液晶的多维度光场调控技术研究进展
下载: 906次特邀综述
As a class of soft functional materials that exhibit both liquid and solid-like properties, liquid crystals can simultaneously show fluidity and crystal anisotropy, and have been widely applied in the fields of image display, integrated optoelectronics, optical communication, and others. In the past decades, with the development of the liquid crystal theory and processing technology, the research on liquid crystals has gradually matured from the conventional display to advanced liquid crystal photonics, which is of great significance for the realization of integrated, intelligent, and miniaturized optical systems. Liquid crystals can exhibit abundant phase states, including smectic, nematic, cholesteric, and blue phases. Each phase state has its unique properties and can be applied in different fields, including tunable optical devices and polarization converters. Therefore, the light modulation characteristics of liquid crystals, including their amplitude, phase, and polarization, are investigated. Based on these properties, a variety of liquid crystal devices can be designed to replace the conventional refraction optical elements, such as gratings, focusing lenses, spiral phase plates, beam steering devices, and holographic imaging devices. For the successful fabrication of devices, liquid crystal molecules should be controlled precisely in terms of the friction orientation, tilt evaporation orientation, and photoalignment. Each technology has its unique advantages from the perspective of different applications. In addition, liquid crystals can be dynamically controlled by varying the electric field, magnetic field, or temperature. The use of liquid crystals in the field of lasers and metasurfaces is of great significance to prepare tunable liquid crystal micro-laser and liquid crystal metasurface composite devices. Therefore, it is extremely important and necessary to summarize the liquid crystal modulation techniques to guide the future development of this field.
This paper summarizes the research progress in multi-dimensional light modulation enabled by liquid crystals. The light modulation characteristics of liquid crystals are derived from their birefringence properties through the Jones matrix (Fig. 2). A variety of photoalignment technologies, including mask exposure, interference exposure, direct write exposure, plasma mask exposure, and dynamic mask exposure, are presented (Fig. 3). According to the light modulation characteristics of liquid crystals, liquid crystal devices are classified into amplitude, phase, and complex amplitude types. Amplitude-type liquid crystal devices include liquid crystal displays, amplitude-type spatial light modulators, and liquid crystal smart windows (Figs. 5-7). Phase-type liquid crystal devices include phase-type spatial light modulators and geometric phase-type liquid crystal devices. The researchers developed an orbital angular momentum holographic encryption system based on a phase-type spatial light modulator. For geometric-phase liquid crystal devices, some geometric-phase nematic liquid crystal devices are first introduced. In order to overcome the low polarization conversion efficiency of nematic liquid crystals, the researchers from Nanjing University obtained a chiral flip controllable self-assembled spiral superstructure by mixing a photosensitive molecular machine with a chiral agent with opposite chirality. An optically controlled transformable optical device with a continuously adjustable working band and geometrically conjugate phase was realized (Fig. 10). By introducing the extension of Marius' law to the orientation arrangement of liquid crystal molecules, the researchers from Wuhan University realized the independent regulation of the amplitude and phase of light waves, realizing a breakthrough in ultra-compact multifunctional liquid crystal devices (Fig. 12). Based on the Jones matrix, the researchers from Hunan University found that binary phase modulation also contributes to continuous amplitude modulation and realized a complex amplitude liquid crystal device (Figs. 13 and 14). The concept of metasurface has attracted considerable attention in recent years. However, once the structure of the metasurface is determined, it is difficult to realize dynamic modulation. To overcome this situation, researchers have combined metasurfaces with liquid crystals to achieve dynamic control of the optical properties of the integrated system (Figs. 15 and 16). Benefiting from the liquid, self-assembling, and tunable properties of liquid crystals, researchers have developed liquid crystal-based micro-lasers (Fig. 17). In addition, other light modulation applications enabled by liquid crystals, including nonlinear optical effects and optical angular momentum modulation, have been discovered (Figs. 18 and 19).
With the development of the liquid crystal research theory and fabrication/processing technologies, different light-modulation technologies based on liquid crystals have been developed for various optical applications ranging from conventional display amplitude modulation to geometric phase modulation, and complex amplitude modulation, static to dynamic modulation, and single-function to multi-function integration. This paper outlines the recent research progress of multi-dimensional light modulation based on liquid crystals, especially the design and processing of multifunctional liquid crystal devices. At the same time, combining a dynamically controllable liquid crystal with a metasurface to form a composite structure can integrate more functions in a single device. The field of liquid crystals also faces certain issues in aspects ranging from structural design to device fabrication, such as how to achieve multifunctional multilayer integrated liquid crystal devices, broadband achromatic liquid crystal devices, polarization-independent liquid crystal devices, vertical chromatic aberration correction of liquid crystal devices, and zoom liquid crystal lenses. Therefore, it is necessary to focus on the above areas, and we believe that optical devices based on liquid crystals will play an increasingly important role in optical encryption, image display, optical communication, and data storage.
