基于双芯光子晶体光纤的耦合器设计方法
Optical fiber directional coupler can realize beam coupling and splitting, which is one of the most important passive devices in the optical fiber communication system after the optical connector. In recent years, with the wide application of various high-capacity wavelength division multiplexing (WDM) communication networks, the bandwidth characteristics of optical fiber directional couplers are facing great challenges. As a practical product, the fabrication technology of optical fiber couplers based on the melt-drawing cone process is very mature and has the advantages of good directionality, high-temperature stability, and low cost. However, due to the strong dependence of the beam-splitting ratio on the operating wavelength, most of the current optical fiber directional couplers operate at specific wavelengths and have narrow bandwidths. The advent of photonic crystal fibers (PCFs) makes it possible to design fiber couplers with higher performance. Compared with traditional fiber couplers, PCF-based couplers have the advantages of low wavelength dependence, short coupling length, and low insertion loss, and they have become a hot spot in related research fields. Among them, the dual-core PCF-based directional coupler has been reported in many experimental and theoretical studies. However, most researchers usually give the structure and parameters of the designed coupler directly and do not make a more detailed summary and generalization of the specific rules of parameter design. If the specific rules of PCF parameter design are summarized in detail, it can provide a meaningful reference for the efficient design of broadband optical fiber directional couplers.
In a dual-core fiber, each core region can be considered as an independent optical waveguide, and the optical field energy conducted within each core is affected by the other core and changes periodically with the length of the coupling region. According to the coupling theory, it can be seen that for two parallel lossless optical waveguides, the internal optical field energy can be expressed by Eqs. (1) and (2), respectively. Eq. (3) indicates that when the two waveguides and their nearby spatial structure and material are consistent, the effective coupling coefficient is equal to the coupling coefficient. When the two waveguides and their nearby spatial structure or material are inconsistent, the effective coupling coefficient will be greater than the coupling coefficient, in which case there will be no specific interaction length so that the incident light field energy can be completely into the coupling arm. At this point, by changing the fiber structure parameters, the effective coupling coefficient can be adjusted without changing the interaction length, and then the desired coupling ratio can be obtained near the center of the target wavelength range with a shorter interaction length. In this paper, the coupling characteristics of the coupler structure under different fiber structure parameters are simulated by using the beam transmission method to obtain the variation of the optical field energy in the structure with the transmission distance. For the determined fiber structure, the variation curves of the optical field energy in the straight through arm and the coupling arm satisfy Eqs. (1) and (2), respectively. Specifically, I1 and I2 are functions of the variable L and the unknown constants κ and δ, which exactly satisfy the applicable conditions for estimating the constants using the least squares method. Since the beam transmission method can provide a sufficiently large number of simulation data points, Eq. (5) is overdetermined, and the equations can be solved by curve fitting using the trust-domain reflection algorithm. After κ and δ are obtained using the least squares method, the difference in transmission constants between the cores of the dual-core PCF fibers at this point can also be obtained from Eq. (3).
The influence of structural parameters on the inter-core coupling efficiency in dual-core PCF fibers is discussed in detail. According to the analysis results of Fig. 6, it can be seen that changing the hole spacing and air hole symmetry can realize the coarse adjustment of the coupling region length; changing the air hole symmetry can realize the coarse adjustment of the maximum coupling ratio; changing the core refractive index difference, the central air hole diameter ratio, and the core diameter ratio can realize the fine adjustment of the coupling region length and the maximum coupling ratio. To verify the above theory, we design a broadband 50∶50 coupler containing 1310 nm and 1550 nm with ±5% coupling ratio. We find that the designed structure has the largest bandwidth value of 240 nm while satisfying the design requirements when L=3000 μm, d2=1.8 μm, and d0=0.51 μm. It can be seen from Fig. 8(a) that the coupling ratios of the two arms for wavelengths from 1310 nm to 1550 nm can all fall between 45% and 55%. The additional loss of the structure and the insertion loss of the straight through arm and the coupling arm with wavelength are shown in Fig. 8(b). The insertion loss of the designed structure is not higher than 0.2 dB, and the insertion loss of both arms is around 3 dB.
