锆离子掺杂对掺镱SiO2微球自激拉曼激光的增强
下载: 777次
Objective Stimulated Raman scattering is an important method to extend the wavelength of the laser. The self-stimulated Raman laser is a phenomenon of the pump wavelength to excite the ions of the rare-earth-doped laser gain media and generate the fundamental mode, single-frequency laser, which then generates the stimulated Raman scattering laser. The self-stimulated Raman laser reduces the optical elements in the cavity, yielding a compact laser to reduce the optical loss, and improve the performance and efficiency of the laser. Due to the high-quality factor (Q, up to 10 9) of the whispering gallery mode (WGM), the silica microcavity has been widely used in the study of nonlinear optical phenomena. The first-order self-Raman laser for Nd 3+ doped SiO2 microspheres with high Q-value has been reported. To enhance the self-Raman laser, the silica microspheres were coated with Yb 3+-Zr 4+ doped silica film using the sol-gel and immersion method. The 976 nm pump laser was coupled with a tapered optical fiber to form a highpower density WGM in the inner surface of the microspheres. The results provide a reference for the preparation of materials with a high efficiency self-stimulated Raman laser, allowing the laser to operate at 1.2--1.3 μm wavelength band which can be used in the second near-infrared window.
Methods After removing the coating layer of the standard single mode fiber, the single mode fiber was heated using an alcohol lamp to form the tapered fiber. The end of the tapered fiber was fused with the electrode discharge arc and formed into SiO2 microspheres with handles for operation. The Yb 3+Zr 4+ doped silica was prepared by the sol-gel method with the Yb 3+ concentration of 4%, and the doping concentrations of Zr 4+ were 0%, 5%, 10%, and 15%. The optimal doping concentration of Zr 4+ was 10%. The sol-gel film was deposited on the surface of SiO2 microspheres via the immersion-lifting method. After drying, the film was melted using the electrode discharge arc to improve the Q-value of the coated microspheres. A 976 nm semiconductor laser was used as the pump source. Next, the pump light was coupled into the inner surface of the microsphere equator to form WGM by the tapered optical fiber. The coupling position between the tapered optical fiber and the microsphere was controlled by a three-dimensional adjusting frame and monitored by a charge-coupled device microscope. The output end of the tapered fiber was connected to an optical spectral analyzer (OSA, 600--1700 nm) to measure the transmission spectrum of the microspheres (Fig. 1). The basic frequency laser and self-Raman laser of Yb 3+-doped and Yb 3+-Zr 4+ co-doped microspheres were measured using this setup by gradually increasing the pump power.
Results and Discussions The Yb 3+-doped microsphere and Yb 3+-Zr 4+ co-doped microsphere have the same diameter of 68 μm with the function film thickness of 0.5 μm. Under the 976 nm excited laser with the power of 18.5 dBm, the self-stimulated Raman scattering spectra can be observed. Three orders of the cascade self-stimulated Raman laser were obtained; the wavelength of the third-order self-Raman laser can reach up to 1295 nm (Fig. 4).Alternatively, the Yb 3+-doped microsphere obtained three cascade self-Raman lasers with the pump power of 24.3 dBm, which verified that Zr doping can enhance the self-stimulated Raman laser. Thus, the laser conversion efficiency and the threshold of the third-order self-Raman laser can be achieved (Fig. 6). The 10% doping concentration of Zr 4+ makes the third-order self-Raman laser threshold only 12% of the Yb-doped microsphere, and the conversion efficiency is 3.09 times of Yb 3+-doped, which attributes to the improvement of the high polarization of the silica bond by Zr 4+ doping, thereby increasing the phonon vibration and Raman gain coefficient of the silica. According to the results, the Raman gain coefficient of the microsphere material with and without Zr 4+doping is estimated and then compared; the results show that the Yb 3+ 4% mol/Zr 4+ 10% mol doped silica increases the Raman gain factor by 18.966 times compared to the Yb 3+4% mol doped silica.
