无频响影响的光弹调制器定标新方法【增强内容出版】
With the wide application of ellipsometry spectroscopy technology as the core device for ellipsometry spectrometers in recent years, an increasing number of researchers have begun to study photoelastic modulators (PEM). The amplitude of the phase delay generated when the PEM performs phase modulation is influenced by the control voltage, temperature, and other factors; therefore, the PEM must perform calibration before carrying out the work. Current calibration methods for the PEM mainly include the Bessel function ratio, half-wave flat-top, and fundamental frequency component maximum methods. Many calibration methods require the use of the Bessel function ratio, and the frequency response of the calibration system must be considered based on the ratio between different frequency octaves, which is also a problem likely to be ignored. Moreover, this problem is difficult to solve. To solve this problem, we propose a new calibration method that can eliminate the influence of the frequency response of the calibration system. The proposed method exhibits excellent system robustness.
The proposed system consisted of a calibration optical route polarizer, PEM, waveplate, and analyzer. This method has no special requirements for the azimuths of the polarizer, analyzer, waveplate, or the phase delay of the waveplate in the system. In this method, the zero-point of the second-harmonic component, Am=5.136 rad, was used as the calibration auxiliary point, and the ratio of the first-order Bessel function between the undetermined and calibration auxiliary points was used as the calibration function. By determining the ratio of the first harmonic component between the fixed and calibration auxiliary points, the real-phase delay amplitude of the undetermined point was derived via calculations.
The proposed method uses the ratio of the Bessel function of the same order between different values instead of the ratio between different frequencies, which solves the frequency-response problem of the calibration system. The calibration results show that the calibration curves obtained using the fourth- and second-order Bessel function ratio methods significantly differ from that without a frequency response (Fig.3). This is caused by the evident frequency-response difference between the second and fourth octaves of the detection system consisting of the detector and lock-in amplifier. The fitting curve of the proposed method is expressed by Am=1.203vm+0.098. The half-wave flat-top method is used to verify the amplitude of the π rad phase delay in the calibration curve. When the control voltage is 2.53 V, a flat-top waveform is generated (Fig.5), which is consistent with that of the simulation (Fig.4), indicating that the proposed method is feasible. In addition, we calculated the calibration deviation of the light intensity fluctuation for the method, and the deviation of most of the calibration points, Am, was less than 1%. The calibration near the extreme point of the Bessel function is significantly influenced by the light intensity fluctuation (Fig.6) owing to the insignificant change in the value of the Bessel function near the extreme point. A laser with a smaller light-intensity fluctuation or a more stable laser can be used to control the power supply to minimize the Am calibration deviation caused by light-intensity fluctuations.
In this study, a new calibration method for a PEM is proposed. In the proposed method, the zero-value point of the second-order Bessel function is used as the auxiliary calibration point. The ratio of the amplitude of the fundamental frequency component between the punctuation point to be determined and the auxiliary calibration point corresponds to the ratio of the first-order Bessel function used for calibrating the entire working range of the PEM. The experimental results show that the calibration relationship between Vm and Am is linear, except near the extreme points of the fundamental frequency components (1.841 and 5.331 rad), and the calibration results are verified using the half-wave flat-top method. The calibration deviation caused by the fluctuation in light intensity is lower than 1%, except near the extreme points of the Bessel function. The calibration method is not affected by the frequency response of the detection system and has no special numerical and accurate requirements for the azimuth angle and phase delay of the waveplate, polarizer, and polarizer. It is simple to operate and robust, and it achieves PEM calibration in the entire Vm working range.
