基于连续激光器的侧向拉曼散射激光雷达无盲区探测大气温度技术
Pure rotational Raman scattering lidar is an important remote sensing tool for atmospheric temperature measurement. However, traditional backward pure rotational Raman scattering lidar has limitations in achieving effective atmospheric temperature detection in the lower atmosphere due to the overlap function effect of lidar systems. We present a novel technique of lateral scanning pure rotational Raman scattering lidar for accurate measurement of atmospheric temperature without the influence of blind zones and transition zones, which employs the bistatic structure of lidar systems. The atmospheric temperature profiling is realized by the detection of high- and low-quantum-number transitions of lateral pure rotational Raman scattering spectra at different heights, which is performed by elevation angle scanning of the lateral receiver system. The biggest advantage of this technique is the utilization of continuous-wave lasers as the excitation source, which not only significantly reduces equipment costs but also facilitates convenient mobility for outdoor observations.
The lateral pure rotational Raman scattering lidar technique is studied in the accurate measurement applications of atmospheric temperature from the ground to the height of interest. First, a novel lateral scanning pure rotational Raman scattering lidar technique is proposed and systematically designed. Each telescope combined with a narrow-band interferometric filter is adopted to detect the lateral scattering signals of the low- and high-quantum-number transitions of pure rotational Raman scattering spectra. Then, the atmospheric temperature inversion algorithm for lateral scanning pure rotational Raman scattering lidar is established and the calibration function is optimized to improve the inversion accuracy of atmospheric temperature. Finally, the experimental system is constructed, and the preliminary experiments are conducted using the lateral scanning pure rotational Raman scattering lidar. Two different rotation schemes including the continuous equidistant resolution and segmented equidistant resolution are employed during the experimental observations.
The detection principle of the proposed Raman scattering lidar is innovatively presented. It breaks through the traditional backward pure rotational Raman scattering lidar using a monostatic transceiver system structure, which produces blind and transition zones that cannot realize effective detection of near-surface atmospheric temperature. Meanwhile, this technology can leverage a continuous-wave laser, which features light weight, portability, mobility, and low cost (Fig. 1). By analyzing the eight newly expanded calibration functions, the calibration function that introduces the smallest error is selected as the expression for atmospheric temperature detection by a lateral pure rotational Raman scattering lidar (Fig. 2). Based on completing the lateral pure rotational Raman scattering lidar system design, the lateral Raman scattering lidar system is constructed (Fig. 3). Preliminary experimental observational studies of a lateral scanning pure rotational Raman scattering lidar are performed by two different rotation schemes of the continuous equidistant resolution and segmented equidistant resolution, which are employed during the experimental observations. The experimental results show that the lateral scanning pure rotational Raman scattering lidar has precise detection capability of atmospheric temperature up to a height of 1400 m. Furthermore, the segmented equidistant resolution rotation scheme provides a finer spatial distribution of temperature within the height interval of 0-312 m (Figs. 4-7),compared with the continuous equidistant resolution rotation scheme.
We propose a novel lateral pure rotational Raman scattering lidar technique to realize non-blind detection of the temperature profile distribution in the lower atmosphere. The profiling of lidar returns in the lateral pure rotational Raman scattering lidar is performed by elevation angle scanning of the lateral receiving system. Meanwhile, the intensities of the lateral Raman scattering signals at each setting of elevation angles are sampled and analyzed, and the biggest advantage of this technique is that a low-cost continuous laser can be employed as the excitation light source to simplify the system and reduce costs. Additionally, the pulsed laser in the backward pure rotational Raman scattering lidar can be adopted as the transmitter to construct a lateral + backward pure rotational Raman scattering lidar for finely detecting the atmospheric temperature from the ground to the height of interest. The preliminary experimental results show that the atmospheric temperature below the height of 1400 m can be detected finely with the 60 m distance between the transmitter and receiver. The segmented equidistant resolution rotational scheme can realize a more refined temperature profile than the whole continuous equidistant resolution rotation scheme.