Conclusions In this study, the phenomenon of the self-stimulated Raman laser enhancement is investigated using a high Q-value microsphere cavity coupled with a tapered optical fiber. The SiO2 microspheres were coated with Yb 3+ or Yb 3+/Zr 4+ doped SiO2 thin films via the sol-gel adhesive coating method. Theoretical analysis shows that the Zr 4+ ion doped films can increase the susceptibility of the rare-earth-doped gain media and increase the Raman gain factor as well as increase the film’s refractive index to result in gathering WGMs, thereby reducing the mode volume and increasing the power density of the WGM. Both effects can reduce the Raman laser threshold and improve the efficiency of the self-stimulated Raman laser. When compared with the Yb 3+-doped silica microsphere, the experimental results show that the silica microsphere with co-doping concentration of 4% mol Yb 3+/10 mol% Zr 4+increases the Raman gain coefficient by 19 times, the slope efficiency of the third-order self-stimulated Raman laser increases by 3.09 times, and the third-order self-stimulated wavelength extends to 1295 nm. The enhancement effect of the self-stimulated Raman laser of the Yb 3+ /Zr 4+ co-doped silica microspheres is consistent with the theoretical analysis.
1 引言
自激发拉曼激光是一种泵浦光激励增益介质,受激辐射产生基频激光,进而基频激光在介质传输,因受激散射而产生频移的拉曼激光的现象。受激拉曼散射是拓展激光波长的一种重要手段。高功率密度光源泵浦拉曼活性介质所产生的受激拉曼散射能产生一阶以上的级联的受激拉曼散射,使激光波长以介质的拉曼频移为间隔拓展,获得线宽较窄的新波长激光。固体拉曼活性介质有钒酸盐(如YVO4)[1]、钼酸盐(如PbMO4)[2]等晶体或玻璃材料[3-4]。当这些拉曼活性介质掺杂了稀土离子时,可作为受激辐射的增益材料,在强泵浦光激励下既可产生受激辐射的基频激光,又可以实现基频激光拉曼频移,即实现自激发拉曼激光输出,如Nd∶GdV
