. Zhao Jiang, Junkun Huang, Min Zhang, Hong Su, Ling Li, Huawei Liang. A multibeam rotatable metallic waveguide terahertz array antenna[J]. Opto-Electronic Engineering, 2023, 50(9): 230140.