1 引言
大气温度是大气冷热程度的表征参数,其时空分布和变化对于大气的压力、风、湿度以及天气、气候等具有重要影响,是大气热平衡、大气物理与化学、天气预报以及环境监测等方面研究的重要参数[1-2]。大气温度由于受到地表的辐射冷却、冷暖锋等因素影响,时常产生逆温层,特别是近地表的大气温度,其变化相当复杂,这与近地表大气中发生的热力过程和水汽凝结现象有着密切关系,也与大气水循环和大气中污染物的分布、扩散及传输等具有密切的关系[3]。由于近地表层大气温度变化与人类活动密切相关,因此针对近地表大气分布特征,开展对大气温度精确探测技术的研究工作,包括其时空分布规律,这对于深入研究全球变暖与极端天气的相关性具有非常重要的意义[4]。
纯转动拉曼散射激光雷达是一种能够实现大气温度探测的重要遥感工具,其基于大气中N2和O2分子的纯转动拉曼散射高低量子数谱线与温度的敏感性原理来探测大气温度[5-6]。由于纯转动拉曼散射信号比较微弱且其谱线靠近弹性散射光谱会导致精细分离提取难度较大,研究人员在专注于大功率的脉冲激光器、大口径的接收望远镜的研究,以提升激光雷达的探测能力和探测性能之余,还会特别关注激光雷达分光系统的研制,设计了超窄带滤光片、法布里-珀罗标准具和闪耀光栅与光纤Bragg光栅组成的两级并行多通道拉曼分光系统等,这些都能够满足纯转动拉曼谱线精细提取的需求[7-9]。同时,全天时大气温度探测、数据反演和数据修正等能提升激光雷达实际应用能力的技术,也是近些年的研究热点[10]。然而,上述研究均采用传统激光雷达同轴或平行轴的单基地配置模式,其发射系统和接收系统间的匹配程度较低导致激光雷达系统存在几何重叠因子,严重限制了激光雷达对近地表大气温度的探测能力[11]。近年来,同轴激光雷达自准直系统、几何重叠因子校准算法等相继被人们所采用,以减小激光雷达几何重叠因子对激光雷达探测性能的影响,但是各种方法均未能有效地解决收发系统之间的匹配问题,在后向散射激光雷达探测近地表大气参量过程中仍具有相当的局限性[12-13]。
为有效打破后向散射激光雷达因几何重叠因子的存在导致无法对近地表大气参量进行探测这一技术壁垒,侧向散射激光雷达技术被提出,其采用CCD图像传感器作为接收装置与激光发射器共同构建出双基地的侧向图像激光雷达系统,可实现对近地表大气气溶胶光学参量的探测[14-16]。在国内,侧向图像激光雷达的研究人员对系统搭建、回波信号噪声的分析和提取、CCD像元角宽度和探测高度间的关系、大气总散射相函数探测以及与后向散射激光雷达联合探测实验等方面的研究取得了一定的成果[17-22]。但是考虑到纯转动拉曼散射信号强度比米氏-瑞利(Mie-Rayleigh)散射信号弱了2~3个数量级,因此很难使用图像传感器作为接收器件对近地表大气温度敏感性相关的纯转动拉曼散射高低量子数谱线进行探测。
本文以近地表大气温度的精细探测为目标,提出了一种新型的侧向扫描纯转动拉曼散射激光雷达探测技术,有效解决了后向纯转动拉曼散射激光雷达受几何重叠因子影响而无法对近地表大气温度进行有效探测的难题。该技术的最大优势是采用连续激光器作为激励光源,还具有轻量化、低成本、便于移动以进行户外观测的优势。将基于双望远镜和超窄带干涉滤光片的侧向接收系统置于云台上,利用云台的俯仰扫描功能实现高低量子数通道的侧向纯转动拉曼散射回波信号廓线的收集。实验初步设计了两种扫描探测方案,均验证了侧向纯转动拉曼散射激光雷达系统可实现0~1400 m范围内的大气温度探测。