1 引言
液晶是一种介于液体与晶体之间的物质,同时表现出液体流动性和晶体的各向异性[1-2],由于其优异的光电特性而被广泛应用于平板显示领域,如手机、计算机、液晶电视等。随着液晶理论研究的深入和加工技术的不断发展,液晶对于实现集成化、智能化和小型化的光学系统具有越来越重要的意义。近年来,研究人员利用液晶对偏振、振幅、相位等多维参数进行调控,构建了一系列平面功能型液晶器件,如聚焦透镜、螺旋相位板、光栅、光束转向器等[3-6],如
![平面功能性液晶器件。(a)菲涅耳液晶透镜[3];(b)液晶螺旋相位板[4];(c)可调谐液晶光栅[5];(d)液晶红外光束转向器[6]](/richHtml/zgjg/2023/50/18/1813006/img_01.jpg)
图 1. 平面功能性液晶器件。(a)菲涅耳液晶透镜[3];(b)液晶螺旋相位板[4];(c)可调谐液晶光栅[5];(d)液晶红外光束转向器[6]
Fig. 1. Planar functional liquid crystal devices. (a) Fresnel liquid crystal lens[3]; (b) liquid crystal spiral phase plate[4]; (c) tunable liquid crystal grating[5]; (d) liquid crystal beam steering device[6]
液晶有着丰富的相态,包括近晶相、向列相、胆甾相、蓝相等。对于近晶相液晶(SLC),其液晶分子长轴相互平行,呈层状结构,分子在每一层内容易流动,但难以在层间移动,如
![液晶分子排列示意图。(a)近晶相液晶[26];(b)向列相液晶[26];(c)胆甾相液晶[26];(d)蓝相液晶[24]](/richHtml/zgjg/2023/50/18/1813006/img_02.jpg)
图 2. 液晶分子排列示意图。(a)近晶相液晶[26];(b)向列相液晶[26];(c)胆甾相液晶[26];(d)蓝相液晶[24]
Fig. 2. Schematics of liquid crystal molecule arrangement. (a) Smectic liquid crystal[26]; (b) nematic liquid crystals[26]; (c) cholesteric liquid crystal[26]; (d) blue phase liquid crystal[24]
本文首先从液晶的双折射特性入手,基于琼斯矩阵推导了液晶调控光波振幅和相位等的特性。随后讨论了摩擦取向、光控取向等液晶取向技术的发展,介绍了几种常见的光控取向技术。然后从液晶振幅调控器件、液晶相位调控器件、液晶复振幅调控器件、液晶-超表面动态调控器件四个方面,详细地讨论了基于液晶器件的多维度光场调控的最新研究进展和应用,并且讨论了相应器件在光学加密系统中的应用。
2 液晶的光场调控特性
2.1 双折射特性
如
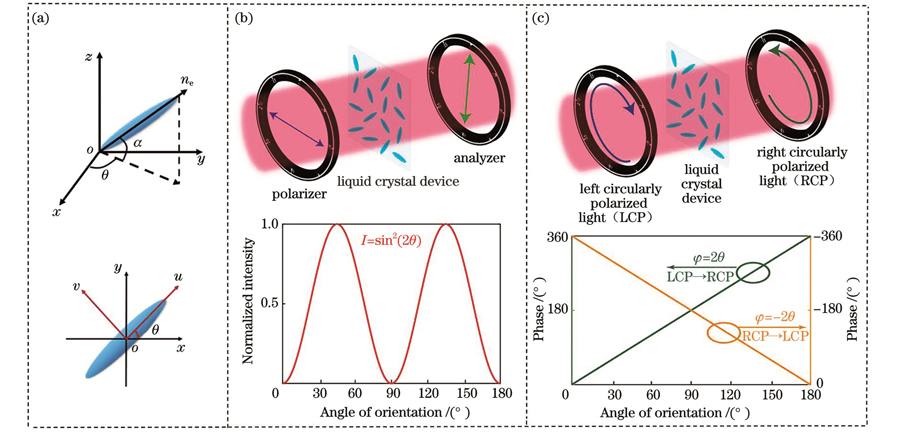
图 3. 液晶分子调控机理。(a)液晶分子的结构示意图;(b)液晶的振幅调控特性;(c)液晶的几何相位调控特性
Fig. 3. Molecular modulation mechanism of liquid crystal. (a) Structural diagram of liquid crystal molecular; (b) amplitude modulation properties of liquid crystals; (c) geometric phase modulation properties of liquid crystals
当液晶分子与xy平面存在夹角即面外倾斜角(α)时,此时的等效双折射率(
式中:neeff表示等效的液晶材料的折射率。此时,光透过液晶后的光程差(
式中:d为液晶材料的厚度;λ为光的波长。面外倾斜角α的变化会导致光程差、透射率等参数变化,这是液晶显示器振幅调控的原理。在液晶两端施加电压并且配合线偏振片,最终可产生不同的透射振幅。
2.2 振幅调控
通过控制液晶的面内取向角(θ)也可以实现输出光场的连续调控,我们可以利用琼斯矩阵来推导
式中:
式中:θ表示面内取向角,即液晶分子在xy面上的投影与x轴之间的夹角;
式中:tu和tv是沿液晶分子长短轴(
从
2.3 几何相位调控
几何相位是伴随圆偏振翻转过程出现的相位类型,可以通过旋转液晶面内取向角来实现精确调控。几何相位是Berry等[28-30]提出的,又称为Pancharatnam-Berry(PB)相位。下面根据琼斯矩阵研究几何相位调控。当圆偏振光通过液晶分子后,输出光场表达式为
式中:
3 液晶取向技术发展
液晶取向技术主要有摩擦取向、倾斜蒸镀取向和光控取向等技术[31-35],各项技术在不同的应用场合有其独特的优势。例如,工业上常用摩擦取向技术进行液晶取向层的加工,这种方法具有操作简单、成本低、稳定性高、可靠性好等优点,但也存在一些问题,如摩擦过程中基材表面容易产生缺陷、静电以及弯曲不规则的基材难以取向等。随着材料学的发展,光控取向剂的研发促进了液晶光控取向技术的发展,这种技术具有非接触、高分辨、图案化、可编程、高效率等特点,为液晶光子学和集成光电子学等前沿领域的发展奠定了基础。
目前液晶光控取向技术主要分为掩模版曝光系统、干涉曝光系统、直写曝光系统、等离激元掩模版曝光系统、动态掩模曝光系统等[36-41]。掩模版曝光系统是将掩模版放置在衬底的合适位置,再使用一定偏振方向的光进行取向处理,被取向光照射的部分材料记录下了光的偏振信息,而未被照射部分保持原样,如
![各种光取向技术系统。(a)掩模版曝光系统[40];(b)干涉曝光系统[39];(c)直写曝光系统[37];(d)DMD动态掩模曝光系统[38];(e)等离激元掩模版曝光系统[41];(f)SLM动态掩模曝光系统[36]](/richHtml/zgjg/2023/50/18/1813006/img_04.jpg)
图 4. 各种光取向技术系统。(a)掩模版曝光系统[40];(b)干涉曝光系统[39];(c)直写曝光系统[37];(d)DMD动态掩模曝光系统[38];(e)等离激元掩模版曝光系统[41];(f)SLM动态掩模曝光系统[36]
Fig. 4. Various optical orientation technology systems. (a) Mask exposure system[40]; (b) interference exposure system[39]; (c) direct write exposure system[37]; (d) DMD dynamic mask exposure system[38]; (e) plasmon mask exposure system[41]; (f) SLM dynamic mask exposure system[36]
4 液晶器件及其应用
4.1 振幅型液晶器件
4.1.1 液晶显示器
液晶振幅调控最广泛的应用便是液晶显示器[42-45]。液晶显示器最常见的类型之一是扭曲向列(TN)型显示器[43]。以TN型显示器为例,如
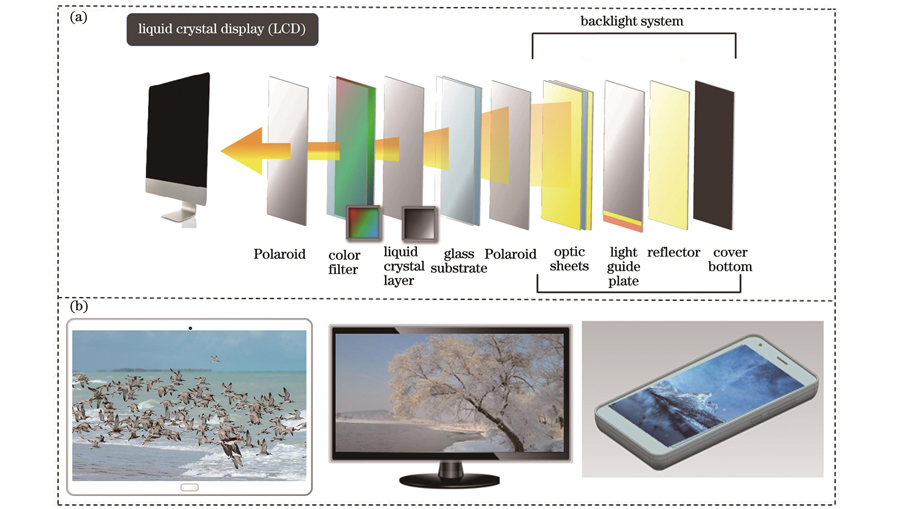
图 5. 液晶显示器的原理与应用。(a)液晶显示器的结构示意图;(b)液晶显示器的应用
Fig. 5. Principle and applications of liquid crystal display. (a) Structural diagram of liquid crystal display; (b) applications of liquid crystal display
4.1.2 振幅型空间光调制器
振幅型空间光调制器由一系列独立的单元组成,可以通过控制这些单元来实现光波的振幅调控,其调控机理与液晶显示器相似,也是在液晶盒两端施加一定外电压,使液晶分子发生倾斜,即液晶分子的面外倾斜角α发生变化,进而透过液晶盒的线偏光的偏振态发生改变,线偏光的偏振方向与检偏器的偏振方向不再平行,出射光强也相应发生变化,如
![振幅型空间光调制器原理及应用。(a)Holoeye LC2012振幅型空间光调制器及其原理[48];(b)利用空间光调制器实现激光光束整形[49];(c)基于空间光调制器测试折射率的可调折光仪示意图[50]](/richHtml/zgjg/2023/50/18/1813006/img_06.jpg)