In this paper, the influence of various parameters on the coupling efficiency between cores of a dual-core PCF is analyzed by using the beam transmission method, and the coupling coefficient and other parameters between waveguides are estimated by using the least squares method according to the principle of dual-core coupling. It makes clear that in a dual-core PCF, each structural parameter regulates the coupling ratio and the length of the coupling region. By summarizing the specific rules of parameter design in detail, the coarse and fine-tuning design methods for the coupling performance of dual-core PCFs are proposed. Then, an asymmetric dual-core PCF broadband directional coupler is designed according to the proposed design method, and the coupling ratio and coupling zone length of the designed coupling structure are coarse-tuned and fine-tuned by adjusting the symmetry of the air holes and the diameter of the central air holes, respectively, enabling the coupler to achieve a coupling ratio of 50%±5% in the interval of 1. 31-1.55 μm, a bandwidth of 240 nm, and an ultra-short coupling length of 3 mm. The research results can provide a meaningful reference for the efficient design of broadband optical fiber directional couplers.
1 引言
光纤定向耦合器可以实现光的耦合和分束等功能,是光纤通信系统中仅次于光连接器的重要无源器件[1]。近年来,随着各种大容量波分复用通信网络的广泛应用,光纤定向耦合器的带宽特性面临巨大挑战。作为实用化的产品,基于熔融拉锥工艺的光纤耦合器制备技术十分成熟,且具有方向性好、温度稳定性高以及成本低等优点[2]。由于光纤定向耦合器的分束比对工作波长的依赖性较强,目前它们大都工作在特定的波长且带宽较窄[3]。
光子晶体光纤(PCF)的出现使得设计出具有更高性能的光纤耦合器成为可能。相比于传统光纤耦合器,基于PCF的耦合器具有波长依赖性弱、耦合长度短以及插入损耗低等优点,已成为相关研究领域的热点[4]。其中,基于双芯PCF的定向耦合器在实验和理论方面的研究已有不少报道。2011年,Li等[5]提出一种既采用折射率导光又采用带隙导光的高双折射混合PCF,在1.53~1.72 μm的大波长范围内,分光比优于20 dB,带宽约为190 nm。同年,Lou等[6]通过在双芯PCF中引入一个椭圆形的中心气孔来抵消双芯光纤产生的轻微双折射,实现了宽带和偏振无损光纤的耦合特性。汪徐德等[7]发现双芯PCF中耦合长度随空气填充率的增大而减小,并据此设计了多种波分复用器件。2012年,王立文等[8]提出一种可用于宽带偏振不敏感光定向耦合器的新型双芯PCF,并试制了类似结构的双芯PCF。同年,Liu等[9]提出一种基于碲酸盐玻璃双芯PCF的新型偏振耦合器,其长度为0.36 mm、工作波长为1.55 μm时带宽为20 nm。2015年,Sun等[10]从理论上研究了金属丝填充的双芯PCF,通过选择合适的光纤参数实现了在250 nm波长范围内消光比小于-20 dB的偏振耦合器。2017年,Liu等[11]提出一种基于双芯PCF的超短高灵敏度折射率传感器,光纤长度仅为3 mm,可广泛应用于集成传感系统。Wang等[12]则提出一种基于双芯方形晶格PCF的超短偏振耦合器,在1.55 μm波长处带宽约为70 nm。2018年,Xu等[13]提出一种基于双芯PCF和金线的超短偏振耦合器,该偏振分路器可实现19.2 nm的带宽。Dou等[14]则在双芯PCF的中心引入额外的能量传输通道,使其具有长度短、损耗低的特点,可用于偏振耦合器。2021年,Anbazhagan等[15]提出一种基于内嵌硅纳米线双芯PCF的偏振无关耦合器,采用108.75 μm的PCF实现了偏振无关耦合器。2022年,黄旭等[16]提出一种双芯太赫兹光纤定向耦合器,在保证两个偏振模的耦合长度差小于1%的前提下,其带宽可达到220 GHz。同年,Hagras等[17]提出并分析了紧凑型太赫兹双芯PCF偏振耦合器,在0.6 THz的中心频率附近实现了58 GHz的大带宽。2023年,杨世泰等[18]提出一种多芯光纤对角芯反射耦合器,实现了多芯光纤对称纤芯的光路低损耗串联。