1 引言
光弹调制器(PEM)是偏振调制技术中的核心关键器件,在晶体生长、物理化学、生物物理等领域均有广泛的应用。其基本原理是利用光弹晶体的光弹效应,在两个正交方向上产生周期性变化的折射率差,进行光的相位调制,相比于电光调制器、液晶空间光调制器,以及磁光调制器等,其具有调制频率高、通光孔径大、适用波段宽,以及驱动功率小等优点[1-5]。PEM的基本结构是由一个压电晶体和光弹晶体以一定方式胶合而成的,光弹晶体选择各向同性材料,如熔融石英、单晶硅、硒化锌、氟化钙等[6]。在对胶合在光弹晶体上的压电晶体施加一定频率的正弦驱动电压时,由于压电晶体的逆压电效应,其会以相同的频率进行伸缩振动,当压电晶体与光弹晶体的振动频率相匹配后,在光弹晶体内部将产生周期性变化的应力驻波[7],基于光学材料的光弹效应,通过光弹晶体的光将随应力驻波形成周期性变化的双折射,从而改变光的偏振态,实现相位调制[8]。PEM产生的相位延迟量幅值(Am)与光学材料的形变量幅值有关,该形变量的幅值由施加在压电晶体上的正弦驱动电压幅值(Vm)决定。因此,在实际使用中,要准确知道某一特定波长下PEM产生的Am,首先应该知道其与施加的Vm的对应关系,即PEM的定标工作[9]。
目前已有的定标方法,包括基频分量极值法、贝塞尔函数比值法、直流分量法等。基频分量极值法[9]利用一阶贝塞尔函数极值点进行标定,操作简单,但受限于一阶贝塞尔函数的特点。当Am在1.841 rad和5.331 rad附近的一定范围内时,由于贝塞尔函数的变化幅值非常小,因此在该两点附近的一倍频读数变化并不明显,导致不能准确定标到该两点的数值,且仅能对极值点定标。直流分量法[6,10]利用零阶贝塞尔函数与一阶贝塞尔函数的性质,在基频分量极值法的基础上改进而来,通过将变化率不明显的基频分量极值点转换到变化率明显的直流分量上,消去了传统基频分量极值法定标不准确的问题,但该方法的定标范围仍然较窄,仅针对基频分量极值点,并受光源波动影响较大。贝塞尔函数比值法[11-15]利用不同阶贝塞尔函数之间的比值关系进行定标,如四二阶贝塞尔函数比值法,利用
本文提出一种新的光弹调制器定标方法,利用Am=5.136 rad的二次谐波分量零值点作为定标辅助点,将待定标点与定标辅助点间的一阶贝塞尔函数比值作为定标函数,通过测量待定标点与定标辅助点的一次谐波分量比值反推出待定标点的Am值。该方法采用同阶贝塞尔函数比值,排除了探测系统频率响应特性的影响,可以对光弹调制器整个工作区间的Am值进行定标。
2 定标原理
定标系统由电路部分和光路部分组成,其中,光路部分由光源、起偏器、PEM、波片,以及检偏器组成,电路部分由光电探测器、锁相放大器、PEM控制器和计算机组成,系统结构如

图 1. 定标系统及各器件取向(与x轴夹角)
Fig. 1. Calibration system and orientation of each device (angle with x-axis)
光源使用中心波长为653 nm的半导体二极管激光器(LD),峰值半高宽为2 nm,起偏器和检偏器选用消光比大于105∶1的格兰泰勒棱镜,探测器为半导体的硅光伏探测器,选用Hinds公司II/FS50型PEM及其控制器,通过控制器控制电压(vm)实现对PEM幅值电压Vm的调控,锁相放大器以控制器信号频率为参考频率,将探测器信号分频后输出至计算机。以光弹调制器应力调制方向为x轴,起偏器、检偏器透光方向与x轴夹角分别为P、A,波片快轴方向与x轴夹角为θ。激光器光源发出的激光经自带的非球面透镜准直后依次经过起偏器、PEM、波片及检偏器,最后由光电探测器接收,该过程可利用琼斯矩阵进行描述[16]。激光经过起偏器后的线偏振光由琼斯矢量表示为
式中:I0为线偏振光光强;P为起偏器透光轴与x轴夹角。
应力轴方向与x轴平行的光弹调制器的琼斯矩阵为
式中:δ为光弹调制器在x、y轴方向上产生的相位延迟量差,且δ=Amsin ωt[17],Am为相位延迟量幅值,ω是PEM调制频率,Am可表示为
式中:d为PEM厚度;λ为入射激光波长;k为PEM光学应力常数以及压电晶体与光弹晶体之间耦合效率有关的系数[17];Vm为压电晶体峰值驱动电压。由
式中:∆为波片慢、快轴间的相位延迟量差。
透光轴与x轴夹角为A的检偏器的琼斯矩阵为
从检偏器出射的线偏振光的琼斯矢量可表示为
由探测器得到的光强可表示为
根据贝塞尔公式:
将
式中:T1、T2为与探测器、锁相放大器组成的探测系统的频率响应相关的系数。
根据贝塞尔函数性质,二阶贝塞尔函数J2(Am)在Am=5.136 rad时数值为零,如
另外,由
因此,根据
由
3 分析与讨论
按照
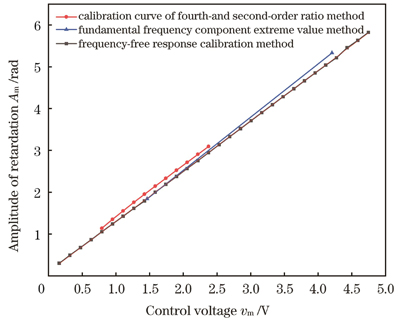
图 3. 四二阶比值法、基频分量极值法,以及无频响定标法校准曲线