图 6. 振幅型空间光调制器原理及应用。(a)Holoeye LC2012振幅型空间光调制器及其原理[48];(b)利用空间光调制器实现激光光束整形[49];(c)基于空间光调制器测试折射率的可调折光仪示意图[50]
Fig. 6. Principle and applications of amplitude type spatial light modulator. (a) Holoeye LC2012 amplitude type spatial light modulator and its principle[48]; (b) laser beam shaping realized by using space light modulator[49]; (c) schematic of adjustable refraction instrument for measuring refractive index based on spatial light modulator[50]
4.1.3 液晶智能窗
液晶智能窗是一种基于液晶振幅调控技术的智能建筑材料,它可以通过控制液晶分子的取向和排列方式,调节窗户的透明度和反射率[57-59]。2017年,Wang等[60]设计了一种基于液晶-石墨烯复合材料的智能窗系统,该系统可以利用多种外部刺激改变智能窗的光学透明度,如
![基于液晶的智能窗。(a)基于液晶/石墨烯复合材料的智能窗示意图[60];(b)基于聚合物材料液晶的稳定可扩展的智能窗示意图[61]](/richHtml/zgjg/2023/50/18/1813006/img_07.jpg)
图 7. 基于液晶的智能窗。(a)基于液晶/石墨烯复合材料的智能窗示意图[60];(b)基于聚合物材料液晶的稳定可扩展的智能窗示意图[61]
Fig. 7. Smart windows based on liquid crystal. (a) Schematic of smart window based on liquid crystal/graphene composites[60]; (b) schematic of stable and expandable smart window based on polymer material liquid crystal[61]
4.2 相位型液晶器件
4.2.1 相位型空间光调制器
相位型空间光调制器主要通过控制传输相位来调控入射光的相位[62-63]。如
![相位型空间光调制器原理及应用。(a)Holoeye PLUTO-2相位型空间光调制器示意图及其原理;(b)基于空间光调制器的可扩展性全光神经网络[64];(c)基于相位型空间光调制器的加密系统[65]](/richHtml/zgjg/2023/50/18/1813006/img_08.jpg)
图 8. 相位型空间光调制器原理及应用。(a)Holoeye PLUTO-2相位型空间光调制器示意图及其原理;(b)基于空间光调制器的可扩展性全光神经网络[64];(c)基于相位型空间光调制器的加密系统[65]
Fig. 8. Principle and applications of phase type spatial light modulator. (a) Holoeye PLUTO-2 phase type spatial light modulator and its principle; (b) scalable all-optical neural network based on spatial optical modulator[64]; (c) encryption system based on phase type spatial light modulator[65]
2020年,Fang等[66]将光学轨道角动量(OAM)作为全息信息载体,提出了全新的OAM全息技术,设计了基于空间光调制器的轨道角动量全息加密系统,如
![基于空间光调制器的动态加密系统。(a)基于空间光调制器的轨道角动量全息加密[66];(b)基于空间光调制器的空间非线性光学图像加密[67]](/richHtml/zgjg/2023/50/18/1813006/img_09.jpg)
图 9. 基于空间光调制器的动态加密系统。(a)基于空间光调制器的轨道角动量全息加密[66];(b)基于空间光调制器的空间非线性光学图像加密[67]
Fig. 9. Dynamic encryption systems based on spatial light modulator. (a) Holographic encryption of orbital angular momentum based on space light modulator[66]; (b) spatial nonlinear optical image encryption based on spatial light modulator[67]
4.2.2 几何相位器件
液晶的几何相位器件主要是基于几何相位的宽带相位调制和液晶的低耗损高偏振转换效率来调控光场。2016年,Duan等[68]基于几何相位通过光取向技术制备了一个双频液晶偏振光栅,并验证了其作为光学开关的作用,如
![几何相位型液晶器件。(a)基于几何相位的双频液晶偏振光栅[68];(b)基于几何相位的偏振切换型液晶透镜[69];(c)基于几何相位的液晶双焦透镜[70];(d)不同相位分布下的胆甾相液晶[71];(e)可光控手性翻转的自组装螺旋超结构[72]](/richHtml/zgjg/2023/50/18/1813006/img_10.jpg)
图 10. 几何相位型液晶器件。(a)基于几何相位的双频液晶偏振光栅[68];(b)基于几何相位的偏振切换型液晶透镜[69];(c)基于几何相位的液晶双焦透镜[70];(d)不同相位分布下的胆甾相液晶[71];(e)可光控手性翻转的自组装螺旋超结构[72]
Fig. 10. Geometric phase liquid crystal devices. (a) Dual-frequency liquid crystal polarization grating based on geometric phase[68]; (b) polarization switching liquid crystal lens based on geometric phase[69]; (c) liquid crystal bifocal lens based on geometric phase[70]; (d) cholesteric liquid crystals under different phase distributions[71]; (e) self-assembling spiral superstructure with optically controlled chiral inversion[72]
2021年,Li等[73]基于胆甾相液晶固有的自组织螺旋结构,设计了一种染料掺杂手性液晶,对圆偏振发光性能进行调谐,如
![基于液晶的动态加密系统。(a)基于染料掺杂手性液晶对可调圆偏振发光信号进行传输的加密系统示意图[73];(b)受虎符启发的高安全性液晶全息加密系统示意图[74]](/richHtml/zgjg/2023/50/18/1813006/img_11.jpg)
图 11. 基于液晶的动态加密系统。(a)基于染料掺杂手性液晶对可调圆偏振发光信号进行传输的加密系统示意图[73];(b)受虎符启发的高安全性液晶全息加密系统示意图[74]
Fig. 11. Dynamic encryption systems based on liquid crystal. (a) Schematic of encryption system for transmission of tunable circularly polarized luminescent signals based on dye-doped chiral liquid crystals[73]; (b) schematic of high security liquid crystal holographic encryption system inspired by Tiger Amulet[74]
2022年,Chen等[75]设计了一种融合平板显示和全息投影的超紧凑多功能液晶器件,该器件可以在液晶表面显示灰度图案,同时利用几何相位在远场显示独立的全息图像,如
