Bright/dark switchable mode-locked fiber laser based on alcohol
1. Introduction
Ultrafast fiber lasers have attracted considerable attention owing to their versatile applications in the fields of optical communication[1], optical sensing[2], biomedical research[3], material processing[4], and optical continuum generation[5]. Mode locking is an important method to obtain ultrashort pulses, and generally, active and passive techniques are typically employed for obtaining the mode-locking operations. Taking the advantages of no requirement of additional drives, compactness, and low cost, the passive mode-locking technique that involves inserting a nonlinear optical device called a saturable absorber (SA) into the laser cavity has been investigated extensively[6,7]. Over the past couple of decades, many varieties of SA materials have been reported to generate ultrashort laser pulses, including semiconductor saturable absorber mirrors (SESAMs)[8], graphene[9], carbon nanotubes (CNTs)[10], black phosphorus (BP)[11], transition metal chalcogenides (TMDs)[12], and so on[13]. Unfortunately, the majority of all these SAs exhibit some drawbacks, affecting their further development. The operation bandwidth of SESAMs is usually limited to tens of nanometers, which is severely constrained by its inherent energy bandgap; meanwhile, the fabrication procedure of SESAMs is complicated and expensive. CNTs and graphene have also been widely employed as SAs due to their excellent physical and chemical properties. However, the modulation depth per layer of graphene is typically less than 1%, which makes the saturating intensity low, so that the obtained laser pulse energy and duration are both limited. In terms of the laser using CNT as an SA, the operation wavelength is largely impacted by the different diameters of the CNTs adopted. BP has the defects of a relatively lower damage threshold. TMDs are another type of transition metals with the ultrahigh multi-electron transfer and high electrical conductivity (e.g., , , , and ). However, chemical vapor deposition (CVD) or/and pulsed laser deposition (PLD) are inevitably adopted in the manufacturing process[14,15], making the fabrication really complicated.
More recently, a kind of organic liquid SA, alcohol, has attracted particular interest for generating ultrashort pulses, since it possesses an ultrafast nonlinear response and broad operation bandwidth. Additionally, SAs still have excellent optical qualities such as high damage threshold, simplicity of fabrication, low cost, and thermal stability. Last but not least, the modulation depth can be adjusted to achieve the desired ultrashort pulses by varying the amount of alcohol. To date, several schemes adopting alcohol as an SA for achieving ultrashort pulses have been demonstrated in fiber lasers. Wang et al. reported the -switching and mode-locking operation employing alcohol–SA in an anomalous dispersion cavity successively[16,17], where the pulsed laser was emitted at the communication band. Then, the pulsed laser working at the 2 µm waveband was investigated in a thulium-doped fiber laser using alcohol as the SA[18]. In order to pursue better pulse performance and produce more kinds of optical solitons, the laser using alcohol and alcohol-like organic liquid as the SA to obtain the desired pulse has been reported by many researchers[19–24]. These results show that shorter pulse lasers can be generated by using organic liquid as the SA. However, most of the research focused on the generation of bright soliton or dark soliton, and alcohol-assisted mode-locked fiber laser delivered with a switchable pulse has not yet been reported so far.
Here, we demonstrate a switchable passively mode-locked erbium-doped fiber (EDF) laser in an anomalous dispersion region. As a high nonlinear medium, the alcohol–SA is used for mode locking and stabilizing the pulse oscillation in the laser cavity. Based on the nonlinear polarization evolution (NPE) effect, different operation states, including bright pulse, bright/dark soliton pair, and dark pulse can be conveniently achieved in the communication band by precisely adjusting the pump strength and polarization state. The generated pulsed laser is relative stable, operating at the fundamental frequency state. The obtained results indicate that the alcohol–SA could be indeed a good candidate for highly nonlinear optical material for potential application in ultrafast photonics.