近年来,熔融液体表面张力形成的介质微腔由于具有很高的品质因子(可达109),光波在其中以倏逝场传播时形成一种具有小模式体积(约300λ3)、高能量密度(约1 GW/cm2)的回音壁模(WGM), 而被广泛用于基础物理与应用研究中[12],如稀土离子掺杂低阈值光学微腔激光器[13-14]、低阈值多级拉曼激光器[15-16]以及微腔传感器[17-18] 等领域。Vahala课题组[19]报道了采用硅片上的SiO2微芯环形腔,在泵浦波长1561 nm处获得了高达2.5×107的Q值,在泵浦功率640 μW的情况下获得1679 nm的拉曼激光;采用锥球耦合系统从理论和实验两方面研究了级联受激拉曼散射的阈值和效率、转换效率对Q值的依赖性和耦合情况对低阈值拉曼激光的影响[18,20];采用高Q值SiO2微球在980 nm附近获得五阶级联拉曼散射,最高延展至1231 nm[15-16]。
同时,对比稀土离子掺杂晶体的自激发拉曼激光现象,黄衍堂等[21-23]报道了采用锥光纤将 808 nm 的激光耦合入Nd3+离子掺杂的高Q值SiO2微球,激发产生了1080~1097 nm波段基频激光和波长为1120~1143 nm一级自受激拉曼激光。为增强拉曼激光,在微腔外层镀重金属敏化离子掺杂的SiO2膜层,如掺T
为增强自激发拉曼激光,本文通过溶胶凝胶法对二氧化硅微球腔镀Yb3+-Zr4+共掺杂功能薄膜,研究基于微球腔的自激发拉曼激光增强,本成果一方面产生了可用于第二生物窗口的1.2~1.3 μm波段激光,另一方面可为产生高效率自激发拉曼光的材料制备提供参考。
2 基本原理
2.1 材料的极化强度与拉曼增益系数
玻璃的三阶非线性光学性质在很大程度上取决于在玻璃中掺杂形成网格的阴阳离子键的极化率,并且与离子的掺杂浓度有着比较大的关联性[28]。已报道通过超瑞利光散射法计算了SiO2和ZrO2的极化率可以证实系统的极化率取决于粒径和浓度[29]。SiO2材质通常约在500 cm-1和1000 cm-1处显示出两个不同的拉曼响应峰[30],分别代表弯曲模式和拉伸模式的拉曼截面A500和A1000,拉曼增益系数与这二者之间的比值A500/A1000正相关。在SiO2溶胶中掺杂Zr元素会改变Si—O键的性质并影响O元素的电荷分布,从而影响弯曲模式和拉伸模式的拉曼截面,增大SiO2膜层的A500/A1000比值,导致极化强度和拉曼增益因子同时增大[25,30]。体材料的本征拉曼增益因子gR可以表示为[18,31-33]
式中:M为分子数密度;c是真空中的光速;下标S和P分别分示信号光和泵浦光;ωS和ωP分别是斯托克斯光和泵浦光的频率;nS是微球介质的折射率;
式中:Δω是频率增量。散射截面对立体角的偏微分定义为单位体积散射介质斯托克斯光光子通量与泵浦光光子通量的比值:
光子通量与电磁波振幅E之间的关系可表示为
式中:k为波矢量大小;h是常数;μ0为磁导率。由(3)、(4)式可得:
斯托克斯光与泵浦光满足三阶极化耦合方程:
将三阶极化率χ(3)分解为实部与虚部之和χ(3)=χR+iχI,当发生拉曼散射时,虚部χI恒小于零,且绝对值随极化率增加而增大,则斯托克斯光场可分解为相位调制项和强度变化项的乘积:
联立(1)、(2)、(5)、(9)式可得:
由(10)式可知,虽然折射率增大会减弱拉曼增益,但极化率增加能增大拉曼增益因子。
2.2 微腔拉曼阈值