![基于几何相位的多功能液晶器件。(a)近远场图像同时显示的多功能液晶器件[75];(b)偏振、波长、位置复用的液晶器件[76]](/richHtml/zgjg/2023/50/18/1813006/img_12.jpg)
图 12. 基于几何相位的多功能液晶器件。(a)近远场图像同时显示的多功能液晶器件[75];(b)偏振、波长、位置复用的液晶器件[76]
Fig. 12. Multifunctional liquid crystal devices based on geometric phase. (a) Multifunctional liquid crystal device for simultaneous display of near-field and far-field images[75]; (b) liquid crystal device with polarization, wavelength, and position multiplexing[76]
4.3 复振幅型液晶器件
相较于纯振幅或相位调控,复振幅调控可以同时调控光波的振幅和相位,从而增加了调控自由度,在多通道通信、聚焦成像和全息成像等领域中具有较大的应用潜力。根据
![具有表面显示和远场全息显示功能的多功能液晶器件[79]。(a)液晶分子在正交偏振光路中的复振幅调控特性;(b)器件设计原理示意图;(c)实验测试结果](/richHtml/zgjg/2023/50/18/1813006/img_13.jpg)
图 13. 具有表面显示和远场全息显示功能的多功能液晶器件[79]。(a)液晶分子在正交偏振光路中的复振幅调控特性;(b)器件设计原理示意图;(c)实验测试结果
Fig. 13. Multifunctional liquid crystal device with surface display and far-field holographic display[79]. (a) Complex amplitude modulation of liquid crystal molecules in orthogonal polarized light paths; (b) schematic of device design principle; (c) experimental test results
2023年,Xie等[80]通过进一步优化近场图像,在两个独立的近场通道中显示了二进制图像,并且没有出现串扰,同时在远场显示了一个全息图像,实现了三通道显示,如
![具有多通道表面图像显示与远场全息显示功能的多功能液晶器件[80]。(a)液晶分子旋转前后的归一化强度与液晶取向角θ的关系;(b)液晶分子的四种取向角及其对应的双通道二进制强度编码;(c)实验测试结果](/richHtml/zgjg/2023/50/18/1813006/img_14.jpg)
图 14. 具有多通道表面图像显示与远场全息显示功能的多功能液晶器件[80]。(a)液晶分子旋转前后的归一化强度与液晶取向角θ的关系;(b)液晶分子的四种取向角及其对应的双通道二进制强度编码;(c)实验测试结果
Fig. 14. Multifunctional liquid crystal device with multi-channel surface image display and far-field holographic display[80]. (a) Relationship between normalized strength of liquid crystal molecules and orientation angle θ before and after rotation; (b) four orientation angles of liquid crystal molecules and their corresponding binary intensity codes; (c) experimental test result
因此将旋转前后每个液晶分子的旋向角和光强进行对应,可获得两个独立的二进制图像;同时,他们通过控制电压来开启或关闭图像,证明了液晶分子的电场可调性。这种技术在多通道图像显示、光学防伪、加密、存储、安全识别、增强现实/虚拟现实(AR/VR)设备等光学领域中具有广阔应用的前景。
4.4 液晶-超表面动态调控器件
超表面可以灵活地调控光波的振幅、相位或偏振等信息[81-82],得到了国内外学者的广泛关注[83-89]。但是,确定的超表面结构存在难以动态调控的问题。利用电场、磁场或温度等变化可以动态调控液晶,因此,将超表面与液晶结合实现光学特性的动态控制具有非常重要的意义。2021年,Li等[90]提出了一种电控的偏振相关加密显示方法,此方法主要利用聚合物分散液晶实现电控开关的效果,为偏振相关的超表面结构色增加了额外的加密自由度,如
![集成液晶与超表面的调控器件。(a)集成聚合物分散液晶和超表面的光学加密系统示意图[90];(b)集成向列相液晶和超表面的器件示意图[91]](/richHtml/zgjg/2023/50/18/1813006/img_15.jpg)
图 15. 集成液晶与超表面的调控器件。(a)集成聚合物分散液晶和超表面的光学加密系统示意图[90];(b)集成向列相液晶和超表面的器件示意图[91]
Fig. 15. Modulator components with integrated liquid crystal and metasurface. (a) Schematic of optical encryption system with integrated polymer dispersed liquid crystal and metasurface[90]; (b) schematic of device with integrated nematic liquid crystal and metasurface[91]
2021年,Hu等[92]利用超表面的偏振操纵能力,设计了一种在可见光范围内与液晶集成的电可调谐多功能偏振相关超表面,如
![液晶-超表面动态调控器件。(a)集成液晶的电可调谐多功能偏振依赖超表面示意图[92];(b)电可调谐液晶负载超表面示意图[93]](/richHtml/zgjg/2023/50/18/1813006/img_16.jpg)
图 16. 液晶-超表面动态调控器件。(a)集成液晶的电可调谐多功能偏振依赖超表面示意图[92];(b)电可调谐液晶负载超表面示意图[93]
Fig. 16. Liquid crystal-metasurface dynamic control devices. (a) Schematic of electrically tunable multifunctional polarimetric dependent metasurface with integrated liquid crystal[92]; (b) schematic of electrically tunable liquid crystal loaded metasurface[93]
4.5 液晶微型激光器
由于液晶的液态、自组装、可调谐等特性,基于液晶的微型激光器吸引了研究人员的关注[94-96]。2022年,Muszyński等[97]将染料分子分散在液晶微腔中,开发了一种540~580 nm范围内可调谐的微型激光器件,且该激光器微腔表面上存在自旋螺旋,激光器可发射两束具有不同偏振角度的圆偏振光,如
![可调谐液晶微型激光器件。(a)宽波段、双光出射液晶激光器件[97];(b)宽温域蓝相液晶激光器件[98]](/richHtml/zgjg/2023/50/18/1813006/img_17.jpg)
图 17. 可调谐液晶微型激光器件。(a)宽波段、双光出射液晶激光器件[97];(b)宽温域蓝相液晶激光器件[98]
Fig. 17. Tunable liquid crystal micro laser devices. (a) Wide-band and dual outgoing liquid crystal laser[97]; (b) blue phase liquid crystal laser with wide temperature domain[98]
4.6 液晶的其他光场调控应用