2 侧向纯转动拉曼散射激光雷达系统设计
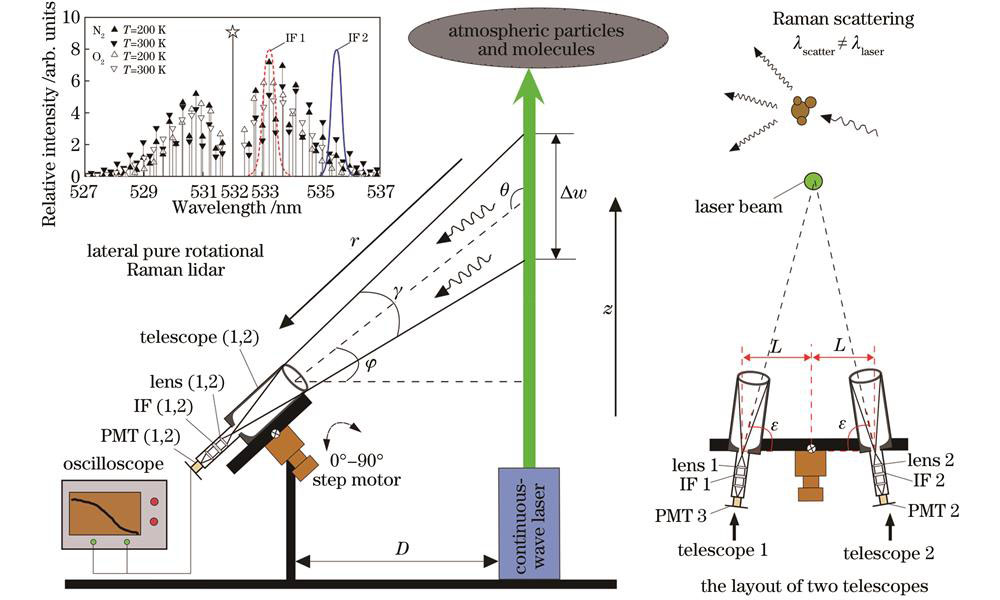
图 1. 基于连续激光器的侧向纯转动拉曼散射激光雷达系统结构及探测原理图
Fig. 1. Structure and detection principle diagram of lateral pure rotational Raman scattering lidar system with continuous-wave laser
表 1. 基于连续激光器的侧向纯转动拉曼散射激光雷达的系统参数
Table 1. Parameters of lateral pure rotational Raman scattering lidar system based on continuous-wave laser
|
在温度测量中,考虑到波长为532.1 nm的可见光激发的荧光效应极其微弱,并且纯转动拉曼谱线的Stokes分支强度比Anti-Stokes分支强,因此选择强度较强的Stokes分支。同时为了提高探测灵敏度并减少弹性散射信号的干扰,实验采用的高低量子数通道的中心波长由温度不确定度确定。选取Stokes分支低量子数通道的三条氮气谱线(J=4、J=5、J=6),中心波长为533.24 nm;选取高量子数通道的三条氮气谱线(J=14、J=15、J=16),中心波长为535.52 nm。在侧向纯转动拉曼散射激光雷达系统中,低量子数通道的侧向纯转动拉曼散射信号被光学望远镜telescope 1接收,经透镜lens 1准直后,再经中心波长为533.24 nm、带宽为0.45 nm的干涉滤光片IF 1进行滤波,作为侧向纯转动拉曼散射低量子数探测通道;高量子数通道的侧向纯转动拉曼散射信号被光学望远镜telescope 2接收,经透镜lens 2准直后,再经中心波长为535.52 nm、带宽为0.45 nm的滤光片IF 2进行滤波,作为侧向纯转动拉曼散射高量子数探测通道。各通道的侧向纯转动拉曼散射回波信号由PMT进行光电转换,并送入数据采集系统。NI示波器对每个俯仰角下的侧向纯转动拉曼散射回波信号进行20 s时间累积的数据采集和数据存储。值得注意的是,侧向纯转动拉曼激光雷达的分光系统集成在每个光学望远镜的出光口处,便于户外移动并进行野外观测。基于连续激光器的侧向纯转动拉曼散射激光雷达的系统参数如
3 侧向纯转动拉曼散射激光雷达探测大气温度技术
3.1 侧向纯转动拉曼散射激光雷达的探测原理及大气温度反演算法
侧向纯转动拉曼散射激光雷达探测大气温度技术基于纯转动拉曼散射原理,其中,激光雷达发射系统和侧向望远镜接收系统采用双基地模式,侧向望远镜接收系统通过俯仰扫描实现对不同高度处激光束的扫描探测,有效解决了后向纯转动拉曼散射激光雷达受几何重叠因子影响而无法对近地表大气温度进行探测的问题。根据收发分置的双基地侧向散射激光雷达系统结构,侧向纯转动拉曼散射激光雷达回波信号可表示为