微球腔的拉曼阈值Pth的定义[30]为
式中:β是与锥光纤与微球耦合模场参数相关的常数;neff是有效折射率;Vm是回廊模的模式体积;λp和λR分别是泵浦波长和拉曼波长。由(11)式可知,重金属氧化物掺杂增加neff使拉曼阈值增加,但能更大地增加gR,减小模式体积Vm能减小阈值功率。
利用溶胶凝胶方法[34]将单掺Yb3+和共掺Yb3+-Zr4+离子膜层粘涂在微球腔表面。镀Yb3+-Zr4+离子掺杂的膜层,一方面通过增加极化率使拉曼增益系数gR增大,另一方面掺Zr4+的SiO2膜层折射率neff增大,微球这层高折射率的膜层使大部分WGM光场约束在其中[35],改变了WGM模场分布方式,将光场向膜层中聚焦,减小光学模式体积[25],提高了WGM功率密度,二者都有利于降低拉曼激光阈值,有利于自激发拉曼激光的产生。
3 微球制备及实验装置与测试
3.1 微球功能膜层制备及实验装置
采用标准通信光纤去除涂覆层后在酒精灯火焰上加热成光纤锥,用电极放电电弧熔融光纤锥末端,烧制成带柄的SiO2微球备用。选用硝酸镱五水合物Yb(NO3)3·5H2O、Zr(NO3)4·5H2O分别作为Yb3+、Zr4+离子提供试剂。用溶胶凝胶法制备Yb3+-Zr4+掺杂的二氧化硅凝胶,采用浸入提拉法在SiO2微球表面镀溶胶-凝胶膜,风干后采用电极放电火花融熔膜层使之致密光滑,以提高镀膜微球腔的Q值。掺杂的Yb3+离子浓度(摩尔分数)固定为4%[23],作为对比Zr4+离子掺杂浓度分别为0%、5%、10%,实验证明三种掺杂浓度中,Zr4+离子掺杂浓度为10%(当硝酸锆提供的Zr4+离子大于10%时,凝胶容易固化)时具有最高的拉曼增益,因此将其与掺杂浓度0%的情况进行比较。
实验装置如
3.2 微球膜层折射率估测
将
式中:λυ、λυ+1是相邻的谐振波长;R是微球腔的半径。虽然折射率是与波长相关的参数,但在实验涉及的波长范围内,其变化量远小于Zr4+掺杂导致的折射率变化,因此在讨论中将其视为仅随掺杂浓度变化的参数,并以ASE透射光谱测得的ΔλFSR计算得到的折射率平均值作为该浓度下实验波长范围的折射率。
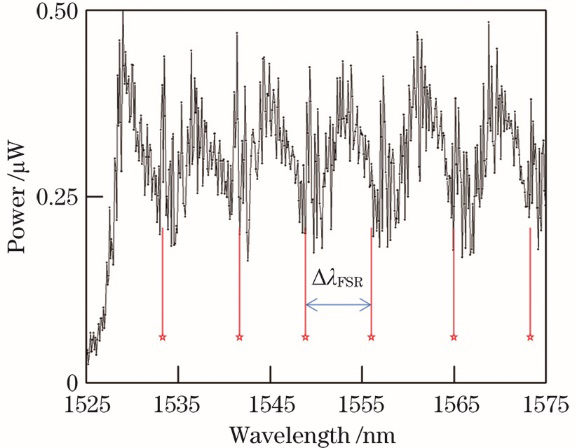
图 2. 以1550 nm ASE光源为泵浦源锥光纤耦合输出的微球谐振吸收谱
Fig. 2. Resonance absorption spectra of microspheres coupled out of tapered fiber with 1550 nm ASE light source as pump source
根据测量结果计算,可得单独掺杂Yb3+离子的微球膜层折射率约为1.482,而Yb3+-Zr4+共掺微球膜层折射率约为1.521。折射率的增加是由于ZrO2的折射率高于SiO2(nZrO2=2.208,nSiO2=1.454),外层折射率的增加将导致回廊模向空气穿透深度减少,使模式体积(Vm)减小。
3.3 微球腔品质因子测量
光学微球腔中的品质因子可以通过谐振峰的谱线宽度来计算,定义为谐振波长λυ与谐振谱线宽Δλυ的比值为Q=
Q值测量方法如
式中:f是微球谐振频率(对应微球的谐振波长λυ);Δf是谐振峰的半峰全宽;tFWHM为对应于共振吸收下降峰半峰全宽的时间;Usingle和Tsingle分别为函数发生器输出的三角波峰-峰电压和信号周期。
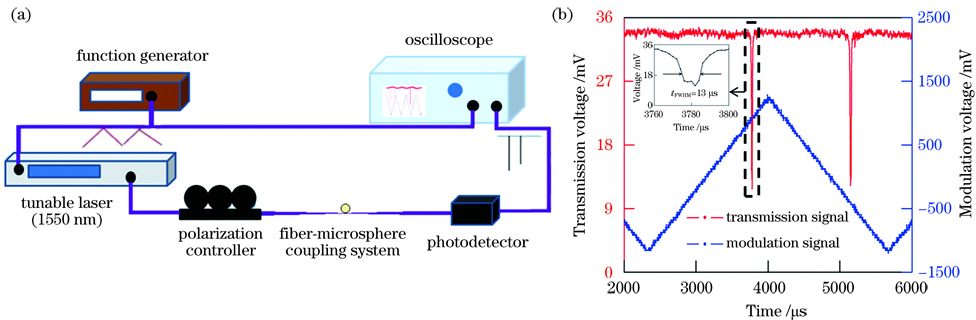
图 3. Q值测试图。(a)Q值测试装置示意图;(b)示波器显示图