液晶的光场除了具有振幅、相位、动态可调谐等特性外,在非线性光学[99-101]、光学角动量调控[102-107]等方面也具有优势。近年来,铁电向列相液晶材料的发展为开发液晶的非线性光学特性提供了可能性,其除了具备传统向列相的特征外,还具备超高的介电常数、出色的非线性光学特性及对电场的高响应灵敏度[108-111]。2022年,Folcia等[112]基于铁电向列相液晶材料进行了光学二次谐波产生的研究,发现该材料在透明状态下的非线性磁化率为5.6 pm/V,如
![基于液晶的非线性光场调控。(a)铁电向列相液晶的非线性特性研究[112];(b)基于螺旋铁电向列相液晶的非线性特性系统[113]](/richHtml/zgjg/2023/50/18/1813006/img_18.jpg)
图 18. 基于液晶的非线性光场调控。(a)铁电向列相液晶的非线性特性研究[112];(b)基于螺旋铁电向列相液晶的非线性特性系统[113]
Fig. 18. Nonlinear light field control based on liquid crystal. (a) Study on nonlinear properties of ferroelectric nematic liquid crystals[112]; (b) nonlinear property system based on spiral ferroelectric nematic liquid crystals[113]
2022年,Liu等[114]设计了一种基于几何相位的新型液晶平面光学器件。他们将二值精细结构与渐变几何相位结构集成到一个器件中,获得了偏振可控、模式分布可定制的轨道角动量光束三维阵列,如
![基于液晶器件的光场角动量调控。(a)基于液晶几何相位的轨道角动量光束三维阵列[114];(b)四维度光场可调谐液晶器件[115]](/richHtml/zgjg/2023/50/18/1813006/img_19.jpg)
图 19. 基于液晶器件的光场角动量调控。(a)基于液晶几何相位的轨道角动量光束三维阵列[114];(b)四维度光场可调谐液晶器件[115]
Fig. 19. Control of angular momentum of light field based on liquid crystal devices. (a) Three-dimensional array of orbital angular momentum beams based on geometric phase of liquid crystal[114]; (b) four-dimensional optical tunable liquid crystal device[115]
5 总结与展望
随着液晶理论研究的深入和液晶加工技术的进展,基于液晶的光场调控技术向着多样化、多功能、多维度方向发展,从传统显示中的振幅调控发展到几何相位调控和复振幅调控,从静态调控发展到动态调控,从单功能发展到多功能集成。结合液晶相关技术工艺成熟、成本低、大尺寸等特点,详细介绍了基于液晶的多维度光场调控的研究进展。近年来多功能液晶器件的设计和加工解决了液晶器件功能单一的问题,同时液晶作为一种相对有序的材料,在动态调控方面具有得天独厚的优势,结合液晶动态调控性能的超表面作为复合结构,可以实现更多功能的集成。目前,基于液晶的多维度光场调控技术仍然存在一些尚未解决的问题。这些问题包括如何实现多功能多层集成化液晶器件、宽带消色差液晶器件、偏振无关型液晶器件、液晶器件纵向色差校正、变焦液晶透镜、新型液晶(例如胆甾相液晶)与超表面结合等,同时,在液晶加工过程中,当像素尺寸缩小时需要保证加工精度。因此,需要广大研究人员聚焦上述领域,深入开展相关研究工作。我们相信,基于液晶的光学器件将在光学加密、图像显示、光通信和数据存储等领域中发挥越来越重要的作用。
[1] Brown G H. Structure, properties, and some applications of liquid crystals[J]. Journal of the Optical Society of America, 1973, 63(12): 1505-1514.
[2] Korenic E M. A millennium of liquid crystals[J]. Optics and Photonics News, 2000, 11(1): 16-22.
[3] Huang S J, Li Y, Zhou P C, et al. Polymer network liquid crystal grating/Fresnel lens fabricated by holography[J]. Liquid Crystals, 2017, 44(5): 873-879.
[4] Caño-García M, Quintana X, Otón J M, et al. Dynamic multilevel spiral phase plate generator[J]. Scientific Reports, 2018, 8: 15804.
[5] Li Y L, Li N N, Wang D, et al. Tunable liquid crystal grating based holographic 3D display system with wide viewing angle and large size[J]. Light: Science & Applications, 2022, 11: 188.
[6] Gou F W, Peng F L, Ru Q T, et al. Mid-wave infrared beam steering based on high-efficiency liquid crystal diffractive waveplates[J]. Optics Express, 2017, 25(19): 22404-22410.
[7] Stephen M J, Straley J P. Physics of liquid crystals[J]. Reviews of Modern Physics, 1974, 46(4): 617-704.
[8] Kogelnik H, Li T. Laser beams and resonators[J]. Applied Optics, 1966, 5(10): 1550-1567.
[9] Eringen A C. Electrodynamics of cholesteric liquid crystals[J]. Molecular Crystals and Liquid Crystals, 1979, 54(1/2): 21-43.
[10] Bonneville R. Symmetries of the electrodynamic interactions between chiral molecules[J]. Molecular Physics, 2008, 106(21/22/23): 2627-2642.
[11] Emelyanenko A V. Induction of new ferrielectric smectic phases in the electric field[J]. Ferroelectrics, 2016, 495(1): 129-142.
[12] Carlsson T. Macroscopic flow behaviour of smectic C and smectic C* liquid crystals[J]. Ferroelectrics, 1998, 212(1): 123-131.
[13] Lee S H, Lee S L, Kim H Y. Electro-optic characteristics and switching principle of a nematic liquid crystal cell controlled by fringe-field switching[J]. Applied Physics Letters, 1998, 73(20): 2881-2883.
[14] George A K. Optical anisotropy of nematic liquid crystals[J]. Physics and Chemistry of Liquids, 1998, 37(1): 65-71.