式中:E(z, λ0, λX, θ)是当探测高度为z且散射角为θ时的侧向纯转动拉曼散射回波信号的接收功率;E0是连续激光器的初始功率;A是光学望远镜的有效接收面积;K是包含望远镜接收光学效率和PMT量子效率的系统常数;D是发射系统和接收系统之间的水平距离;τi(λX)是分子各转动拉曼谱线对应波长下滤光片的透过率;ηi为N2和O2分子所占大气体积比;dπσX(J, T)/dΩ为分子各条纯转动拉曼散射光谱的后向散射截面;N(z)为大气分子密度; f(θ)为归一化的侧向散射相函数;Tz(z, λ0)为激光发射波长为λ0的激光束射向高度为z的去程大气透过率;TX(z, λX, θ)为纯转动拉曼波长λX下侧向望远镜所接收的高度z处的斜程大气透过率,下标X表示高低量子数通道的纯转动拉曼散射光谱。
侧向纯转动拉曼散射激光雷达的去程大气透过率和斜程大气透过率分别表示为
式中:α(z, λ0)和α(z, λ
根据侧向拉曼散射原理,侧向纯转动拉曼散射高量子数通道和低量子数通道的激光雷达方程可分别表示为
利用纯转动拉曼散射激光雷达探测大气温度时,通过构建两个温度灵敏度相反的高量子数通道和低量子数通道的信号强度之比Q(T, z)来反演大气温度信息。侧向纯转动拉曼激光雷达反演近地表大气温度算法可表示为
式中:ΔfLow/High是大气分子散射相函数的校正系数,考虑到散射角θ是影响大气分子散射相函数的唯一参数,而利用双望远镜接收时其散射角保持一致,因此可认为ΔfLow/High等于1;ΔTr(z, λLow, λHigh, θ)是在斜程路径上因纯转动拉曼散射高低量子数通道的波长差所引起的大气透过率的差异,但是考虑到两个通道的波长差极小(~2.28 nm)且距离侧向接收系统的斜程距离是一致的,因此在近地表大气温度反演算法中忽略了气溶胶分布对探测结果的影响。
在大气温度反演算法中,高低量子数通道的信号强度之比Q(T, z)与温度T(z)之间的关系式可进一步表示为
式中:a、b为侧向纯转动拉曼散射激光雷达系统的校正参数,可通过无线电探空仪进行标定。本文中对Q(T, z)添加多次项构成非线性关系以提高对大气温度的反演精度,同时引入了新的校正参数c、d[23]。
3.2 标定函数的优化
在实验过程中,使用滤光片对两路温度灵敏度相反的高低量子数纯转动拉曼散射信号进行提取,考虑到滤波器存在一定的带宽,在提取单条谱线的同时往往会混入所提取谱线周围相邻的N2和O2分子的拉曼谱线,因此不能单一 使用
表 2. 添加x=1/T和y=ln Q的标定函数
Table 2. Add the calibration function of x=1/T and y=ln Q
|
在温度测量过程中,通过
在第一类标定函数CF 1~CF 4中,标定函数CF 1的引入误差最小。在第二类标定函数CF 5~CF 8中,标定函数CF 8的引入误差最小。对比CF 1和CF 8,其中CF 8的引入误差较小,因此采用CF 8作为标定函数,具体温度表达式为
4 实验观测
在完成侧向纯转动拉曼散射激光雷达系统设计的基础上,搭建了侧向扫描纯转动拉曼散射激光雷达系统,并开展了初步的实验观测研究。
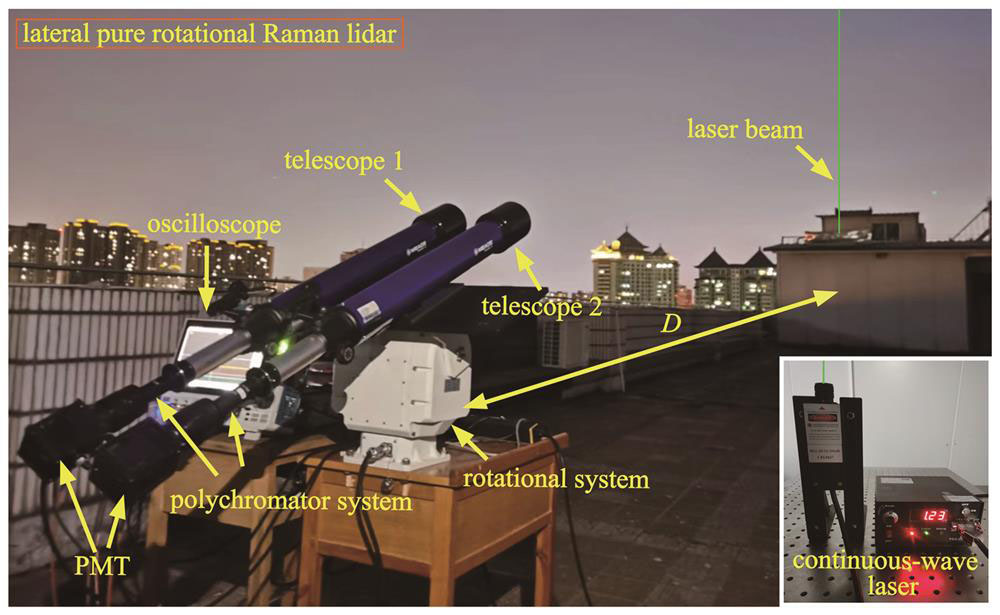
图 3. 基于连续激光器的侧向纯转动拉曼散射激光雷达实验系统
Fig. 3. Experimental system of lateral pure rotational Raman scattering lidar with continuous-wave laser