Fig. 3. Schematic of Q factor testing. (a) Schematic of Q factor test setup; (b) oscilloscope display
将测量结果(对于单掺Yb3+微球腔,λυ=1521.541 nm,Usingle=2400 mV,Tsingle=3376 μs,tFWHM=9 μs;对于Yb3+-Zr4+共掺微球腔,λυ=1521.290 nm,Usingle=2480 mV,Tsingle=3360 μs,tFWHM=13 μs)代入计算,单掺Yb3+离子的微球腔Q值约为5.14×107,而Yb3+-Zr4+共掺微球腔Q值约为3.43×107。虽然掺入了Zr元素SiO2溶胶有着比通常更高的固有拉曼增益因子gR,但是Zr元素的掺杂会导致品质因子Q的下降,这是由于掺杂离子导致的材料吸收损耗增加。然而,微球腔的Q值仍超过107。对于制备好的锥与微球腔,通常将其用干燥盒保护,以避免灰尘和水分子附着在微球腔表面导致Q值下降。
4 联级自激发拉曼激光及结果分析
4.1 基频激光与三阶自激发拉曼激光
采用锥球耦合系统,逐渐增大泵浦功率,测试了Yb3+单掺与Yb3+-Zr4+共掺微球的基频激光与自激发拉曼激光。实验采用直径同为68 μm、膜层厚度约为0.5 μm的单掺Yb3+和Yb3+-Zr4+共掺微球。在相同的泵浦波长(976 nm)和不同(24.3 dBm)及相同(18.5 dBm)泵浦功率,可以观察到对照组的基频激光和自激发拉曼激光光谱如
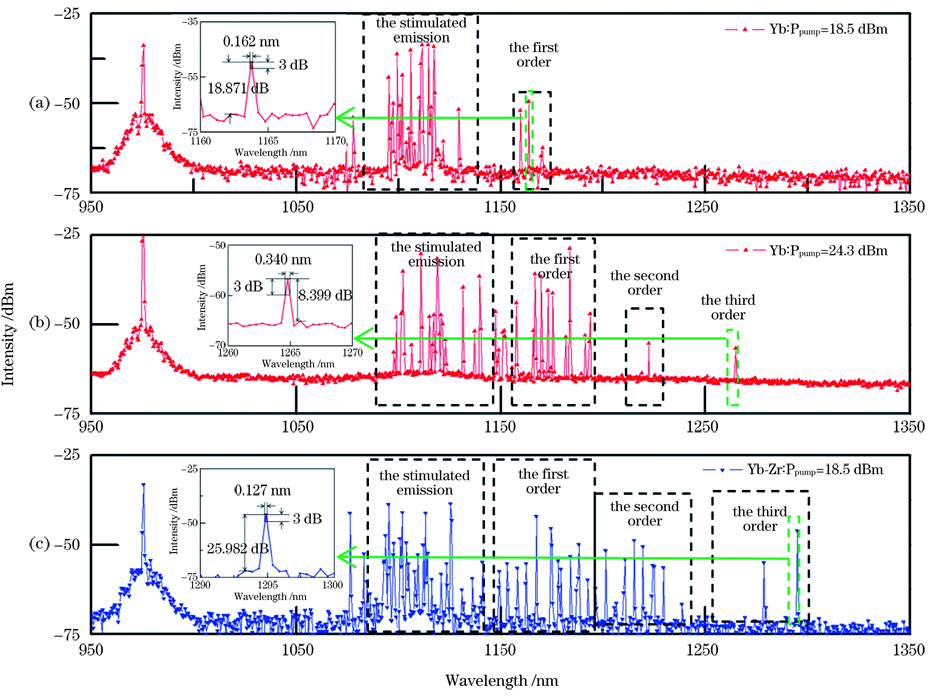
图 4. 在976 nm激光泵浦下Yb3+单掺与Yb3+-Zr4+共掺微球自激发拉曼激光光谱(插图:最高阶自激发拉曼激光的局部细节图)。(a)泵浦功率为18.5 dBm时Yb3+单掺SiO2微球腔一阶自激发拉曼激光光谱;(b)泵浦功率为24.3 dBm时Yb3+单掺SiO2微球腔三阶自激发拉曼激光光谱;(c)泵浦功率为18.5 dBm时Yb3+-Zr4+共掺SiO2微球腔三阶自激发拉曼激光光谱, 第三阶激光波长为1295 nm
Fig. 4. Yb3+ doped and Yb3+-Zr4+ co-doped self-stimulated Raman scattering spectrum under same pump wavelength but different pump powers(inset: a partial detail view of the highest order self-stimulated Raman scattering laser). (a) First-order self-stimulated Raman output spectrum of Yb3+ doped microsphere obtained under pump power of 18.5 dBm; (b) third-order self-stimulated Raman output spectrum of Yb3+ doped microsphere under pump power of 24.3 dBm; (c) third-order self-stimulated Raman spectrum of Yb3+-Zr4+ co-doped microsphere under pump power of 18.5 dBm, with third-order wavelength of 1295 nm
