Simple real-time high-sensitivity heterodyne coherent optical transceiver at intraplane satellite communication
1. Introduction
The satellite optical communication network has become a promising core network structure and has attracted many commercial companies, including SpaceX, OneWeb, and Telesat. It aims to deploy thousands of low Earth orbit (LEO) satellites to provide high-speed and low-latency global broadband services[1–3]. Up till now, many satellite optical communication field tests have been successfully demonstrated, such as LEO-GND[4,5], LEO-LEO[6], LEO-GEO[7], and GEO-GND[8]. Based on these, several satellite optical network programs have been raised[9,10].
Meanwhile, with the introduction of coherent optical techniques, it is possible to realize large-capacity satellite communication[11–14]. In Ref. [15], a 160 Gbps single-channel polarization-division multiplexing—quadrature phase-shift keying (PDM-QPSK) intersatellite transmission at 40,000 km has been simulated. And in Ref. [16], a 100 to 600 Gbps 16-QAM WDM system over 5000 km transmission was also verified through software simulation. As for practical tests, in Ref. [17], a 13.16 Tbps 54-channel DWDM free-space optical data transmission over 10.45 km was demonstrated. While in a recent report, a single-carrier 0.94 Tbps with polarization-multiplexed high-order complex modulation formats over 53.42 km transmission has been realized by employing a full adaptive optics system[18]. However, besides the communication performance, the costs for the design of satellite transceivers should also be considered. Traditional coherent optical transceivers could be costly in an LEO scenario. Yet the low-cost IM/DD system, another transceiver structure widely used in the LEO-GND scenario, cannot provide enough sensitivity in some long-distance LEO-LEO communication situations. Therefore, in order to establish a network consisting of thousands of satellites, it is essential to further simplify the coherent transceiver design.
In this Letter, we propose an advanced and simple scheme of coherent optical transceiver for satellite communication without digital-to-analog converters (DACs) and an optical phase lock loop (OPLL). In our scheme, the DACs at the transmitter are replaced by a pair of DC blocks. On the other hand, a low-noise erbium-doped optical fiber amplifier (EDFA) is used as the pre-amplifier and a heterodyne receiver is used to detect the signal. The target link budget is analyzed, and the numerical receiver noise model is discussed. Through real-time experimental results, the bit error rate (BER) of the 5 Gbps QPSK signal can reach below the hard-decision forward-error-correction (HD-FEC) threshold of at the received optical power (ROP) of . Based on our proposed scheme, the DAC is not needed, and the heterodyne receiver is applied in place of the common homodyne or intradyne receiver. This not only reduces the system cost but also shows a comparable sensitivity performance. And the least ADC bit-width requirement is further discussed through offline processing to simplify the transceiver. We believe that our proposed scheme is a promising design for intersatellite optical communication.
2. System Setup Design
2.1. Link budget analysis
To discuss the link budget of intersatellite optical communication, the average ROP can be estimated as[19,20]
The Tx optical antenna gain can be calculated by
The free-space link loss is given by
The Rx optical antenna gain is shown by
The pointing loss is estimated by
As shown in Table 1, the intraplane link distance can be roughly summarized as between 1000 and 5000 km. Taking into consideration other parameters in Table 2, the theory ROP shown in Fig. 1 ranges from to . Therefore, the basic sensitivity requirement should be at least below .
Table 1. Link Distances for Intraplane Satellite Links[21]
|
Table 2. Link Parameters
|
2.2. Transceiver scheme
The block diagram of the proposed scheme is represented in Fig. 2(a). The transmitter is composed of an external cavity laser (ECL), an IQ modulator, a modulator bias controller (MBC), an EDFA booster, two DC blocks, and two driving amplifiers. The transmitted bits are directly output from the service processor without pulse formation. The DAC is replaced by a pair of DC blocks. Two driving amplifiers are used to amplify the electrical signal, which is ready to be modulated through the IQ modulator. The MBC here is used to control the bias of the IQ modulator. The modulated optical signal is then boosted by the EDFA and sent to the Tx optical antenna. The receiver side consists of an ECL, an EDFA (which acts as a pre-amplifier), an optical bandpass filter (BPF), and a coherent receiver. The received signal is amplified by a low-noise EDFA. Extra-band noise induced from the EDFA is filtered by a normal WDM optical filter. After being detected by a commercial integrated coherent receiver (ICR), the electrical signal is sampled by an ADC and processed by the digital signal processing (DSP) module. The optical part prototype (without EDFAs) is shown in Fig. 2(b).
In the traditional coherent optical transceiver used in satellite communication systems, the DAC is indispensable. However, the DAC is expensive and has a large power consumption. In addition, different from normal homodyne receivers, we choose the heterodyne structure to decrease the application of the OPLL. The OPLL is a complicated backfeed design that needs precise control, which increases the cost of the transceiver. Thanks to our proposed scheme, the DAC and OPLL are no longer required.
For frequency offset, since the Doppler shift in the intraplane scenario is relatively small, actual frequency offset is simply estimated by the frequency recovery module in Rx DSP. If the offset exceeds our DSP compensation range of , the local oscillator (LO) wavelength will be adjusted by varying the current of Rx ECL (LO) temperature controller according to a pre-made control instruction. And as long as the offset is within , it can be adjusted automatically.