Fig. 3. Calibration curve of fourth- and second-order ratio method, fundamental frequency component extreme value method and frequency-free response calibration method
如
为对比不同定标方法间的区别,利用现有的四二阶贝塞尔函数比值法和基频分量极值法分别进行定标,得到定标曲线如
对于所提方法的可行性,利用半波平顶法进行验证。半波平顶法是指当PEM产生的相位延迟量幅值Am为π rad或λ/2,即半波条件时,示波器上会出现上平下尖的特殊波形;当Am略大于π rad时,波形会出现上凹下尖的特点;当Am略小于π rad时,波形则会出现上圆下尖的特点。利用Mathematica软件对

图 4. 仿真曲线。(a)Am设定为π rad时的仿真曲线;(b)Am设定为3.106 rad时的仿真曲线;(c)Am设定为3.178 rad时的仿真曲线
Fig. 4. Simulation curves. (a) Simulation curve when Am is set to π rad; (b) simulation curves when Am is set to 3.106 rad; (c) simulation curve when Am is set to 3.178 rad
利用半波平顶法对定标拟合曲线中的π rad相位延迟量幅值进行验证。已知新方法得到的定标拟合曲线为Am=1.203vm+0.098,产生π rad的相位延迟量幅值对应的控制电压为2.53 V。当控制电压设置为2.53 V时,示波器上出现明显的平顶波形,此时产生的相位延迟量幅值为π rad,如
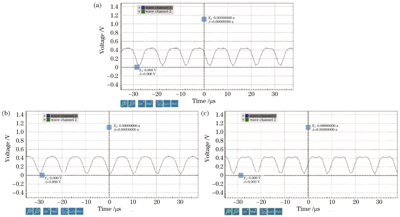
图 5. 不同控制电压下的波形。(a)控制电压为2.53 V(对应Am为π rad)时产生的平顶波形;(b)控制电压为2.5 V(对应Am为3.106 rad)时产生的上圆下尖波形;(c)控制电压为2.56 V(对应Am为3.178 rad)时产生的上凹下尖波形
Fig. 5. Waveforms under different control voltages. (a) Flat-top waveform generated when the control voltage is 2.53 V (corresponding to Am= π rad); (b) the upper circle and lower sharp waveform generated when the control voltage is 2.5 V (corresponding to Am=3.106 rad); (c) the concave and downward tip waveform generated when the control voltage is 2.56 V (corresponding to Am=3.178 rad)
对所使用的LD激光器进行连续30 min的输出光强采集,每次采集间隔为30 s,得到该激光器在30 min内的最大光强波动为0.385%,并依此计算了该量值的光强波动对Am定标偏差的影响,如

图 6. 定标曲线。(a)带有各点定标偏差的原始定标曲线;(b)由光强波动引起的Am定标偏差曲线
Fig. 6. Calibration curves. (a) Original calibration curve with calibration deviation per point; (b) Am calibration deviation curve caused by light intensity fluctuations
4 结论
本文提出了一种新的光弹调制器定标方法。该方法将二阶贝塞尔函数的零值点作为辅助定标点,利用待定标点与辅助定标点间基频分量幅值之比对应于一阶贝塞尔函数比的特点,实现了对PEM整个工作区间的定标。实验结果表明,除基频分量极值点(1.841 rad和5.331 rad)附近外,vm与Am之间的定标关系曲线符合线性关系,且定标结果通过了半波平顶法的验证,由光强波动引起的定标偏差除贝塞尔函数极值点附近,均小于1%。所提定标方法不受探测系统频率响应影响,对波片、起偏器、检偏器的方位角及波片相位延迟量无特殊数值及精度要求,操作简单、鲁棒性强,实现了对PEM在整个Vm工作区间的定标。
[1] 武燕婷, 熊伟, 李超波, 等. 光弹调制器谐振特性的研究及验证[J]. 光学学报, 2021, 41(15): 1523002.