4.2 自激发拉曼激光阈值及泵浦光的转换效率
按
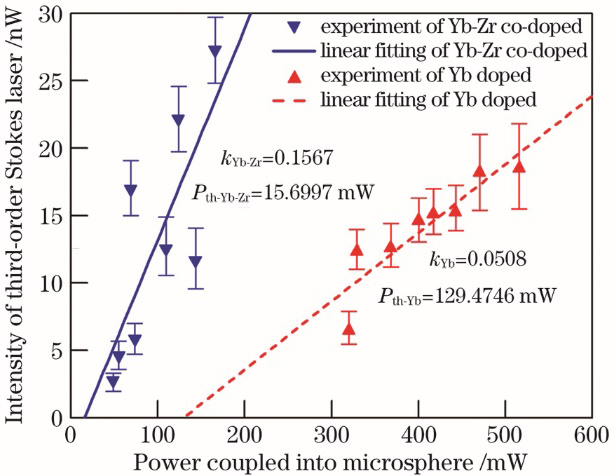
图 5. 掺Yb3+和Yb3+-Zr4+共掺微球的三阶斯托克斯激光阈值及斜率效率对比
Fig. 5. Comparison of third-order Stokes laser threshold and conversion efficiency of Yb3+-doped and Yb3+-Zr4+ co-doped microsphere
4.3 材料拉曼增益系数估算及比较
根据以上测试结果对微球材料掺Zr4+离子与否的拉曼增益系数进行估算。超高品质因子微腔中WGM的有效模式体积通常使用下式近似[40]
代入微球腔内电磁场各分量可得近似表达式
由(3)式可得拉曼增益因子的近似公式[40]
除β(与耦合参数相关的常数,在锥光纤相同的情况下,其值近似相等)以外,式中所有参数都可通过实验确定。由以上测试结果,能够获得摩尔分数0%与10% Zr4+掺杂的拉曼增益因子gR。将实验测得的各参量代入(17)式,计算可得:当溶胶凝胶中Zr4+的摩尔分数由0%增加至10%时,以0%掺杂的gR为1,10%掺杂的gR值增加到18.97,即实验结果表明摩尔分数10%的Zr4+掺杂使掺镱二氧化硅的拉曼增益因子增加了18.97倍。
5 结论
本文采用双锥光纤与高Q微球腔耦合系统研究了自激发拉曼激光增强现象。用溶胶-凝胶法和粘涂法在SiO2微球外层镀上单掺Yb3+ 与Yb3+ -Zr4+共掺SiO2薄膜。理论分析表明,Zr4+离子掺杂的膜层,一方面增加了介质的极化率,从而增大拉曼增益因子gR,另一方面通过增大膜层折射率,使回廊模在膜层聚集,减小了模式体积提高了WGM的功率密度,二者都有利于降低拉曼激光阈值、提高自激发拉曼激光效率。对比摩尔分数0%、5%和10%的Zr4+掺杂所增强的三阶自激发拉曼增益系数,实验结果表明,当掺杂Yb3+的摩尔分数为4%,Zr4+为10%时,与单掺Yb3+相比拉曼增益因子增大了约19倍,三阶自激发拉曼激光斜率效率增加了3.09倍,第三级自激发波长扩展到1295 nm。 Zr4+离子掺杂对掺Yb3+ SiO2微球自激发拉曼激光的增强作用,与理论分析相一致。通过改进Yb3+-Zr4+共掺方法与比例,以及改进微球腔的制备工艺,增加品质因子,有望获得更低阈值、更高阶数的自激发拉曼散射激光。该系统的优势在于结构紧凑、制作简易、重复性好,自激发拉曼激光性能较好。
[1] Chang Y T, Su K W, Chang H L, et al. Compact efficient Q-switched eye-safe laser at 1525 nm with a double-end diffusion-bonded Nd∶YVO4 crystal as a self-Raman medium[J]. Optics Express, 2009, 17(6): 4330-4335.
[4] Zhang H N, Chen X H, Wang Q P, et al. Dual-wavelength actively Q-switched diode-end-pumped ceramic Nd∶YAG/BaWO4 Raman laser operating at 1240 and 1376 nm[J]. Laser Physics Letters, 2014, 11(10): 105806.