2. Experimental Setup
The experimental setup of the proposed mode-locked fiber laser is illustrated in Fig. 1. As shown in Fig. 1, the laser cavity consists of a 976 nm laser diode (LD), a 980 nm/1550 nm wavelength-division multiplexer (WDM), a piece of 2.5 m EDF, a polarization controller (PC), a polarization-independent isolator (PI-ISO), a 10/90 optical coupler (OC), and an SA made with a certain amount of alcohol. The LD with the maximum power of 600 mW is used as the pump source to pump the EDF through the fused WDM. The EDF with the group velocity dispersion (GVD) of −26 ps/(km nm) at 1550 nm and 80 dB/m absorption ratio at 1530 nm contributes to the gain media. The PC is utilized to adjust the polarization state and intracavity loss for realizing the soliton operation switching. The PI-ISO is inserted into the cavity to force the laser to have unidirectional circulation in the cavity. The alcohol–SA is employed as a mode-locking element, which is formed by sandwiching a certain amount of alcohol between two optical ferrules inside a connector. After the sandwiched structure is finished, the alcohol–SA was immersed in a sealed container full of alcohol to avoid evaporation.
Notably, detected by a typical balanced two-detector measurement system (same as that in Ref. [16]), the modulation depth of the adopted SA is measured to be 6.4%, which is present in Fig. 2. The OC is used as an output port that extracts 10% power from the generated laser for measurements. All other fibers are standard single-mode fibers (SMFs) with a total length of 7.6 m and a dispersion parameter of 17 ps/(km nm) at 1550 nm. So the total length of the cavity is 10.1 m, and the net cavity dispersion is estimated as .
With the dynamic balance among dispersion, nonlinearity, gain, and loss, a stable mode-locking operation with different working states can be realized. The optical performance of the output pulsed laser was measured by an optical spectrum analyzer (Yokogawa AQ6370D) with spectral resolution of 0.02 nm. The time-domain characteristics were monitored by an oscilloscope (RTO 2022, 2 GHz) combined with a high-speed 10 GHz bandwidth photodetector (Newport 818-BB-51F) and a power meter, respectively. The frequency-domain characteristics were detected by the same photodetector and measured by a radio-frequency (RF) spectrum analyzer (Keysight N9000B).
3. Results and Discussions
3.1. Bright soliton emission
In the proposed laser, by properly adjusting the polarization state and increasing the pump power to the threshold, three mode-locking states can be obtained, including bright soliton emission, bright/dark pair soliton emission, and dark soliton emission, respectively.
First, the output performance of the generated bright pulse operation under the pump power of 180 mW is demonstrated and discussed, as shown in Fig. 3. As depicted in Fig. 3(a), faint Kelly sidebands are distributed at both sides of the spectrum, which is formed due to interaction between the dispersive wave and the bright soliton pulse[25]. The appearance of the sidebands indicates that the observed soliton is indeed a conventional soliton. The central wavelength locates at 1563.4 nm and the 3 dB bandwidth is 0.36 nm. Figure 3(b) illustrates the time-domain characteristics of the bright pulse. A uniform pulse train with a temporal period of about 49.8 ns is given, which is consistent with the cavity round-trip time. Due to the lack of autocorrelation instruments in the laboratory, we present the zoom-in single-pulse profile of the bright-soliton pulse trains to characterize its pulse width performance, which is shown in Fig. 3(b). According to the Fourier transform limit, if a profile was assumed for calculation, the pulse duration would be estimated greater than 7.5 fs. In addition, to investigate the operation stability of the mode-locking pulse, we measured its RF spectrum. As shown in Fig. 3(c), the fundamental pulse repetition rate is 20.05 MHz with a resolution bandwidth (RBW) of 1 kHz, which agrees well with the temporal period of 49.8 ns in Fig. 3(a). The signal-to-noise ratio (SNR) is . The considerably high SNR indicates that the obtained pulsed laser is relatively stable.