上述研究通常直接给出所设计的耦合器的结构及参数,并未对参数设计的具体规律进行较为详尽的总结与归纳。本文采用光束传播法对双芯PCF进行建模,充分讨论了双芯PCF的多种结构参数对纤芯间耦合效率的具体影响。首先,根据双芯耦合原理,使用最小二乘法对波导间的耦合系数等进行估计,明确了孔间距、纤芯折射率差、中央空气孔直径比例、纤芯直径比例、空气孔直径比例和空气孔对称度等参数对双芯PCF耦合比及耦合区长度的调节作用,提出一种基于双芯PCF的耦合器性能的粗调与微调设计方法。然后,根据所提出的耦合器设计方法设计了一种具有超短耦合长度的非对称双芯PCF宽带定向耦合器,在1.31~1.55 μm范围内(带宽为240 nm)实现了50%±5%的耦合比。
2 双芯耦合原理与光纤结构仿真
2.1 双芯耦合原理
在双芯光纤中,每一个纤芯区域都可以被认为是一个独立的光波导,每个纤芯内传导的光场能量都会受到另一个纤芯的影响,并随着耦合区域长度的增加而发生周期性变化。根据耦合理论,对于两个平行的无损耗光波导而言,其内部的光场能量可以分别表示为
式中:I1为直通臂内的光场能量;I2为耦合臂内的光场能量;κ为波导间的耦合系数;δ为有效耦合系数;L为相互作用长度;β1为直通臂的传播常数;β2为耦合臂的传播常数。从
当两个波导及其附近的空间结构或材质不一致,即β1≠β2时,有效耦合系数将大于耦合系数,这种情况下将不存在特定相互作用长度使得入射光场能量完全进入到耦合臂中。此时,通过改变光纤结构参数,就可以在不改变相互作用长度的情况下调节有效耦合系数,进而以较短的相互作用长度在目标波长区域的中心附近获得期望的耦合比。
2.2 光纤结构仿真
相比于普通双芯光纤,双芯PCF具有更丰富的结构参数,包括孔间距、纤芯折射率差、空气孔直径、纤芯直径、空气孔对称度和中央空气孔直径等。通过改变这些结构参数,可以实现对纤芯间有效耦合系数的多路径调节,获得更精确的调节结果。本文拟分析的双芯PCF的横截面结构如
采用光束传播法对耦合器结构在不同光纤结构参数下的耦合特性进行仿真,以得到结构中光场能量随传播距离的变化情况。以初始结构参数为例,设光源波长为1550 nm,相互作用长度为1×104 μm,直通臂和耦合臂中光场能量的变化如

图 2. 初始结构参数下,耦合能量随相互作用长度的变化
Fig. 2. Variation of coupling energy with interaction length for initial structural parameters
由双芯耦合理论可知,对于确定的光纤结构,直通臂和耦合臂中的光场能量变化曲线(
式中:L和I
3 分析与讨论
为充分讨论双芯PCF中各结构参数对纤芯间耦合效率的影响,分别对孔间距、纤芯折射率差、中央空气孔直径比例、纤芯直径比例、空气孔直径比例和空气孔对称度进行参数扫描,以得到不同结构参数下的波导间耦合系数、有效耦合系数以及纤芯传播常数差。各结构参数的扫描范围如