系统实验设计了两种不同的云台俯仰扫描转动方案,以满足近地表无盲区大气温度精细探测的不同需求。方案一为整段等距分辨率云台转动方案,从37个角度进行采样,探测高度可达1296 m,整个采样周期保持36 m的距离分辨率,云台俯仰角度从0°变化到87.4°完成对一整条廓线的完整采样。方案二是分段等距分辨率云台转动方案,共设置41个俯仰角度来实现1332 m的采样探测高度。第一个俯仰扫描阶段探测高度为0~72 m,云台从0°转动至50.2°,距离分辨率为7.2 m,采样点为11个;第二个俯仰扫描阶段探测高度为72~312 m,云台从50.2°转动至79.1°,距离分辨率为24 m,采样点为10个;第三个俯仰扫描阶段探测高度为312~732 m,云台从79.1°转动至85.3°,距离分辨率为42 m,采样点为10个;第四个俯仰扫描阶段探测高度为732~1332 m,云台从85.3°转动至87.4°,距离分辨率为60 m,采样点为10个。方案二与方案一相比,云台在低层大气探测时俯仰扫描转动角的变化相对更小,可实现对低层大气温度垂直结构更为精细的探测。两种云台转动方案在各角度采样时间均为20 s的情况下,采用整段等距分辨率云台转动方案完成一次大气廓线的采集时间为14.3 min,采用分段等距分辨率云台转动方案完成一次大气廓线的采集时间为16.0 min。在方案一和方案二的各实验探测结果(图
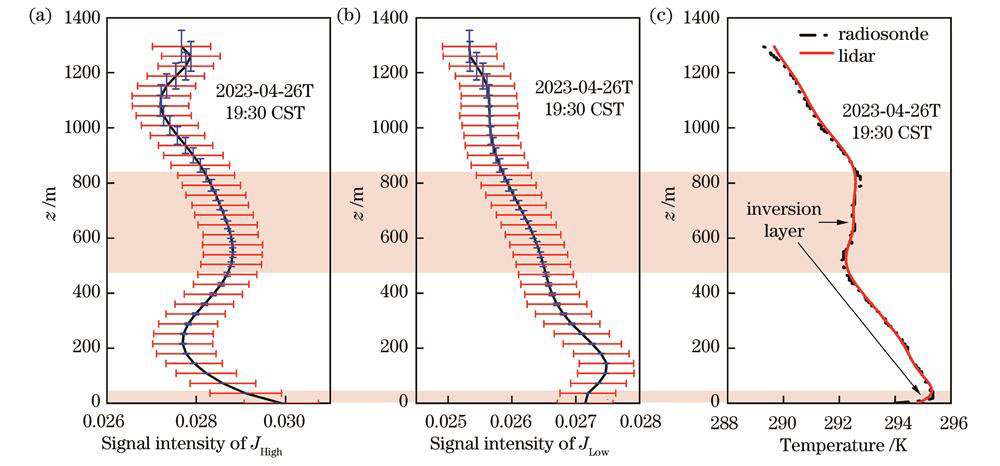
图 4. 2023年4月26日19:30 CST侧向纯转动拉曼散射激光雷达的大气温度探测结果。(a)高量子数通道的侧向纯转动拉曼散射回波信号强度;(b)低量子数通道的侧向纯转动拉曼散射回波信号强度;(c)大气温度的反演结果
Fig. 4. Detection results of atmospheric temperature with lateral pure rotational Raman scattering lidar at 19: 30 CST on April 26, 2023. (a) Lateral pure rotational Raman scattering echo signal intensity of high-quantum-number channel; (b) lateral pure rotational Raman scattering echo signal intensity of low-quantum-number channel; (c) inversion results of atmospheric temperature
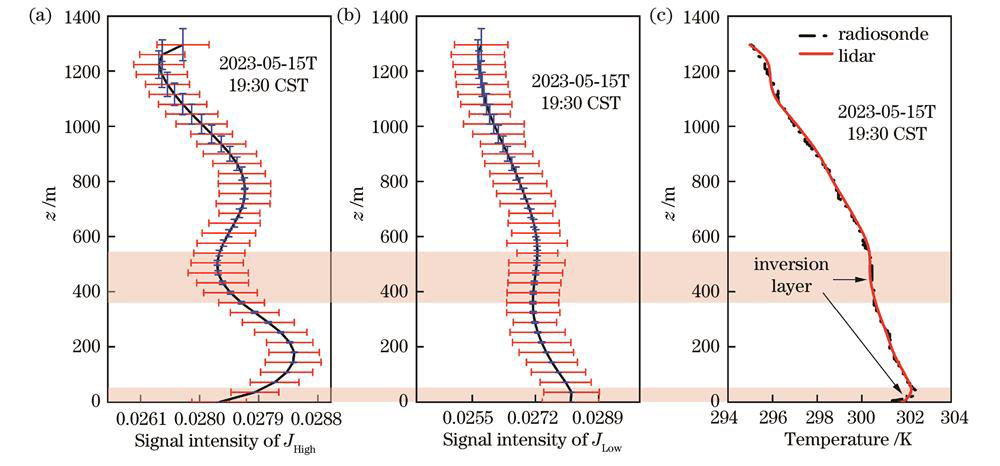
图 5. 2023年5月15日19:30 CST侧向纯转动拉曼散射激光雷达的大气温度探测结果。(a)高量子数通道的侧向纯转动拉曼散射回波信号强度;(b)低量子数通道的侧向纯转动拉曼散射回波信号强度;(c)大气温度的反演结果
