基于反射点源的光学遥感卫星像点提取及精度验证
The automation of calibration and the accuracy of pixel extraction are the key factors in improving the efficiency and accuracy of geometric calibration of optical remote sensing satellites in orbit. With the increasing number of optical remote sensing satellites in orbit and the continuous improvement of satellite image resolution, current level of calibration automation cannot meet future development needs. The coordinates of control points and their corresponding image points are input parameters for the geometric calibration of the camera. The accuracy of their measurement and extraction directly affects the accuracy and precision of the internal and external orientation element calibration of the satellite camera. The measurement accuracy of ground control points and on-orbit attitude has reached centimeter or even millimeter-level precision. However, the pixel extraction of control point images mainly relies on manual selection or pixel measurement algorithms, which cannot match the accuracy of control point measurements. Lightweight and automated quasi-stellar source-reflected point sources are proposed to be used as ground control points, along with an algorithm for matching pixel extraction from these sources. This will be combined with on-orbit calibration experiments based on reflected point sources to verify the accuracy of pixel extraction for optical remote sensing satellites. The objective of this paper is to improve the accuracy of pixel extraction, which in turn will enhance the geometric calibration and remote sensing image positioning accuracy of these satellites in orbit.
First, we propose a Gaussian model-based method for pixel extraction from reflected point sources and a rational function model-based method for verifying the accuracy of pixel extraction. Based on these principles, we design an experiment to verify the accuracy of pixel extraction from reflected point sources. Second, we analyse the experimental image and extract the pixels by the reflected point source method. The accuracy of the extracted pixels is then verified by template matching and nonlinear optimization methods. Finally, we verify the accuracy of pixel extraction by the reflected point source method with a rational function model and calculate the accuracy of pixel positioning by the template matching method and nonlinear optimization method. A comparative verification of the reflected point source method is conducted.
The pixel coordinates extracted by the reflected point source method, template matching method, and nonlinear optimization method are shown in Tables 1 and 4. The results of the three methods are relatively consistent. The differences between the extracted pixel coordinates and the mean values are shown in Table 5. The maximum error is 0.0472 pixel, which is better than 0.05 pixel, which verify the accuracy of the reflected point source method for the optical remote sensing satellite. The pixel extraction accuracy is better than that of square-shaped top-hat markers and manual extraction of natural features. The validation results of the rational function model are shown in Table 7. After the maximum value is removed, the positioning accuracy of the extracted pixel coordinates using the reflected point source method, template matching method, and nonlinear optimization method in the flight direction and sensor line array direction is better than 0.04 pixel, and the planar accuracy is better than 0.05 pixel, further verify the accuracy of pixel extraction using the reflected point source method. The experimental results preliminarily indicate that the reflected point source method can achieve high-precision remote sensing image pixel extraction and meet the application requirements of high-precision targets for the geometric calibration of optical remote sensing satellites.
The preliminary results of on-orbit tests based on reflected point sources for optical remote sensing satellites indicate that compared to conventional methods such as template matching, the reflected point source method has an extraction accuracy of better than 0.05 pixel, which is higher than that for natural objects and artificial targets. The rational function model parameters accompanying the remote sensing images are applied to verify the positioning accuracy of reflected point source method, which is better than 0.05 pixel. Automated reflected point source method not only enable high-precision pixel extraction but also combine the array configuration of point sources with the automatic measurement of atmospheric optical characteristics in the test area. Image quality evaluation and radiation calibration are achieved for optical remote sensing satellites. This can improve the efficiency and accuracy of on-orbit calibration for optical remote sensing satellites. Automated reflected point sources are expected to be networked and deployed within the field of view of optical remote sensing satellites, forming ground control points for on-orbit geometric calibration and enabling high-precision, high-frequency, and normalized geometric radiation calibration for optical remote sensing satellites. Our study provides a reference for automated calibration and accuracy improvement of domestic remote sensing satellites and is of great significance for improving the quantitative level and application of optical remote sensing satellite images.