[5] Chen Y F, Huang H Y, Lee C C, et al. High-power diode-pumped Nd∶GdVO4/KGW Raman laser at 578 nm[J]. Optics Letters, 2020, 45(19): 5562-5565.
[6] Lan R J, Zhang F, Wang Z P, et al. Efficient near-infrared, multiwavelengths PbWO4 Raman laser[J]. Optical Engineering, 2017, 56(9): 096112.
[7] Ren X K, Xie J, Ruan S C, et al. Diode-end-pumped solid state ZnWO4 Raman laser at 2254 nm[J]. Laser Physics, 2020, 30(1): 015001.
[8] 徐瑾瑾, 张行愚, 丛振华, 等. Nd 3+∶YAG/KTiOAsO4可调谐拉曼激光器[J]. 中国激光, 2020, 47(6): 0601002.
[9] 任席奎, 谢建, 阮双琛, 等. 基于ZnWO4/Nd∶YAG的一阶斯托克斯拉曼激光器[J]. 中国激光, 2020, 47(6): 0601003.
[10] 任席奎, 谢建, 阮双琛, 等. 1318 nm ZnWO4/Nd∶YAG二阶拉曼激光器[J]. 光学学报, 2020, 40(5): 0536001.
[11] 赵辉, 王浩宇, 朱思祁, 等. 578.5 nm端面泵浦被动调Q拉曼黄光激光器[J]. 激光与光电子学进展, 2021, 58(1): 0114004.
[12] Wei G Q, Yu Y, Zhuo M P, et al. Organic single-crystalline whispering-gallery mode microlasers with efficient optical gain activated via excited state intramolecular proton transfer luminogens[J]. Journal of Materials Chemistry C, 2020, 8(34): 11916-11921.
[13] Li X J, Wang K Y, Chen M M, et al. Stable whispering gallery mode lasing from solution-processed formamidinium lead bromide perovskite microdisks[J]. Advanced Optical Materials, 2020, 8(15): 2000030.
[14] Fabitha K, Wakiyama Y, Oshima H, et al. Realization of sharp visible WGM lasing from Sm 3+∶ZnO micro-spheres fabricated by laser ablation technique[J]. Journal of Physics D: Applied Physics, 2020, 53(13): 135302.
[15] 张培进, 黄玉, 郭长磊, 等. 976 nm激光抽运二氧化硅微球级联拉曼散射激光的研究[J]. 物理学报, 2013, 62(22): 224207.
Zhang P J, Huang Y, Guo C L, et al. Study of cascaded Raman scattering laser in silica microsphere pumped by 976 nm laser[J]. Acta Physica Sinica, 2013, 62(22): 224207.
[16] Min B, Kippenberg T J, Vahala K J. Compact, fiber-compatible, cascaded Raman laser[J]. Optics Letters, 2003, 28(17): 1507-1509.
[17] Min B, Kippenberg T J, Yang L, et al. Erbium-implanted high-Q silica toroidal microcavity laser on a silicon chip[J]. Physical Review A, 2004, 70(3): 033803.
[18] Kippenberg T J, Spillane S M, Min B, et al. Theoretical and experimental study of stimulated and cascaded Raman scattering in ultrahigh-Q optical microcavities[J]. IEEE Journal of Selected Topics in Quantum Electronics, 2004, 10(5): 1219-1228.
[19] Yang L, Carmon T, Min B, et al. Erbium-doped and Raman microlasers on a silicon chip fabricated by the sol-gel process[J]. Applied Physics Letters, 2005, 86(9): 091114.
[20] Spillane S M, Kippenberg T J, Vahala K J. Ultralow-threshold Raman laser using a spherical dielectric microcavity[J]. Nature, 2002, 415(6872): 621-623.
[21] 黄衍堂, 彭隆祥, 庄世坚, 等. 掺钕微球的受激辐射激光和自受激拉曼散射[J]. 物理学报, 2017, 66(24): 244208.
Huang Y T, Peng L X, Zhuang S J, et al. Stimulated lasing and self-excited stimulated Raman scattering of Nd 3+ doped silica microsphere pumped by 808 nm laser[J]. Acta Physica Sinica, 2017, 66(24): 244208.