Fig. 5. Detection results of atmospheric temperature with lateral pure rotational Raman scattering lidar at 19: 30 CST on May 15, 2023. (a) Lateral pure rotational Raman scattering echo signal intensity of high-quantum-number channel; (b) lateral pure rotational Raman scattering echo signal intensity of low-quantum-number channel; (c) inversion results of atmospheric temperature
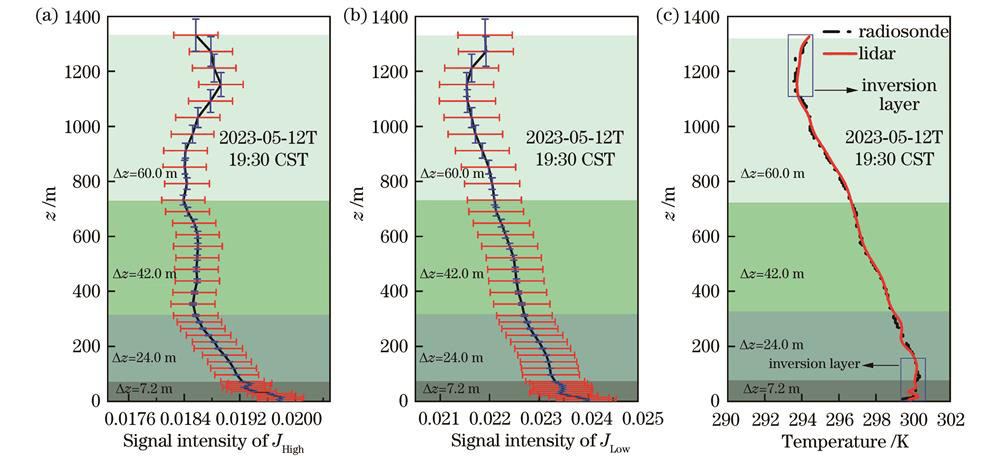
图 6. 2023年5月12日19:30 CST侧向纯转动拉曼散射激光雷达的大气温度探测结果。(a)高量子数通道的侧向纯转动拉曼散射回波信号强度;(b)低量子数通道的侧向纯转动拉曼散射回波信号强度;(c)大气温度的反演结果
Fig. 6. Detection results of atmospheric temperature with lateral pure rotational Raman scattering lidar at 19: 30 CST on May 12, 2023. (a) Lateral pure rotational Raman scattering echo signal intensity of high-quantum-number channel; (b) lateral pure rotational Raman scattering echo signal intensity of low-quantum-number channel; (c) inversion results of atmospheric temperature
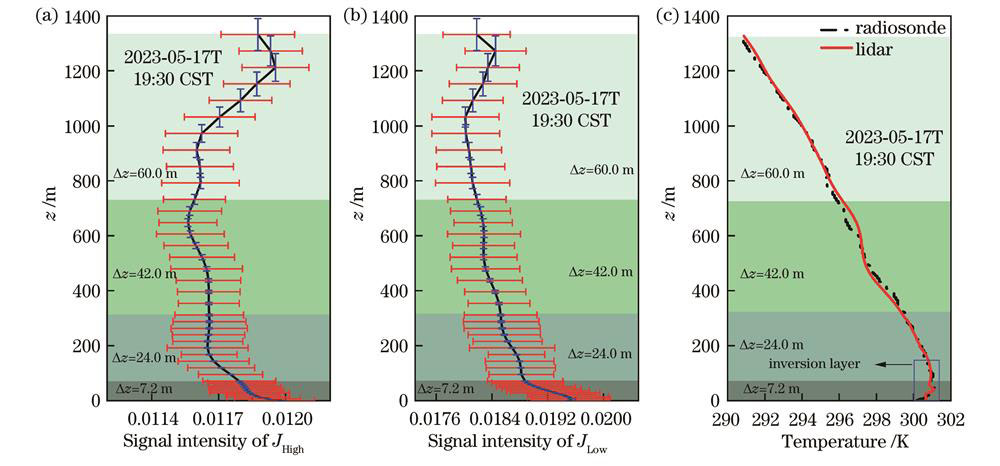
图 7. 2023年5月17日19:30 CST侧向纯转动拉曼散射激光雷达的大气温度探测结果。(a)高量子数通道的侧向纯转动拉曼散射回波信号强度;(b)低量子数通道的侧向纯转动拉曼散射回波信号强度;(c)大气温度的反演结果
Fig. 7. Detection results of atmospheric temperature with lateral pure rotational Raman scattering lidar at 19: 30 CST on May 17, 2023. (a) Lateral pure rotational Raman scattering echo signal intensity of high-quantum-number channel; (b) lateral pure rotational Raman scattering echo signal intensity of low-quantum-number channel; (c) inversion results of atmospheric temperature
1) 基于整段等距分辨率云台转动方案的实验探测结果
侧向纯转动拉曼散射激光雷达系统采用整段等距分辨率的云台转动方案可实现0~1296 m高度范围内的大气温度探测,整条廓线具有相同的36 m的距离分辨率。
2) 基于分段等距分辨率云台转动方案的实验探测结果