1 引言
光学遥感卫星作为全球空间信息获取的重要工具,在自然资源调查和监测、交通道路规划、基础测绘等领域发挥着关键作用,对推动经济发展有重要意义[1]。然而,卫星发射和在轨运行期间会受到空间环境和各种干扰力的影响,导致卫星成像系统的实验室检校参数发生变化,从而增大成像模型定位误差,影响光学遥感卫星产品定量化应用。因此,定期或不定期对光学遥感卫星进行在轨几何定标、获取高精度的内外方位元素,成为决定卫星影像定位精度的重要因素[2-3]。
光学遥感卫星在轨几何定标主要涉及地面控制点、卫星姿轨数据及控制点影像像点等数据源。具有良好几何特征的自然地物或人工靶标作为地面控制点为卫星相机提供了稳定的参考坐标和校准数据,在卫星影像的校正和定量化应用中起到了重要作用。控制点及其像点坐标作为相机几何定标的输入参数,其测量和提取精度直接影响到卫星相机内外方位元素检校的准确性和精度。当前,地面控制点和星上位姿的测量精度已达到cm甚至mm级别,但是控制点影像的像点提取主要依赖人工选点或像点测量算法。人工选点依靠主观判断,误差可达0.5~1.0 pixel[4-5];测量算法一般误差也在0.3 pixel[6-7]。航空遥感制作的高精度数字正射模型(DOM)和数字高程模型(DEM)利用影像匹配算法可以获取大量的控制点和像点坐标,但是航空影像和卫星影像存在异源与时差等问题,使得控制点影像像点提取精度无法与地面测量精度相匹配[8]。恒星作为典型的点源目标,几何形态好且星图像点提取精度高,理论上可以实现快速自动的几何定标,但是对卫星平台的机动能力要求较高,目前尚未实际应用[9-11]。因此,随着在轨运行光学遥感卫星数量的不断增加和卫星影像分辨率的不断提升,在轨几何定标的自动化和控制点影像像点提取的精度提升对于提高卫星相机参数检校与影像定量化应用来说具有重要意义。
本文提出以轻小型、自动化的类恒星源-反射点源作为地面控制点,匹配点源影像像点提取算法,结合基于反射点源的光学遥感卫星在轨定标实验与像点提取精度验证,以期提高光学遥感卫星像点提取精度,进而提高光学遥感卫星在轨几何定标精度与遥感影像定位精度。
2 基本原理
2.1 像点提取
反射点源目标能够将入射的太阳光线反射至卫星相机,从而形成点激冲辐射。经过光学追迹与模拟分析,相机接收的反射光斑仅为反射点源镜面上cm甚至mm量级的小区域,具有相对于背景环境高得多的亮度、相对于相机分辨率小得多的尺寸,可以作为类恒星源目标[12]。由光学原理与傅里叶光学可知,光学遥感卫星成像系统可作为线性位移不变系统,因此以反射点源作为输入的物像关系可表示为
式中:
恒星作为典型的点源目标,在星相机获取星点影像后,通常以高斯函数模拟成像系统的点扩散函数,从而还原目标真实情况或者进行目标中心定位[13-14]。反射点源作为类恒星源,其成像特性和恒星一致。结合地面点源成像实验,可采用近似高斯模型来表征光学遥感卫星成像系统的点扩散特性,具体表示为
式中:
式中:
式中,
根据光学遥感卫星点源成像实验与仿真分析,在传感器点扩散函数的作用下,反射点源影像一般可扩散至3~7 pixel之间。选取反射点源遥感影像中心5 pixel×5 pixel范围内的响应值进行参数解算,可列写25个方程,并根据最小二乘平差原理求解参数的改正数
2.2 精度验证
利用有理函数模型(RFM)进行反射点源像点提取精度验证,其在数学意义上是对严格成像模型(RPM)的最佳拟合[15]。RFM综合考虑了多个因素对结果的影响,以综合性建模的方式实现了准确的目标定位,其结构形式简单,便于使用,具体定义为
式中:
式中:
经传感器校正影像产品生成的RFM模型系数直接定位误差较大,但是误差呈现出一定的系统性[16-17]。研究发现,在RFM的像方空间附加仿射变换模型对定位误差的补偿效果最好[18-19],因此采用此方式对反射点源像点提取精度展开验证。具体表示为
式中:
利用平差后的模型对影像上测量的像点展开验证,即
式中:
3 实验数据处理
光学遥感卫星基于反射点源目标的像点提取及精度验证实验如
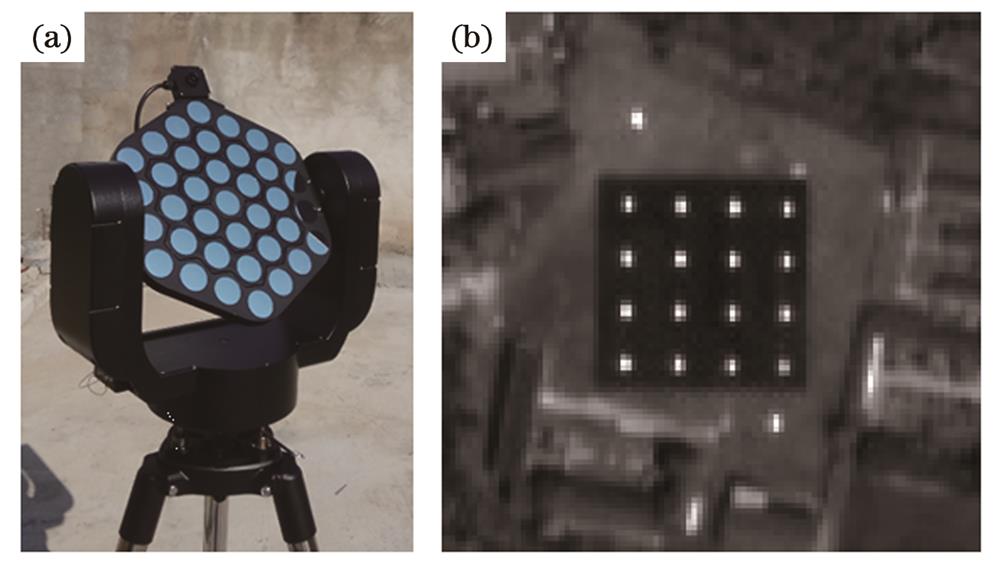
图 1. 在轨验证实验。(a)反射点源;(b)反射点源影像
Fig. 1. In orbit verification experiment. (a) Reflected point source; (b) image of reflected point source
反射点源由多个组件构成,包括反射镜组件、姿态调控组件、电控系统和太阳敏感器等。其中:反射镜组件的作用是将入射的太阳光线反射至卫星相机,形成点激冲辐射。通过调整反射镜的数量来调谐反射的能量,以适应不同分辨率光学遥感卫星相机的动态范围,使得遥感器响应高端且不饱和。电控系统根据卫星过顶预报参数,通过姿态调控组件调整反射镜组件姿态,实现卫星过顶成像时的光路对准,从而将太阳光反射至卫星相机。利用太阳敏感器进行太阳运动轨迹的连续跟踪观测,标定反射点源目标的系统误差及空间指向(精度优于0.1°)。