表 1. 双芯PCF结构参数扫描范围
Table 1. Scanning range of dual-core PCF structural parameters
|
由仿真结果求得的不同结构参数下的κ和δ如
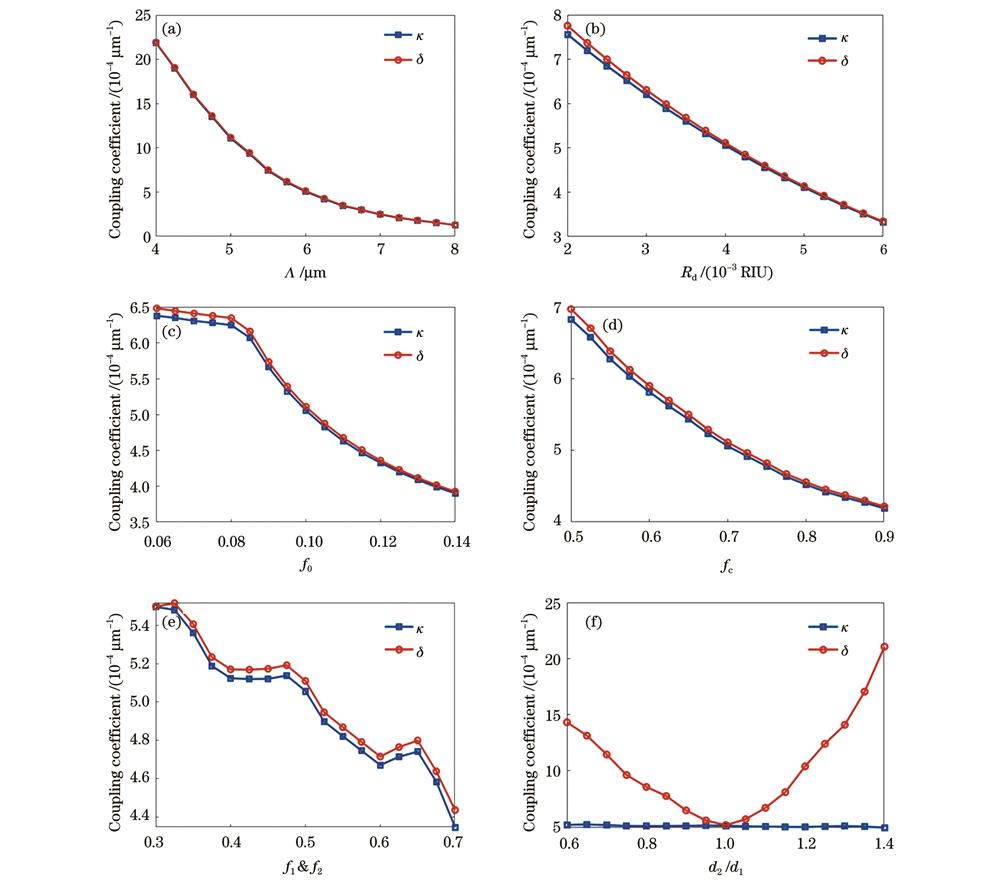
图 3. 不同结构参数时κ和δ的变化曲线。(a)孔间距;(b)纤芯折射率差;(c)中央空气孔直径比例;(d)纤芯直径比例;(e)空气孔直径比例;(f)空气孔对称度
Fig. 3. Variation curves of κ and δ for different structural parameters. (a) Aperture spacing; (b) refractive index difference of fiber core; (c) central air aperture diameter ratio; (d) fiber core diameter ratio; (e) air aperture diameter ratio; (f) air aperture symmetry
为比较不同结构参数对δ的调制效果,将
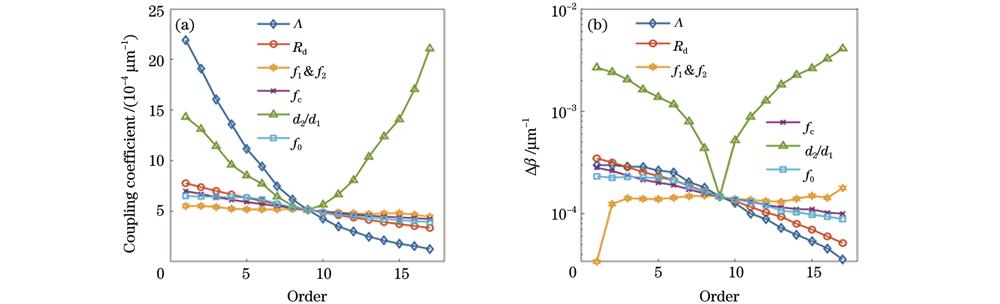
图 4. 不同结构参数时δ和Δβ的变化曲线。(a)δ;(b)Δβ
Fig. 4. Variation curves of δ and Δβ for different structural parameters. (a) δ; (b) Δβ
得到κ和δ后,可以进一步得到κ2/δ2,如
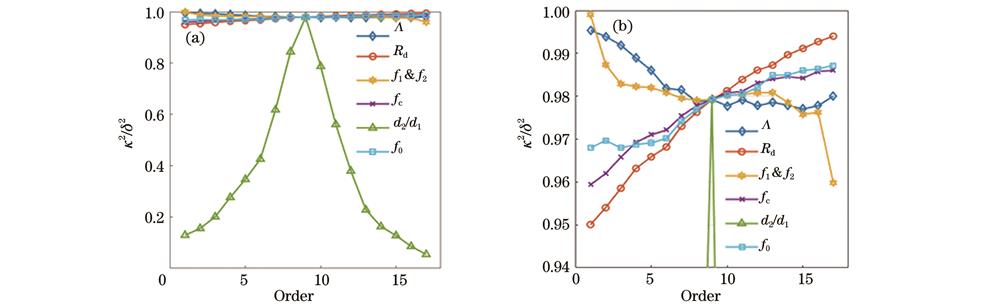
图 5. 不同结构参数时κ2/δ2的变化曲线。(a)全局图;(b)局部放大图
Fig. 5. Variation curves of κ2/δ2 for different structural parameters. (a) Global view; (b) local zoomed-in view
将不同结构参数下计算得到的κ和δ分别代入
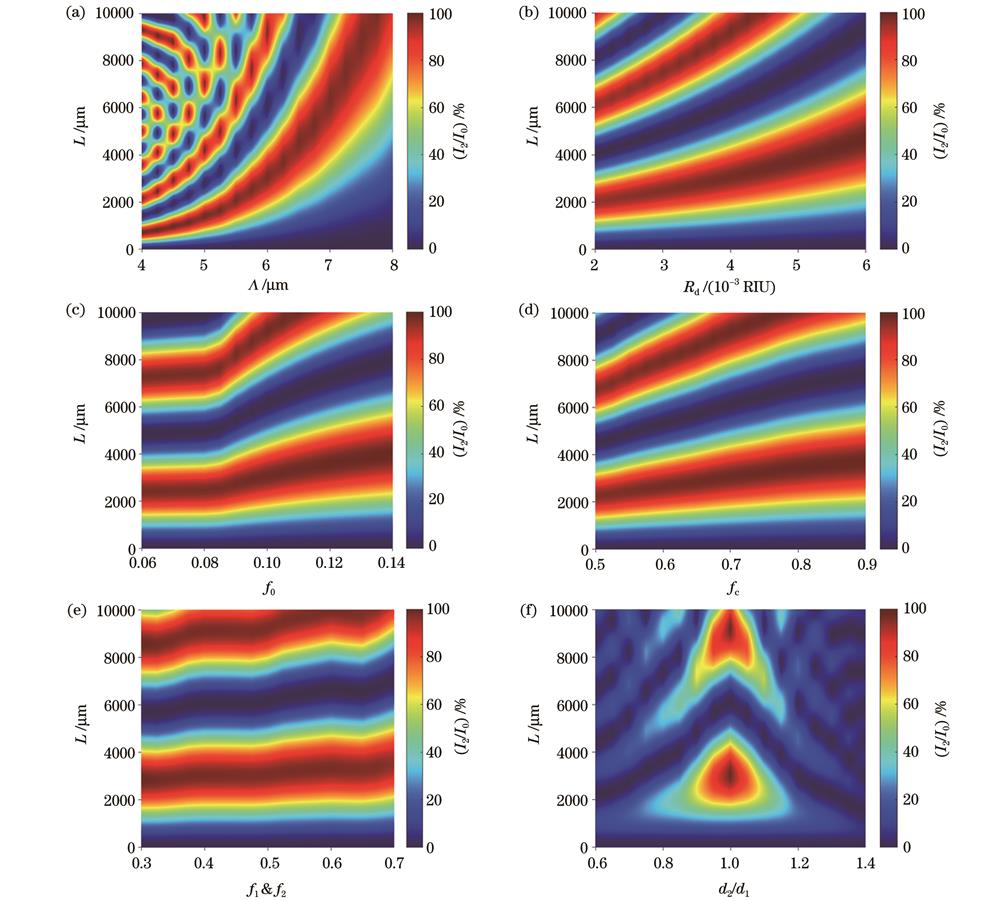
图 6. 不同结构参数下,耦合比随纤芯相互作用长度的变化。(a)孔间距;(b)纤芯折射率差;(c)中央空气孔直径比例;(d)纤芯直径比例;(e)空气孔直径比例;(f)空气孔对称度
Fig. 6. Variation of coupling ratio with core interaction length for different structural parameters. (a) Aperture spacing; (b) core refractive index difference; (c) central air pore diameter ratio; (d) core diameter ratio; (e) air pore diameter ratio; (f) air pore symmetry