侧向纯转动拉曼散射激光雷达系统采用分段等距分辨率的云台转动方案可实现0~1332 m高度范围内的大气温度探测,整条廓线采用4段不同的距离分辨率,能够更加精细地探测出近地表大气温度的波动情况。
5 结论
提出一种新型的侧向纯转动拉曼散射激光雷达技术,可实现无盲区探测低层大气温度的廓线分布,有效解决后向拉曼散射激光雷达因几何重叠因子的存在而对低层大气探测结果产生影响的问题。考虑到侧向纯转动拉曼散射激光雷达系统探测大气温度廓线可通过侧向接收系统的俯仰扫描实现,且该方法的最大优势是使用低成本的连续激光器作为激励光源,能够简化系统,降低成本。同时该系统也可以与后向纯转动拉曼散射激光雷达共用脉冲激光器,构建侧向+后向纯转动拉曼散射激光雷达,以实现从地表到任意高度大气温度的精细探测。系统初步实验探测结果表明,系统在收发装置距离为60 m的条件下,可以实现1400 m高度以下的大气温度的精细探测,且分段等距分辨率云台转动方案相比于整段等距分辨率云台转动方案可得到更为精细的温度廓线分布结构。
[1] Wulfmeyer V, Hardesty R M, Turner D D, et al. A review of the remote sensing of lower tropospheric thermodynamic profiles and its indispensable role for the understanding and the simulation of water and energy cycles[J]. Reviews of Geophysics, 2015, 53(3): 819-895.
[2] Tamarin-Brodsky T, Hodges K, Hoskins B J, et al. A dynamical perspective on atmospheric temperature variability and its response to climate change[J]. Journal of Climate, 2019, 32(6): 1707-1724.
[3] 龚少华, 陈伟鹏, 杨国韬, 等. 基于Rayleigh激光雷达实验观测的中层大气温度反演与重力波事件识别[J]. 光学学报, 2023, 43(24): 2428006.
[4] Munguira A, Hueso R, Sánchez-Lavega A, et al. Near surface atmospheric temperatures at Jezero from Mars 2020 MEDA measurements[J]. Journal of Geophysical Research: Planets, 2023, 128(3): e2022JE007559.
[5] Whiteman D N. Examination of the traditional Raman lidar technique. I. Evaluating the temperature-dependent lidar equations[J]. Applied Optics, 2003, 42(15): 2571-2592.
[6] 刘东, 姚清睿, 张思诺, 等. 拉曼激光雷达大气温湿压探测技术研究进展[J]. 中国光学(中英文), 2023, 16(2): 243-257.