2.3. Receiver noise analysis
The electric field of the received signal can be given by
The LO is expressed as
As shown in Fig. 2(a), in our designed heterodyne receiver, the expression of the output photocurrent is written as
The average power of the photocurrent is given as
On the other hand, the noise of each pair of BPDs mainly comes from three aspects[22].
The total noise of the photocurrent is the sum of the three kinds of noise above,
Then we get the signal-to-noise ratio (SNR) expression,
3. Experimental Setup and Results
The experimental setup of our proposed scheme is depicted in Fig. 3. The Tx and Rx laser diodes are both ECL with 10 kHz linewidth and 10 dBm optical power. PRBS15 bit sequence is generated by a field-programmable gate array (FPGA) without pulse shaping. After the DC blocks, the eye diagram and real-time spectrum are captured by a real-time digital storage oscilloscope (DSO) and are shown in the illustrations in Fig. 3. After modulation, the optical signal is then sent to a VOA to simulate the transmission power loss, taking into consideration that the intersatellite link is an ideal channel. A 50:50 optical splitter (OS) is used to observe the ROP.
At the receiver side, as demonstrated in Section 2.2, after being pre-amplified by a low-noise EDFA, the signal is converted to electrical domain by a commercial coherent receiver with 25 GHz bandwidth. The electrical signal is sampled and quantized by a 10 GSa/s ADC with 8-bit bit-width. It is worth noting that the system is all-polarization-maintained, and there is no extra polarization-induced signal damage. Important experimental details are shown in Table 3.
Table 3. Experimental Parameters
|
The digital samples captured from the ADC are processed in an FPGA, where a set of DSP subsystems is applied to compensate for the channel noise and retrieve the transmitted signal information. The first stages of DSP are aimed at frequency recovery, including downconversion and frequency offset compensation. Next, the symbol synchronization is performed with the inserted pilots, which are the fixed 16 QPSK symbols sequence. After downsampling from the synchronization, carrier-phase recovery is realized. In Fig. 4, the error vector magnitude (EVM) is evaluated between the recovered constellation and the standard QPSK constellation. As the ROP decreases from to , the constellation points become more dispersed, and the EVM increases by 9.88%. Further, we calculated the , and the theoretical value here is deduced according to Section 2.3. The is improved with the increase of the ROP. It can be seen that there is a deviation of about 2 dB between the theoretical value and our platform test result. This could be due to the additional loss caused by sampling quantization error and imperfect DSP recovery.
To reflect the communication performance directly, we calculate the real-time bidirectional communication BER performance according to Figs. 5(a) and 5(b). Figure 6 shows the sensitivity curve to achieve a target BER of (HD-FEC limit). From the results, the sensitivity of our designed transceiver reaches with 5 Gbps QPSK. It perfectly satisfies the real intersatellite optical communication scenario discussed in Section 2.1, leaving an extra 5 dB redundancy. And if higher performance FEC code like low density parity check (LDPC) code is applied, the sensitivity of this transceiver could be decreased to and below. In addition, the average power consumption of our prototypes is stable at 43–44 W during the bidirectional communication test. Moreover, taking the reality that the ROP may be below into account, we provide two additional data rates, 2.5 Gbps BPSK and 1.25 Gbps BPSK, with the minimum sensitivity of about for backup.