为了验证入射光波长改变时所得出的结论是否仍能成立,将入射光波长改为1310 nm后,重复上述实验,得到不同结构参数下耦合臂的耦合比随纤芯相互作用长度的变化,结果如

图 7. 当入射光波长为1310 nm时,不同结构参数下耦合比随纤芯相互作用长度的变化。(a)孔间距;(b)纤芯折射率差;(c)中央空气孔直径比例;(d)纤芯直径比例;(e)空气孔直径比例;(f)空气孔对称度
Fig. 7. Variation of coupling ratio with core interaction length for different structural parameters at an incident light wavelength of 1310 nm. (a) Aperture spacing; (b) core refractive index difference; (c) central air pore diameter ratio; (d) core diameter ratio; (e) air pore diameter ratio; (f) air pore symmetry
至此,本文已经充分讨论了双芯PCF光纤中,结构参数对纤芯间耦合效率的影响。根据以上分析结果:当设计基于双芯PCF的耦合器时,增加孔间距可以在基本不改变最大耦合比的情况下快速增加耦合区域长度;调节空气孔对称度既可以实现对耦合区域长度的调节,又可以快速调节结构所获得的最大耦合比;当纤芯折射率差、中央空气孔直径比例和纤芯直径比例增大时,可以略微增加耦合区域长度以及最大耦合比;当其他参数固定且空气孔直径比例在0.4~0.6范围内时,改变空气孔直径比例基本不会对耦合区域长度以及最大耦合比产生影响。
由上述分析可知:改变孔间距和空气孔对称度,可以实现对耦合区域长度的粗调;改变空气孔对称度,可以实现对最大耦合比的粗调;改变纤芯折射率差、中央空气孔直径比例和纤芯直径比例,可以实现对耦合区域长度以及最大耦合比的微调。因此,在设计基于双芯PCF的耦合器时,可以有目的地改变光纤结构以获得期望的耦合器参数。
为验证上述理论,设计了一个包含1310 nm和1550 nm的50∶50宽带耦合器,耦合比为±5%。耦合器的初始结构参数为:Λ=6 μm,Rd=0.004,d0=0.5 μm,d1=2.2 μm,d2=2.2 μm,dc=5 μm,L=3000 μm。根据前面提出的耦合器设计理论,选择通过改变该耦合器中d2的值来调节空气孔对称度以粗调耦合区域长度和最大耦合比,并通过改变d0的值实现对耦合区域长度以及最大耦合比的微调。
为了初步确定使耦合器满足设计要求的d0、d2以及L取值的大致范围,首先以较大的参数扫描间隔进行仿真并计算了耦合器在不同结构参数下的耦合比,统计了满足耦合比要求的带宽宽度以及是否包含1310 nm与1550 nm,统计结果如