[2] 张跃国. 基于弹光效应的静态傅里叶变换干涉具设计[D]. 太原: 中北大学, 2011.
ZhangY G. Design of static FTIR Interferometer with Photoelastic-effect[D]. Taiyuan: North University of China, 2011.
[3] 吴也, 胡朝晖. 光弹调制器的振动分析与实验[J]. 压电与声光, 2017, 39(5): 665-668.
Wu Y, Hu C H. Vibration analysis and experiment on photoelastic modulator[J]. Piezoelectrics & Acoustooptics, 2017, 39(5): 665-668.
[4] 臧晓阳, 李克武, 王志斌, 等. 快轴可调弹光调制器闭环稳定控制研究[J]. 激光与光电子学进展, 2023, 60(7): 0726003.
[5] 梁振坤, 李晓, 王志斌, 等. 基于驱动电压自适应调节的弹光调制[J]. 中国激光, 2021, 48(11): 1104001.
[6] 周军, 苏桂英, 李国华. 光弹调制器定标新方法[J]. 光子学报, 2001, 30(1): 81-85.
Zhou J, Su G Y, Li G H. A new method for calibration of photoelastic modulator[J]. Acta Photonica Sinica, 2001, 30(1): 81-85.
[7] 武燕婷, 熊伟, 李超波. 光弹调制器研究综述及原理验证[J]. 压电与声光, 2021, 43(3): 352-358.
[8] Hipps K W, Crosby G A. Applications of the photoelastic modulator to polarization spectroscopy[J]. The Journal of Physical Chemistry, 1979, 83(5): 555-562.
[9] 曾爱军, 王向朝, 李代林, 等. 精确标定光弹调制器的新方法[J]. 光学学报, 2005, 25(6): 799-802.
[10] Gu L Y, Wang J, Zhu L L, et al. Calibration of photoelastic modulator using direct current component[J]. Optik, 2017, 140: 304-307.
[11] Wang S, Han X, Wang Y N, et al. Dispersion of the retardation of a photoelastic modulator[J]. Applied Sciences, 2019, 9(2): 341.
[12] Xing B Z, Ding M, Lu J X, et al. Calibration for phase retardation of photoelastic modulator based on compound Bessel function[J]. Optik, 2020, 206: 164322.
[13] Wang M W, Chao Y F, Leou K C, et al. Calibrations of phase modulation amplitude of photoelastic modulator[J]. Japanese Journal of Applied Physics, 2004, 43(2): 827-832.
[14] Wang M W, Tsai F H, Chao Y F. In situ calibration technique for photoelastic modulator in ellipsometry[J]. Thin Solid Films, 2004, 455/456: 78-83.
[15] 李克武, 王爽. 利用反馈光路的弹光调制器定标及稳定控制[J]. 应用光学, 2022, 43(5): 935-942.
[16] 沃新能. 矩阵光学导论[J]. 光学学报, 1983, 3(4): 350-368.
Wo X N. Introduction to matrix optics[J]. Acta Optica Sinica, 1983, 3(4): 350-368.
[17] 刘梓良, 王志斌, 李克武, 等. 一种弹光调制器精确定标微系统设计[J]. 光学技术, 2022, 48(1): 8-13.
杨军营, 韩培高, 魏莹莹. 无频响影响的光弹调制器定标新方法[J]. 中国激光, 2024, 51(8): 0804007. Junying Yang, Peigao Han, Yingying Wei. New Calibration Method for Photoelastic Modulator Without Frequency Response[J]. Chinese Journal of Lasers, 2024, 51(8): 0804007.