[22] 吴天娇, 黄衍堂, 马靖, 等. 掺Yb 3+磷硅酸盐微球腔发光特性的探究[J]. 物理学报, 2014, 63(21): 217805.
Wu T J, Huang Y T, Ma J, et al. Study on luminescent properties of Yb 3+-doped phosphosilicate microsphere[J]. Acta Physica Sinica, 2014, 63(21): 217805.
[25] Choi H, Armani A M. High efficiency Raman lasers based on Zr-doped silica hybrid microcavities[J]. ACS Photonics, 2016, 3(12): 2383-2388.
[26] Choi H, Armani A M. Raman-Kerr frequency combs in Zr-doped silica hybrid microresonators[J]. Optics Letters, 2018, 43(12): 2949-2952.
[27] Farrow L A, Vogel E M. Raman spectra of phosphate and silicate glasses doped with the cations Ti, Nb and Bi[J]. Journal of Non-Crystalline Solids, 1992, 143: 59-64.
[28] Irimpan L, Nampoori V P N, Radhakrishnan P, et al. Size-dependent enhancement of nonlinear optical properties in nanocolloids of ZnO[J]. Journal of Applied Physics, 2008, 103(3): 033105.
[30] Colomban P, Slodczyk A. Raman intensity: an important tool in the study of nanomaterials and nanostructures[J]. Acta Physica Polonica A, 2009, 116(1): 7-12.
[31] Kippenberg T J, Spillane S M, Armani D K, et al. Ultralow-threshold microcavity Raman laser on a microelectronic chip[J]. Optics Letters, 2004, 29(11): 1224-1226.
[32] Stolen R H, Ippen E P. Raman gain in glass optical waveguides[J]. Applied Physics Letters, 1973, 22(6): 276-278.
[33] Rivero C, Richardson K, Stegeman R, et al. Quantifying Raman gain coefficients in tellurite glasses[J]. Journal of Non-Crystalline Solids, 2004, 345/346: 396-401.
[34] Coccioli R, Boroditsky M, Yablonovitch E, et al. Smallest possible electromagnetic mode volume in a dielectric cavity[J]. IEE Proceedings-Optoelectronics, 1998, 145(6): 391-397.
[35] Ostby E P, Yang L, Vahala K J. Ultralow-threshold Yb 3+∶SiO2 glass laser fabricated by the solgel process[J]. Optics Letters, 2007, 32(18): 2650-2652.
[36] Liu J H, Griebner U, Petrov V, et al. Efficient continuous-wave and Q-switched operation of a diode-pumped Yb∶KLu(WO4)2 laser with self-Raman conversion[J]. Optics Letters, 2005, 30(18): 2427-2429.
[37] 郭长磊, 黄玉, 张培进, 等. 976 nm光抽运掺Er 3+二氧化硅微球产生激光的研究[J]. 中国激光, 2013, 40(3): 0302004.
[38] Del’Haye P, Arcizet O, Gorodetsky M L, et al. Frequency comb assisted diode laser spectroscopy for measurement of microcavity dispersion[J]. Nature Photonics, 2009, 3(9): 529-533.
[39] Spillane S M, Kippenberg T J, Painter O J, et al. Ideality in a fiber-taper-coupled microresonator system for application to cavity quantum electrodynamics[J]. Physical Review Letters, 2003, 91(4): 043902.
[40] Cai M, Painter O, Vahala K J. Observation of critical coupling in a fiber taper to a silica-microsphere whispering-gallery mode system[J]. Physical Review Letters, 2000, 85(1): 74-77.
Article Outline
黄衍堂, 林晟, 刘锦萍, 许灿华, 廖廷俤. 锆离子掺杂对掺镱SiO2微球自激拉曼激光的增强[J]. 中国激光, 2021, 48(24): 2401001. Yantang Huang, Sheng Lin, Jingping Liu, Canxua Xu, Tingdi Liao. Self-Stimulated Raman Laser Enhancement of Yb 3+-Zr 4+ Co-Doped Microspheres[J]. Chinese Journal of Lasers, 2021, 48(24): 2401001.