表 1. 反射点源像点坐标
Table 1. Pixel coordinates of reflected point source image
|
表 2. 平差后的定位坐标
Table 2. Positioning coordinates after adjustment
|
表 3. 点源法像点提取精度验证
Table 3. Verification of the pixel extraction accuracy using the point source method
|
通过分析反射点源目标的遥感影像可知,点源反射的太阳光束在光学遥感卫星相机成像系统点扩散函数作用下,集中分布在3 pixel×3 pixel范围内,如
光学遥感卫星影像经传感器校正,并附带有RFM参数文件。根据反射点源法像点提取原理拟合得到点源像点坐标和有理函数模型的像方投影坐标,对仿射变换模型参数进行平差,然后将仿射变换模型附加在有理函数模型的像方坐标上以获取平差后的像方坐标,结果如
4 分析与讨论
在光学遥感卫星反射点源像点提取方法及精度验证中,以“小”而“亮”、类恒星的自动化反射点源目标作为地面控制点,匹配模型拟合算法获取高精度的像点坐标,进而以有理函数模型验证点源像点提取精度,不仅要考虑反射点源结构形式与影像特征,还要对像点提取算法准确性等进行分析讨论。
反射点源反射至卫星入瞳的光斑、辐射亮度远高于地面背景,且空间尺度相对卫星相机分辨率小得多。相对于SPOT卫星的主动式点源(聚光灯),反射点源不需要大功率电源供电以及光谱非一致性校正[20];相对于IKONOS等卫星对深空恒星成像,反射点源不需要卫星平台翻转或姿态调整[21]。反射点源目标的数量和反射辐射亮度自主可控,且其影像信噪比较高,优于一般恒星影像;此外,其能够在光学遥感卫星对地观测状态下进行在轨几何定标,不存在恒星定标中光谱非一致问题,且具有较好的几何形态,可以获取高精度的地面坐标。采用模板匹配法与非线性优化求解法对反射点源法提取的像点坐标
表 4. 多种方式像点提取结果
Table 4. Pixel extraction results by multiple methods
|
表 5. 像点坐标误差
Table 5. Errors of pixel coordinate
|
表 6. 常规方法平差后的定位坐标
Table 6. Positioning coordinates after conventional method adjustment
|
表 7. 多种像点提取方法精度验证
Table 7. Accuracy verification of multiple pixel extraction results
|
通过模板匹配法等常规方法验证了反射点源目标的像点提取精度优于0.05 pixel。进一步结合有理函数模型对反射点源像点提取结果进行精度验证。模板匹配法与非线性优化求解法平差后的定位结果如
在基于反射点源的光学遥感卫星像点提取精度验证实验中,结合反射点源布设阵列将阵列点源影像数据按照提取的像点坐标配准,可得到光学遥感卫星相机成像系统的点扩散轮廓,如
5 结论
光学遥感卫星反射点源法像点提取及精度验证以小型化、自动化的类恒星源-反射点源目标作为地面控制点,匹配像点提取算法,实现高精度的点源像点提取,并结合有理函数模型进行像点提取精度验证。基于反射点源的光学遥感卫星在轨实验结果初步表明:与模板匹配法等常规方法相比,反射点源法的点源像点提取精度优于0.05 pixel,相对自然地物与人工靶标具有较高的提取精度;利用遥感影像附带的有理函数模型参数验证了反射点源定位精度优于0.05 pixel;自动化反射点源不仅能够实现高精度的像点提取,与点源目标阵列化布设和实验场区大气光学特性参数自动化测量结合,还能够实现光学遥感卫星的像质评价与辐射定标,从而提高光学遥感卫星在轨定标的效率及精度。自动化反射点源有望在光学遥感卫星幅宽范围内网络化布设,形成卫星在轨几何定标的地面控制点,实现光学遥感卫星高精度、高频次、常态化几何辐射综合定标,为国产遥感卫星自动化定标与精度提升提供参考,对提升光学遥感卫星影像的定量化水平,拓展其应用具有重要意义。
[1] 王任享, 王建荣. 我国卫星摄影测量发展及其进步[J]. 测绘学报, 2022, 51(6): 804-810.
Wang R X, Wang J R. Development and progress of satellite photogrammetry in China[J]. Acta Geodaetica et Cartographica Sinica, 2022, 51(6): 804-810.