[7] Weng M, Yi F, Liu F C, et al. Single-line-extracted pure rotational Raman lidar to measure atmospheric temperature and aerosol profiles[J]. Optics Express, 2018, 26(21): 27555-27571.
[8] Pan X L, Yi F, Liu F C, et al. Diurnal temperature variations in the lower troposphere as measured by an all-day-operational pure rotational Raman lidar[J]. Applied Optics, 2020, 59(28): 8688-8696.
[9] 李启蒙, 李仕春, 秦宇丽, 等. 绝对测温转动拉曼激光雷达分光系统设计及性能[J]. 物理学报, 2018, 67(1): 014207.
Li Q M, Li S C, Qin Y L, et al. Design and performance of spectroscopic filter of rotational Raman temperature lidar for absolute measurement[J]. Acta Physica Sinica, 2018, 67(1): 014207.
[10] 高飞, 黄波, 石冬晨, 等. 全天时大气温度探测的纯转动拉曼激光雷达系统设计与仿真[J]. 光学学报, 2019, 39(3): 0301004.
[11] Sassen K, Dodd G C. Lidar crossover function and misalignment effects[J]. Applied Optics, 1982, 21(17): 3162-3165.
[12] 宋跃辉, 鲁雷雷, 华灯鑫, 等. 同轴米散射激光雷达自准直系统设计[J]. 中国激光, 2016, 43(7): 0710001.
[13] 王欣红, 狄慧鸽, 王娅娅, 等. 基于气溶胶光学参数的拉曼激光雷达重叠因子校正方法[J]. 光学学报, 2023, 43(6): 0601005.
[14] Barnes J E, Bronner S, Beck R, et al. Boundary layer scattering measurements with a charge-coupled device camera lidar[J]. Applied Optics, 2003, 42(15): 2647-2652.
[15] Wang Z Z, Tao Z M, Liu D, et al. New experimental method for lidar overlap factor using a CCD side-scatter technique[J]. Optics Letters, 2015, 40(8): 1749-1752.
[16] Ma X M, Wang Z Z, Zhang H, et al. Detecting aerosol backscattering coefficient across the whole troposphere by the sidescattering lidar system with three CCD cameras[J]. Optics Express, 2022, 30(17): 29969-29978.
[17] 孟祥谦, 胡顺星, 王珍珠, 等. CCD激光雷达探测边界层气溶胶消光系数垂直分布[J]. 光学学报, 2013, 33(8): 0801003.
[18] 孟祥谦, 胡顺星, 王英俭, 等. 基于电荷耦合器件探测气溶胶散射相函数与大气能见度的研究[J]. 光学学报, 2012, 32(9): 0901001.
[19] 陶宗明, 刘东, 麻晓敏, 等. 基于CCD的侧向激光雷达系统研制及探测个例[J]. 红外与激光工程, 2014, 43(10): 3282-3286.
Tao Z M, Liu D, Ma X M, et al. Development and case study of side-scatter lidar system based on charge-coupled device[J]. Infrared and Laser Engineering, 2014, 43(10): 3282-3286.
[20] 麻晓敏, 陶宗明, 马明俊, 等. 基于CCD的侧向散射激光雷达信号提取方法[J]. 光学学报, 2014, 34(2): 0201001.
[21] 麻晓敏, 史博, 单会会, 等. 基于CCD成像的侧向散射激光雷达几何标定方法[J]. 光子学报, 2015, 44(2): 0201002.
[22] Bian Y X, Zhao C S, Xu W Y, et al. Development and validation of a CCD-laser aerosol detective system for measuring the ambient aerosol phase function[J]. Atmospheric Measurement Techniques, 2017, 10(6): 2313-2322.
[23] Gerasimov V V, Zuev V V. Analytical calibration functions for the pure rotational Raman lidar technique[J]. Optics Express, 2016, 24(5): 5136-5151.
Article Outline
杨帆, 高飞, 高雄, 李晓莉, 汪丽, 闫庆, 石冬晨, 宋跃辉, 李仕春, 华灯鑫. 基于连续激光器的侧向拉曼散射激光雷达无盲区探测大气温度技术[J]. 光学学报, 2024, 44(6): 0601015. Fan Yang, Fei Gao, Xiong Gao, Xiaoli Li, Li Wang, Qing Yan, Dongchen Shi, Yuehui Song, Shichun Li, Dengxin Hua. Non-Blind Zone Detection of Atmospheric Temperature Using Lateral Raman Scattering Lidar Based on Continuous-Wave Laser[J]. Acta Optica Sinica, 2024, 44(6): 0601015.