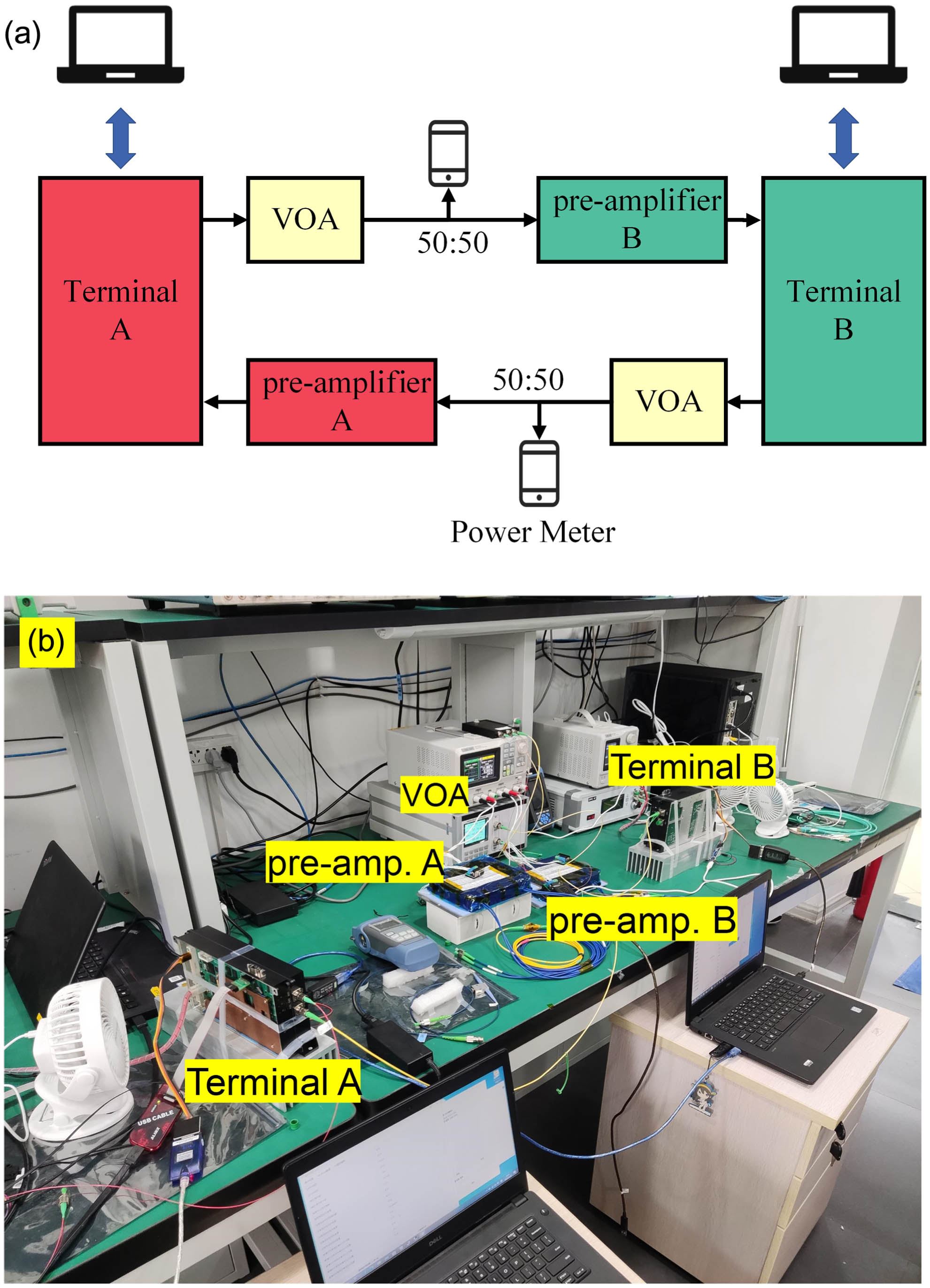
Fig. 5. Real-time bidirectional experimental setup. (a) Block diagram; (b) indoor ob2b experimental environment.
Furthermore, based on the sampling before, we discuss the minimum required ADC bit-width of the transceiver. Through offline DSP, the results of the BER versus the ROP under different sampling bit-widths are shown in Fig. 7. When the bit-width decreases, the sensitivity performance becomes significantly worse. And the limit ADC bit-width is 4-bit with sensitivity of , which satisfies the basic requirement of the real link discussed in Section 2.1. To some extent, this can save the power and DSP resource consumption and reduce the component cost.
In Table 4, several real-time free-space optical (FSO) coherent communication transceivers are listed. To evaluate the sensitivity performance fairly, the results are discussed with respect to photons per bit (PPB). It can be found that the homodyne system has the advantage of extra-low sensitivity at a relatively low data rate. However, the BPSK homodyne transceiver requires the OPLL, which increases the complexity and cost. In order to avoid applying the OPLL, an autodyne transceiver can be a choice in the DPSK system. Yet when the modulation order increases, the intradyne transceiver, also known as a digital homodyne transceiver, becomes the mainstream design but needs a pair of DACs to output the Tx signal for the pulse-forming process. Compared with other published work, our real-time heterodyne transceiver without DAC and OPLL exhibits a comparable sensitivity with other systems and further reduces the cost. In the future, higher data rates will be required for commercial satellite optical communication. And based on our scheme, high-order modulation like QAM-16 or QAM-64 with the same baud rate as the prototype could be a promising way to increase the transmission rate without too much hardware change.
Table 4. Performance of Different Published Real-Time FSO Coherent Transceivers
|
Reference [24] does not mention sensitivity directly; here, we deduce the result from the transmission distance of about 3700–4700 km according to Section 2.1. The real sensitivity performance could be better.
4. Conclusion
In this paper, a novel scheme of high-sensitivity heterodyne optical transceiver without DAC and OPLL for intraplane satellite communication is proposed and experimentally demonstrated. The analytical and experimental results prove the feasibility of our proposed scheme. Based on our scheme, 5 Gbps QPSK real-time bidirectional communication is realized with a sensitivity of at the HD-FEC limit. And we prove that the Rx ADC bit-width can be further reduced to 4-bit. Moreover, our heterodyne transceiver without DAC and OPLL reduces the system cost significantly. We believe the proposed transceiver is a promising component for intersatellite optical communication.
Article Outline
Yuanzhe Qu, Qianwu Zhang, Yanyi Wang, Yanhao Chen, Lewei Gong, Ziyue Liu, Junjie Zhang, Yingchun Li, Jian Chen, Yingxiong Song. Simple real-time high-sensitivity heterodyne coherent optical transceiver at intraplane satellite communication[J]. Chinese Optics Letters, 2024, 22(3): 030601.