[15] Chen C Y, Hsieh C F, Lin Y F, et al. Magnetically tunable room-temperature 2π liquid crystal terahertz phase shifter[J]. Optics Express, 2004, 12(12): 2625-2630.
[16] Varanytsia A, Weng L B, Lin T C, et al. High-performance and low-cost aluminum zinc oxide and gallium zinc oxide electrodes for liquid crystal displays[J]. Journal of Display Technology, 2016, 12(10): 1033-1039.
[17] Rafayelyan M, Tkachenko G, Brasselet E. Reflective spin-orbit geometric phase from chiral anisotropic optical media[J]. Physical Review Letters, 2016, 116(25): 253902.
[18] Li Y, Liu Y J, Dai H T, et al. Flexible cholesteric films with super-reflectivity and high stability based on a multi-layer helical structure[J]. Journal of Materials Chemistry C, 2017, 5(41): 10828-10833.
[19] Mitov M. Cholesteric liquid crystals with a broad light reflection band[J]. Advanced Materials, 2012, 24(47): 6260-6276.
[20] Lin J D, Lin H L, Lin H Y, et al. Widely tunable photonic bandgap and lasing emission in enantiomorphic cholesteric liquid crystal templates[J]. Journal of Materials Chemistry C, 2017, 5(13): 3222-3228.
[21] Huang Y H, Zhou Y, Wu S T. Broadband circular polarizer using stacked chiral polymer films[J]. Optics Express, 2007, 15(10): 6414-6419.
[22] Lin T G, Zhou Y Q, Yuan Y D, et al. Transflective spin-orbital angular momentum conversion device by three-dimensional multilayer liquid crystalline materials[J]. Optics Express, 2018, 26(22): 29244-29252.
[23] Rafayelyan M, Brasselet E. Spin-to-orbital angular momentum mapping of polychromatic light[J]. Physical Review Letters, 2018, 120(21): 213903.
[24] Wang L, Gutierrez-Cuevas K G, Bisoyi H K, et al. NIR light-directing self-organized 3D photonic superstructures loaded with anisotropic plasmonic hybrid nanorods[J]. Chemical Communications, 2015, 51(81): 15039-15042.
[25] Ma L L, Hu W, Zheng Z G, et al. Light-activated liquid crystalline hierarchical architecture toward photonics[J]. Advanced Optical Materials, 2019, 7(16): 1900393.
[26] Kim Y H, Yoon D K, Jeong H S, et al. Smectic liquid crystal defects for self-assembling of building blocks and their lithographic applications[J]. Advanced Functional Materials, 2011, 21(4): 610-627.
[27] Elston S J. Optics and nonlinear optics of liquid crystals[J]. Journal of Modern Optics, 1994, 41(7): 1517-1518.
[28] Berry M V. Quantal phase factors accompanying adiabatic changes[J]. Proceedings of the Royal Society of London A: Mathematical and Physical Sciences, 1984, 392(1802): 45-57.
[29] Bliokh K Y, Gorodetski Y, Kleiner V, et al. Coriolis effect in optics: unified geometric phase and spin-hall effect[J]. Physical Review Letters, 2008, 101(3): 030404.
[30] Pancharatnam S. Generalised theory of interference, and its applications[J]. Proceedings of the Indian Academy of Sciences, 1957, 45(6): 402-411.
[31] Mauguin C. Sur les cristaux liquides de M. Lehmann[J]. Bulletin De La Société Française De Minéralogie, 1911, 34(3): 71-117.
[32] Urbach W, Boix M, Guyon E. Alignment of nematics and smectics on evaporated films[J]. Applied Physics Letters, 1974, 25(9): 479-481.
[33] Ichimura K. Photoalignment of liquid-crystal systems[J]. Chemical Reviews, 2000, 100(5): 1847-1874.
[34] Hasegawa M, Taira Y. Nematic homogeneous photo alignment by polyimide exposure to linearly polarized UV[J]. Journal of Photopolymer Science and Technology, 1995, 8(2): 241-248.
[35] Palto S P, Yudin S G, Germain C, et al. Photoinduced optical anisotropy in Langmuir blodgett films as a new method of creating bistable anchoring surfaces for liquid crystal orientation[J]. Journal De Physique II, 1995, 5(1): 133-142.
[36] De Sio L, Roberts D E, Liao Z, et al. Digital polarization holography advancing geometrical phase optics[J]. Optics Express, 2016, 24(16): 18297-18306.
[37] Kim J, Li Y M, Miskiewicz M N, et al. Fabrication of ideal geometric-phase holograms with arbitrary wavefronts[J]. Optica, 2015, 2(11): 958-964.
[38] Ji W, Lee C H, Chen P, et al. Meta-q-plate for complex beam shaping[J]. Scientific Reports, 2016, 6: 25528.
[39] Lin T G, Xie J, Zhou Y J, et al. Recent advances in photoalignment liquid crystal polarization gratings and their applications[J]. Crystals, 2021, 11(8): 900.
[40] Hu W, Srivastava A, Xu F, et al. Liquid crystal gratings based on alternate TN and PA photoalignment[J]. Optics Express, 2012, 20(5): 5384-5391.
[41] Guo Y B, Jiang M, Peng C H, et al. High-resolution and high-throughput plasmonic photopatterning of complex molecular orientations in liquid crystals[J]. Advanced Materials, 2016, 28(12): 2353-2358.
[42] Schadt M. Liquid crystal materials and liquid crystal displays[J]. Annual Review of Materials Science, 1997, 27(1): 305-379.
[43] Gray G W, Kelly S M. Liquid crystals for twisted nematic display devices[J]. Journal of Materials Chemistry, 1999, 9(9): 2037-2050.
[44] Gregory D A, Kirsch J C, Tam E C. Full complex modulation using liquid-crystal televisions[J]. Applied Optics, 1992, 31(2): 163-165.
[45] Kirsch J C, Gregory D A, Thie M W, et al. Modulation characteristics of the Epson liquid crystal television[J]. Optical Engineering, 1992, 31(5): 963-970.
[46] Anderson J C. Thin-film transistors[J]. Electronics and Power, 1969, 15(3): 90-93.
[47] Huang Y G, Liao E, Chen R, et al. Liquid-crystal-on-silicon for augmented reality displays[J]. Applied Sciences, 2018, 8(12): 2366.
[48] 廖燕平, 宋勇志, 邵喜斌. 薄膜晶体管液晶显示器显示原理与设计[M]. 北京: 电子工业出版社, 2016: 80-85.
LiaoY P, SongY Z, ShaoX B. Thin film transistor liquid crystal display[M]. Beijing: Publishing House of Electronics Industry, 2016: 80-85.