[2] 李晶, 王蓉, 朱雷鸣, 等. “天绘一号”卫星测绘相机在轨几何定标[J]. 遥感学报, 2012, 16(S1): 35-39.
Li J, Wang R, Zhu L M, et al. In-flight geometric calibration for Mapping Satellite-1 surveying and mapping camera[J]. Journal of Remote Sensing, 2012, 16(S1): 35-39.
[3] GrodeckiJ, LutesJ. IKONOS geometric calibrations[C].∥Proceedings of the ASPRS 2005 Annual Conference. Baltimore, Maryland: Springer, 2005.
[4] Cao J S, Yuan X X, Gong J Y. In-orbit geometric calibration and validation of ZY-3 three-line cameras based on CCD-detector look angles[J]. The Photogrammetric Record, 2015, 30(150): 211-226.
[5] 刘楚斌. 高分辨率遥感卫星在轨几何定标关键技术研究[D]. 郑州: 解放军信息工程大学, 2012.
LiuC B. Research on key technologies of geometric calibration of high resolution remote sensing satellite in orbit[D]. Zhengzhou: PLA Information Engineering University, 2012.
[6] 李秀举. 高分辨率遥感卫星在轨几何定标方法研究[D]. 合肥: 中国科学院大学合肥物质科学研究院, 2013.
LiX J. Research on methods for on-orbit geometric calibration of high resolution remote sensing satellite[D]. Hefei: Hefei Institute of Physical Science, Chinese Academy of Sciences, 2013.
[7] 李少峰, 张建霞, 刘保还. 数字摄影测量像点量测精度检测方法研究[J]. 测绘技术装备, 2014, 16(3): 25-28.
Li S F, Zhang J X, Liu B H. Research on measurement accuracy detection method of digital photogrammetry image point[J]. Geomatics Technology and Equipment, 2014, 16(3): 25-28.
[8] 潘红播, 张过, 唐新明, 等. 资源三号测绘卫星影像产品精度分析与验证[J]. 测绘学报, 2013, 42(5): 738-744.
Pan H B, Zhang G, Tang X M, et al. Accuracy analysis and verification of ZY-3 products[J]. Acta Geodaetica et Cartographica Sinica, 2013, 42(5): 738-744.
[9] 徐彬, 郑勇, 陈张雷, 等. 星光导航星点目标区域提取算法改进[J]. 测绘学报, 2023, 52(5): 760-768.
Xu B, Zheng Y, Chen Z L, et al. The improvement of star target region extraction algorithm for star centroid[J]. Acta Geodaetica et Cartographica Sinica, 2023, 52(5): 760-768.
[10] 李凯. 遥感传感器在轨几何定标与自动处理技术研究[D]. 郑州: 战略支援部队信息工程大学, 2019.
LiK. Research on on-orbit geometric calibration and automatic processing technology of remote sensing sensor[D]. Zhengzhou: Information Engineering University, 2019.