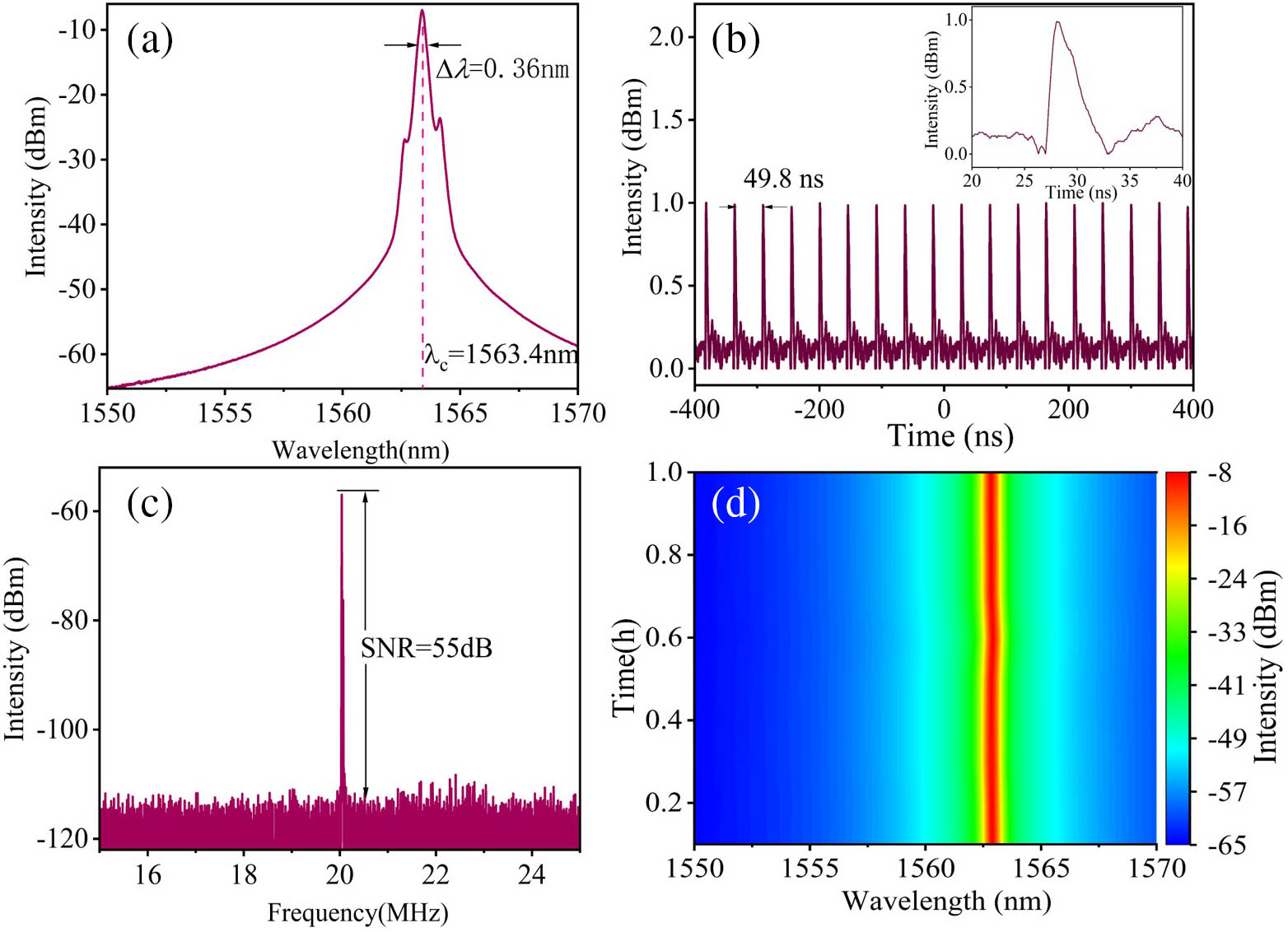
Fig. 3. Typical characteristics of the bright-pulse operation at the pump power of 180 mW. (a) Output optical spectrum; (b) corresponding pulse train in different time ranges (inset, the zoom-in single-pulse profile); (c) corresponding RF spectrum with 1 kHz RBW; (d) stability record of the output spectrum for 1 h.
Notably, the pump power is hold at 180 mW; meanwhile, the output average power of the fiber laser is 6.94 mW. The corresponding single-pulse energy is on the order of nanojoule, which is comparable to the traditional bright soliton. In particular, the pulse tends to split under higher pump power conditions[26]. In order to further prove the stability of the laser, we place the laser in the laboratory environment without external intervention control and record the corresponding spectral evolution every 5 min for 1 h. As shown in Fig. 3(d), there is no obvious alteration of the recorded spectra, confirming the good stability of the bright-soliton mode-locking operation.