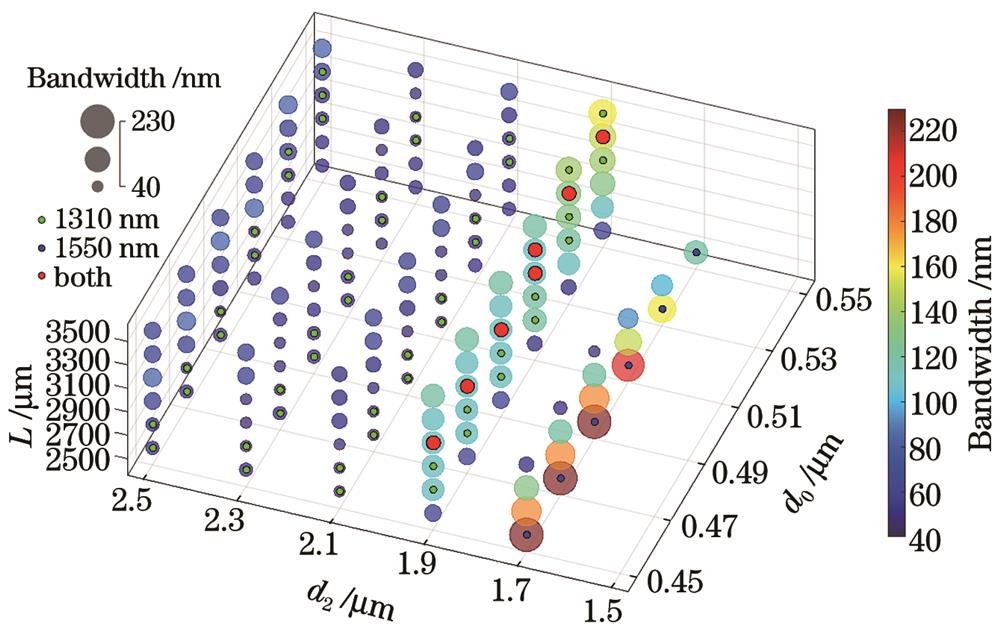
图 8. 不同d0、d2以及L下耦合器的宽带特性
Fig. 8. Broadband characteristics of the coupler for different d0, d2, and L
然后,根据缩小后的参数范围进行更精细的参数扫描仿真,发现当L=3000 μm、d2=1.8 μm、d0=0.51 μm时,所设计结构在满足设计需求的同时,带宽值最大,为240 nm。此时,直通臂与耦合臂的耦合比随波长的变化如
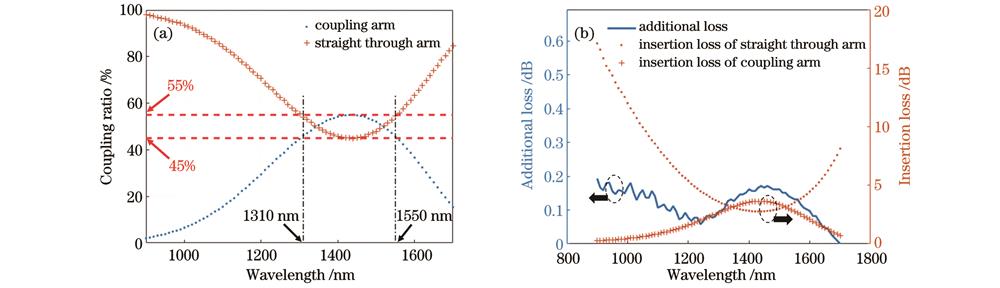
图 9. 当L=3000 μm、d2=1.8 μm且d0=0.51 μm时,直通臂与耦合臂的耦合比变化,以及插入损耗与附加损耗。(a)耦合比变化;(b)插入损耗与附加损耗
Fig. 9. Variation of coupling ratio and insertion loss and additional loss of straight through arm and coupling arm when L=3000 μm, d2=1.8 μm, and d0=0.51 μm. (a) Variation of coupling ratio; (b) insertion loss and additional loss
4 结论
采用光束传播法对双芯PCF中孔间距、纤芯折射率差、中央空气孔直径比例、纤芯直径比例、空气孔直径比例和空气孔对称度等参数对纤芯间耦合效率的影响进行分析。首先,根据双芯耦合原理,使用最小二乘法对波导间的耦合系数等参数进行估计,明确双芯PCF中各个结构参数对耦合比及耦合区长度的调节作用。通过对参数设计的具体规律进行较为详尽的总结与归纳,提出一种基于双芯PCF的耦合器性能的粗调与微调设计方法。然后,根据所提出的设计方法,设计了一种非对称双芯PCF宽带定向耦合器,分别通过调节空气孔对称度和中央空气孔直径,对所设计耦合结构的耦合比及耦合区长度进行粗调与微调,最终使该耦合器在1.31~1.55 μm范围内实现了50%±5%的耦合比,带宽为240 nm,并具有3 mm的超短耦合长度。本研究成果可以为光纤宽带定向耦合器的高效设计提供有意义的参考。
[1] 汪静丽, 刘海广, 张跃腾, 等. 分光比可设计的偏振无关光功分器研究[J]. 激光与光电子学进展, 2023, 60(17): 1723001.
[2] 周梦薇, 任偲源, 朱益清, 等. 熔锥型宽带光纤耦合器的研究[J]. 激光技术, 2019, 43(6): 757-762.
[3] 胡启航. 基于双芯光子晶体光纤的多模式选择性耦合转换器研究[D]. 北京: 北京邮电大学, 2019.