[49] Liang J Y, Kohn R N,, Becker M F, et al. High-precision laser beam shaping using a binary-amplitude spatial light modulator[J]. Applied Optics, 2010, 49(8): 1323-1330.
[50] Dorrah A H, Zamboni-Rached M, Mojahedi M. Experimental demonstration of tunable refractometer based on orbital angular momentum of longitudinally structured light[J]. Light: Science & Applications, 2018, 7: 40.
[51] Hu D, Zheng J, Liao E L, et al. Modulating both amplitude and phase in a single-spatial light modulator (SLM)[J]. Proceedings of SPIE, 2022, 12026: 1202608.
[52] Hellman B, Rodriguez J, Luo C, et al. Angular and spatial light modulation by single digital micromirror device for beam and pattern steering[J]. Proceedings of SPIE, 2020, 11294: 112940C.
[53] Ross W E, Psaltis D, Anderson R H. Two-dimensional magneto-optic spatial light modulator for signal processing[J]. Optical Engineering, 1983, 22(4): 485-490.
[54] Forbes A, Dudley A, McLaren M. Creation and detection of optical modes with spatial light modulators[J]. Advances in Optics and Photonics, 2016, 8(2): 200-227.
[55] Nishchal N K, Joseph J, Singh K. Optical phase encryption by phase contrast using electrically addressed spatial light modulator[J]. Optics Communications, 2003, 217(1/2/3/4/5/6): 117-122.
[56] Yang L, El-Tamer A, Hinze U, et al. Parallel direct laser writing of micro-optical and photonic structures using spatial light modulator[J]. Optics and Lasers in Engineering, 2015, 70: 26-32.
[57] Granqvist C G, Azens A, Hjelm A, et al. Recent advances in electrochromics for smart windows applications[J]. Solar Energy, 1998, 63(4): 199-216.
[58] Wang Y, Runnerstrom E L, Milliron D J. Switchable materials for smart windows[J]. Annual Review of Chemical and Biomolecular Engineering, 2016, 7: 283-304.
[59] Cupelli D, Pasquale Nicoletta F, Manfredi S, et al. Self-adjusting smart windows based on polymer-dispersed liquid crystals[J]. Solar Energy Materials and Solar Cells, 2009, 93(11): 2008-2012.
[60] Wang L, Bisoyi H K, Zheng Z G, et al. Stimuli-directed self-organized chiral superstructures for adaptive windows enabled by mesogen-functionalized graphene[J]. Materials Today, 2017, 20(5): 230-237.
[61] Hu X W, Zhang X M, Yang W M, et al. Stable and scalable smart window based on polymer stabilized liquid crystals[J]. Journal of Applied Polymer Science, 2020, 137(30): 48917.
[62] Konforti N, Marom E, Wu S T. Phase-only modulation with twisted nematic liquid-crystal spatial light modulators[J]. Optics Letters, 1988, 13(3): 251-253.
[63] Li S Q, Xu X W, Veetil R M, et al. Phase-only transmissive spatial light modulator based on tunable dielectric metasurface[J]. Science, 2019, 364(6445): 1087-1090.
[64] Zuo Y, Zhao Y J, Chen Y C, et al. Scalability of all-optical neural networks based on spatial light modulators[J]. Physical Review Applied, 2021, 15(5): 054034.
[65] Li J X, Guan Z Q, Liu H C, et al. Metasurface-assisted indirect-observation cryptographic system[J]. Laser & Photonics Reviews, 2023, 17(1): 2200342.
[66] Fang X Y, Ren H R, Gu M. Orbital angular momentum holography for high-security encryption[J]. Nature Photonics, 2020, 14(2): 102-108.
[67] Hou J F, Situ G H. Image encryption using spatial nonlinear optics[J]. eLight, 2022, 2(1): 1-10.
[68] Duan W, Chen P, Wei B Y, et al. Fast-response and high-efficiency optical switch based on dual-frequency liquid crystal polarization grating[J]. Optical Materials Express, 2016, 6(2): 597-602.
[69] Lin Y H, Wang Y J, Reshetnyak V. Liquid crystal lenses with tunable focal length[J]. Liquid Crystals Reviews, 2017, 5(2): 111-143.
[70] Zhou Y J, Yuan Y D, Zeng T B, et al. Liquid crystal bifocal lens with adjustable intensities through polarization controls[J]. Optics Letters, 2020, 45(20): 5716-5719.
[71] Kobashi J, Yoshida H, Ozaki M. Planar optics with patterned chiral liquid crystals[J]. Nature Photonics, 2016, 10(6): 389-392.
[72] Chen P, Ma L L, Hu W, et al. Chirality invertible superstructure mediated active planar optics[J]. Nature Communications, 2019, 10: 2518.
[73] Li C X, Yang X F, Han J L, et al. Signal transmission encryption based on dye-doped chiral liquid crystals via tunable and efficient circularly polarized luminescence[J]. Materials Advances, 2021, 2(12): 3851-3855.
[74] Huang X J, Zhu D, Zhou Z, et al. Tiger Amulet inspired high-security holographic encryption via liquid crystals[J]. Nanophotonics, 2023, 12(9): 1787-1795.
[75] Chen K X, Xu C T, Zhou Z, et al. Multifunctional liquid crystal device for grayscale pattern display and holography with tunable spectral-response[J]. Laser & Photonics Reviews, 2022, 16(3): 2100591.
[79] Tang D L, Shao Z L, Zhou Y J, et al. Simultaneous surface display and holography enabled by flat liquid crystal elements[J]. Laser & Photonics Reviews, 2022, 16(7): 2100491.
[80] Xie X, Du W J, Shao Z L, et al. Multichannel binary-image and holographic display based on planar liquid crystal devices[J]. Laser & Photonics Reviews, 2023: 2300193.
[81] Luo X G. Principles of electromagnetic waves in metasurfaces[J]. Science China Physics, Mechanics & Astronomy, 2015, 58(9): 594201.
[83] Yu N F, Genevet P, Kats M A, et al. Light propagation with phase discontinuities: generalized laws of reflection and refraction[J]. Science, 2011, 334(6054): 333-337.
[84] Huang L L, Chen X Z, Mühlenbernd H, et al. Three-dimensional optical holography using a plasmonic metasurface[J]. Nature Communications, 2013, 4: 2808.
[85] Huang L L, Chen X Z, Mühlenbernd H, et al. Dispersionless phase discontinuities for controlling light propagation[J]. Nano Letters, 2012, 12(11): 5750-5755.
[86] Khorasaninejad M, Chen W T, Devlin R, et al. Metalenses at visible wavelengths: diffraction-limited focusing and subwavelength resolution imaging[J]. Science, 2016, 352(6290): 1190-1194.