[11] 王密, 田原, 程宇峰. 高分辨率光学遥感卫星在轨几何定标现状与展望[J]. 武汉大学学报(信息科学版), 2017, 42(11): 1580-1588.
Wang M, Tian Y, Cheng Y F. Development of on-orbit geometric calibration for high resolution optical remote sensing satellite[J]. Geomatics and Information Science of Wuhan University, 2017, 42(11): 1580-1588.
[12] 徐伟伟, 张黎明, 李鑫, 等. 高分辨率光学遥感卫星反射点源像点提取方法[J]. 测绘学报, 2020, 49(10): 1295-1302.
Xu W W, Zhang L M, Li X, et al. Pixel extraction of reflected point source image for high spatial resolution optical remote sensing satellite[J]. Acta Geodaetica et Cartographica Sinica, 2020, 49(10): 1295-1302.
[13] 陈怡羽, 郭强. 中短波红外恒星定标点扩散函数求解及精度分析[J]. 遥感技术与应用, 2017, 32(1): 158-165.
Chen Y Y, Guo Q. A precise way to estimate PSF and analyze its accuracy in medium-wave infrared calibration with star-sensing[J]. Remote Sensing Technology and Application, 2017, 32(1): 158-165.
[14] 王敏, 赵金宇, 陈涛. 基于各向异性高斯曲面拟合的星点质心提取算法[J]. 光学学报, 2017, 37(5): 0515006.
[15] MadaniM. Real-time sensor-independent positioning by rational functions[C]∥ISPRS Workshop on Direct Versus Indirect Methods of Sensor Orientation, Barcelona: 1999.
[16] 唐新明, 张过, 祝小勇, 等. 资源三号测绘卫星三线阵成像几何模型构建与精度初步验证[J]. 测绘学报, 2012, 41(2): 191-198.
Tang X M, Zhang G, Zhu X Y, et al. Triple linear-array imaging geometry model of Ziyuan-3 surveying satellite and its validation[J]. Acta Geodaetica et Cartographica Sinica, 2012, 41(2): 191-198.
[17] Fraser C S, Hanley H B. Bias compensation in rational functions for IKONOS satellite imagery[J]. Photogrammetric Engineering & Remote Sensing, 2003, 69(1): 53-57.
[18] 李德仁, 童庆禧, 李荣兴, 等. 高分辨率对地观测的若干前沿科学问题[J]. 中国科学: 地球科学, 2012, 42(6): 805-813.
Li D R, Tong Q X, Li R X, et al. Some frontier scientific problems of high-resolution earth observation[J]. Scientia Sinica: Terrae, 2012, 42(6): 805-813.
[19] 宋美杰, 李浩. 资源三号卫星影像在定位方面的问题研究[J]. 地理空间信息, 2021, 19(11): 26-30.
Song M J, Li H. Research on the positioning problem of ZY-3 satellite images[J]. Geospatial Information, 2021, 19(11): 26-30.
[20] Leger D, Viallefont F, Hillairet E, et al. In-flight refocusing and MTF assessment of SPOT5 HRG and HRS cameras[J]. Proceedings of SPIE, 2003, 4881: 224-231.
[21] Dial G, Bowen H, Gerlach F, et al. IKONOS satellite, imagery, and products[J]. Remote sensing of Environment, 2003, 88(1-2): 23-36.
[22] 司孝龙, 张黎明, 付兴科, 等. 基于地面人工标志的卫星在轨几何定标方法研究[J]. 大气与环境光学学报, 2014, 9(2): 149-158.
[23] 徐伟伟, 张黎明, 杨宝云, 等. 光学卫星相机在轨调制传递函数检测方法[J]. 光学学报, 2020, 40(22): 2228001.
[24] 徐伟伟, 张黎明, 陈洪耀, 等. 基于反射点源的高分辨率光学卫星传感器在轨辐射定标方法[J]. 光学学报, 2017, 37(3): 0328001.
[25] 郑昱君, 徐伟伟, 李鑫, 等. 基于阵列点源的光学遥感卫星像质评价方法[J]. 光子学报, 2023, 52(4): 0428001.
王强, 徐伟伟, 司孝龙, 李鑫, 杨宝云, 张黎明. 基于反射点源的光学遥感卫星像点提取及精度验证[J]. 光学学报, 2024, 44(6): 0628004. Qiang Wang, Weiwei Xu, Xiaolong Si, Xin Li, Baoyun Yang, Liming Zhang. Pixel Extraction and Accuracy Verification of Optical Remote Sensing Satellite Based on Reflected Point Source[J]. Acta Optica Sinica, 2024, 44(6): 0628004.