HuQ H. Research on multi-mode selective coupled converter based on two-core photonic crystal fiber[D]. Beijing: Beijing University of Post and Telecommunications, 2019.
[4] 陈曼雅, 冯素春, 任国斌. 双芯光纤的应用及研究进展[J]. 激光与红外, 2013, 43(6): 604-610.
Chen M Y, Feng S C, Ren G B. Development and application of dual-core fiber[J]. Laser & Infrared, 2013, 43(6): 604-610.
[5] Li J H, Wang J Y, Wang R, et al. A novel polarization splitter based on dual-core hybrid photonic crystal fibers[J]. Optics & Laser Technology, 2011, 43(4): 795-800.
[6] Lou S Q, Tang Z W, Wang L W. Design and optimization of broadband and polarization-insensitive dual-core photonic crystal fiber coupler[J]. Applied Optics, 2011, 50(14): 2016-2023.
[7] 汪徐德, 周正, 罗爱平, 等. 波分复用双芯光子晶体光纤耦合器的设计[J]. 激光与红外, 2011, 41(8): 875-879.
Wang X D, Zhou Z, Luo A P, et al. Design of twin core photonic crystal fiber coupler using WDM[J]. Laser & Infrared, 2011, 41(8): 875-879.
[8] 王立文, 娄淑琴, 陈卫国, 等. 用于宽带偏振无关耦合器的双芯光子晶体光纤[J]. 中国激光, 2012, 39(6): 0605008.
[9] Liu S, Li S G, Du Y. Analysis of the characteristics of the polarization splitter based on tellurite glass dual-core photonic crystal fiber[J]. Optics & Laser Technology, 2012, 44(6): 1813-1817.
[10] Sun B, Chen M Y, Zhang Y K, et al. Polarization-dependent coupling characteristics of metal-wire filled dual-core photonic crystal fiber[J]. Optical and Quantum Electronics, 2015, 47(2): 441-451.
[11] Liu Q, Li S G, Li J S, et al. Ultrashort and high-sensitivity refractive index sensor based on dual-core photonic crystal fiber[J]. Optical Engineering, 2017, 56(3): 037107.
[12] Wang H Y, Yan X, Li S G, et al. Ultra-short polarization beam splitter based on dual core photonic crystal fiber[J]. Journal of Modern Optics, 2017, 64(5): 445-450.
[13] Xu Q, Zhao Y, Xia H P, et al. Ultrashort polarization splitter based on dual-core photonic crystal fibers with gold wire[J]. Optical Engineering, 2018, 57(4): 046104.
[14] Dou C, Jing X L, Li S G, et al. A compact and low-loss polarization splitter based on dual-core photonic crystal fiber[J]. Optical and Quantum Electronics, 2018, 50(6): 255.
[15] Anbazhagan L, Ganesan G. Polarization-independent wavelength splitter based on silicon nanowire embedded dual-core pentagonal lattice photonic crystal fiber for optical communication systems[J]. Optical Engineering, 2021, 60(4): 047103.
[16] 黄旭, 骆兴芳, 左翔宇, 等. 双芯太赫兹光纤定向耦合器[J]. 光学学报, 2022, 42(2): 0206006.
[17] Hagras E A A, Hameed M F O, Obayya S S A. Compact dual-core liquid crystal photonic crystal fiber polarization splitter for terahertz applications[J]. Optik, 2022, 265: 169396.
[18] 杨世泰, 陈大伟, 段劭琛, 等. 多芯光纤对角芯反射耦合器[J]. 光学学报, 2023, 43(13): 1306001.
[19] Ding Z W, Zou N M, Zhang C, et al. Self-optimized vibration localization based on distributed acoustic sensing and existing underground optical cables[J]. Journal of Lightwave Technology, 2022, 40(3): 844-854.
Article Outline
王胜意, 丁哲文, 郑祥亮, 赵春柳. 基于双芯光子晶体光纤的耦合器设计方法[J]. 光学学报, 2024, 44(5): 0506004. Shengyi Wang, Zhewen Ding, Xiangliang Zheng, Chunliu Zhao. Design Method of Coupler Based on Dual-Core Photonic Crystal Fibers[J]. Acta Optica Sinica, 2024, 44(5): 0506004.