[87] Wang S M, Wu P C, Su V C, et al. Broadband achromatic optical metasurface devices[J]. Nature Communications, 2017, 8: 187.
[88] Huo P C, Zhang C, Zhu W Q, et al. Photonic spin-multiplexing metasurface for switchable spiral phase contrast imaging[J]. Nano Letters, 2020, 20(4): 2791-2798.
[89] Chen J W, Wang K, Long H A, et al. Tungsten disulfide-gold nanohole hybrid metasurfaces for nonlinear metalenses in the visible region[J]. Nano Letters, 2018, 18(2): 1344-1350.
[90] Li K, Wang J W, Cai W F, et al. Electrically switchable, polarization-sensitive encryption based on aluminum nanoaperture arrays integrated with polymer-dispersed liquid crystals[J]. Nano Letters, 2021, 21(17): 7183-7190.
[91] Zhu S Q, Xu Z T, Zhang H, et al. Liquid crystal integrated metadevice for reconfigurable hologram displays and optical encryption[J]. Optics Express, 2021, 29(6): 9553-9564.
[92] Hu Y Q, Ou X N, Zeng T B, et al. Electrically tunable multifunctional polarization-dependent metasurfaces integrated with liquid crystals in the visible region[J]. Nano Letters, 2021, 21(11): 4554-4562.
[93] Wang J W, Li K, He H L, et al. Metasurface-enabled high-resolution liquid-crystal alignment for display and modulator applications[J]. Laser & Photonics Reviews, 2022, 16(1): 2100396.
[94] Mysliwiec J, Szukalska A, Szukalski A, et al. Liquid crystal lasers: the last decade and the future[J]. Nanophotonics, 2021, 10(9): 2309-2346.
[95] Cho S, Yoshida H, Ozaki M. Emission direction-tunable liquid crystal laser[J]. Advanced Optical Materials, 2020, 8(16): 2000375.
[96] Wang L, Urbas A M, Li Q. Nature-inspired emerging chiral liquid crystal nanostructures: from molecular self-assembly to DNA mesophase and nanocolloids[J]. Advanced Materials, 2020, 32(41): 1801335.
[97] Muszyński M, Król M, Rechcińska K, et al. Realizing persistent-spin-helix lasing in the regime of rashba-dresselhaus spin-orbit coupling in a dye-filled liquid-crystal optical microcavity[J]. Physical Review Applied, 2022, 17(1): 014041.
[98] Chen Y J, Zheng C L, Yang W J, et al. Over 200 ℃ broad-temperature lasers reconstructed from a blue-phase polymer scaffold[J]. Advanced Materials, 2022, 34(47): 2206580.
[99] Allen L, Beijersbergen M W, Spreeuw R J C, et al. Orbital angular momentum of light and the transformation of Laguerre-Gaussian laser modes[J]. Physical Review A, 1992, 45(11): 8185-8189.
[100] Shen Y J, Wang X J, Xie Z W, et al. Optical vortices 30 years on: OAM manipulation from topological charge to multiple singularities[J]. Light: Science & Applications, 2019, 8: 90.
[102] Rosales-Guzmán C, Bhebhe N, Forbes A. Simultaneous generation of multiple vector beams on a single SLM[J]. Optics Express, 2017, 25(21): 25697-25706.
[103] Arbabi A, Horie Y, Bagheri M, et al. Dielectric metasurfaces for complete control of phase and polarization with subwavelength spatial resolution and high transmission[J]. Nature Nanotechnology, 2015, 10(11): 937-943.
[104] Wang E L, Shi L N, Niu J B, et al. Multichannel spatially nonhomogeneous focused vector vortex beams for quantum experiments[J]. Advanced Optical Materials, 2019, 7(8): 1801415.
[105] Zhang Y H, Chen P, Ge S J, et al. Spin-controlled massive channels of hybrid-order Poincaré sphere beams[J]. Applied Physics Letters, 2020, 117(8): 081101.
[106] Xu C T, Chen P, Zhang Y H, et al. Tunable band-pass optical vortex processor enabled by wash-out-refill chiral superstructures[J]. Applied Physics Letters, 2021, 118(15): 151102.
[107] Chen P, Ge S J, Duan W, et al. Digitalized geometric phases for parallel optical spin and orbital angular momentum encoding[J]. ACS Photonics, 2017, 4(6): 1333-1338.
[108] Chen X, Korblova E, Dong D P, et al. First-principles experimental demonstration of ferroelectricity in a thermotropic nematic liquid crystal: polar domains and striking electro-optics[J]. Proceedings of the National Academy of Sciences of the United States of America, 2020, 117(25): 14021-14031.
[109] Mertelj A, Cmok L, Sebastián N, et al. Splay nematic phase[J]. Physical Review X, 2018, 8(4): 041025.
[110] Li J X, Nishikawa H, Kougo J, et al. Development of ferroelectric nematic fluids with giant-ε dielectricity and nonlinear optical properties[J]. Science Advances, 2021, 7(17): eabf5047.
[111] Zhao X H, Zhou J C, Li J X, et al. Spontaneous helielectric nematic liquid crystals: electric analog to helimagnets[J]. Proceedings of the National Academy of Sciences of the United States of America, 2021, 118(42): 1-9.
[112] Folcia C L, Ortega J, Vidal R, et al. The ferroelectric nematic phase: an optimum liquid crystal candidate for nonlinear optics[J]. Liquid Crystals, 2022, 49(6): 899-906.
[113] Zhao X H, Long H Q, Xu H, et al. Nontrivial phase matching in helielectric polarization helices: universal phase matching theory, validation, and electric switching[J]. Proceedings of the National Academy of Sciences of the United States of America, 2022, 119(29): 1-8.
[114] Liu S J, Chen P, Ge S J, et al. 3D engineering of orbital angular momentum beams via liquid-crystal geometric phase[J]. Laser & Photonics Reviews, 2022, 16(6): 2200118.
[115] Hu H L, Liu B H, Li M Q, et al. A quadri-dimensional manipulable laser with an intrinsic chiral photoswitch[J]. Advanced Materials, 2022, 34(15): 2110170.
Article Outline
朱宸, 邵正龙, 周英杰, 任佳琪, 樊帆, 汤东亮. 液晶的多维度光场调控技术研究进展[J]. 中国激光, 2023, 50(18): 1813006. Chen Zhu, Zhenglong Shao, Yingjie Zhou, Jiaqi Ren, Fan Fan, Dongliang Tang. Advances in Multi-Dimensional Light Field Modulation Based on Liquid Crystal[J]. Chinese Journal of Lasers, 2023, 50(18): 1813006.