3.2. Bright/dark pair soliton emission
Additionally, by properly adjusting the PC to another state, the bright soliton and dark soliton, combined as a unit, can be observed simultaneously, which means that the bright/dark soliton pair is produced. The formation can be explained by the fact that when two solitons are spaced so close to each other, their soliton profiles will overlap strongly. Under a certain soliton phase difference, a strong binding force is induced between them by direct soliton interaction[27,28]; then they coexist and co-propagate stably in the cavity, manifesting that the bright/dark soliton pair is generated. Here, we focus the discussion on the optical characteristics of the bright/dark soliton pair at the same pump power of 180 mW. As shown in Fig. 4(a), the central wavelength is 1563.18 nm, with a spectral bandwidth of 0.31 nm. The spectral width is slightly narrow owing to the combination of spectral filtering effect, the nonlinear effect of TDF, and loss in the cavity. Notably, the Kelly sidebands of the optical spectrum disappear, which may be attributed to the NPE[29]. It can be interpreted that different polarization states means various intensity gain and/or loss; based on the complexity of the gain modulation and NPE effect, the sidebands vanish. The elimination of Kelly sidebands can not only reduce the waste of energy and facilitate amplification, but also has a significant impact on the interaction between the pulses and the mode-locked states. As shown in Fig. 4(b), the period of the bright/dark soliton pair pulse train is 49.8 ns, corresponding to the cavity length of 10.1 m. The single-pulse profile of the bright/dark soliton pair is also present as the inset. And we notice that the pulse intensity and pulse duration of the bright soliton and the dark soliton are both different, which may be caused by the combined influence of the nonlinearity of the alcohol–SA and the total dispersion of the laser cavity. Likewise, we did not measure the autocorrelation trace of the soliton pair either. In order to testify to the stability of the bright/dark soliton pair operation, the RF spectrum is measured, as exhibited in Fig. 4(c). The fundamental peak is situated at the cavity repetition rate of 20.05 MHz, with a higher SNR of 65 dB, indicating good stability of the mode-locking operation of the bright/dark soliton pair. Similarly, the stability of the bright/dark soliton pair emission of the laser was also verified by recording its spectral variation for 1 h. As presented in Fig. 4(d), the spectra recorded are shown without any noticeable fluctuation, indicating the stability of soliton pair operation further. To the best of our knowledge, this is the first demonstration of the bright/dark pair soliton in a fiber laser based on the alcohol–SA.

Fig. 4. Typical characteristics of the bright/dark soliton pair operation at the pump power of 180 mW. (a) Output optical spectrum; (b) corresponding pulse train in different time ranges (inset, the zoom-in single pulse profile); (c) corresponding RF spectrum with 1 kHz RBW; (d) stability record of the output spectrum for 1 h.
3.3. Dark soliton emission
Furthermore, dark pulse can also be achieved by suitably rotating the PC to a particular condition. The formation mechanism of the dark pulse may be similar to that in Ref. [21]. That is to say, taking the combination of fiber dispersion, intracavity birefringence, the polarization dependent loss of PC and alcohol–SA into consideration, a dark pulse could be achieved by properly adjusting the pump strength and the polarization state[30,31]. Figure 5(a) displays the optical spectrum of the dark pulse emission of the proposed laser. Clearly, there are no sidebands emerging because of the polarization effect. The central wavelength and spectral bandwidth are 1563.56 and 0.48 nm, respectively. Figure 5(b) exhibits the pulse train of the dark soliton operation with the same period. In addition, a slight nonuniformity is observed in the measured dark-pulse intensity, which may be generated by the limit of the electronic detection. The inset of Fig. 5(b) shows the single-pulse profile of the dark-pulse train. Figure 5(c) illustrates that the fundamental repetition is 20.05 MHz corresponding to the total cavity length of 10.1 m. The SNR is up to 60 dB, manifesting that the achieved dark soliton operation is relatively stable. Furthermore, we provide the results of the recorded spectral variation under the same conditions. As plotted in Fig. 5(d), no significant change appears in the whole recording process, indicating the good mode-locking stability of the laser.
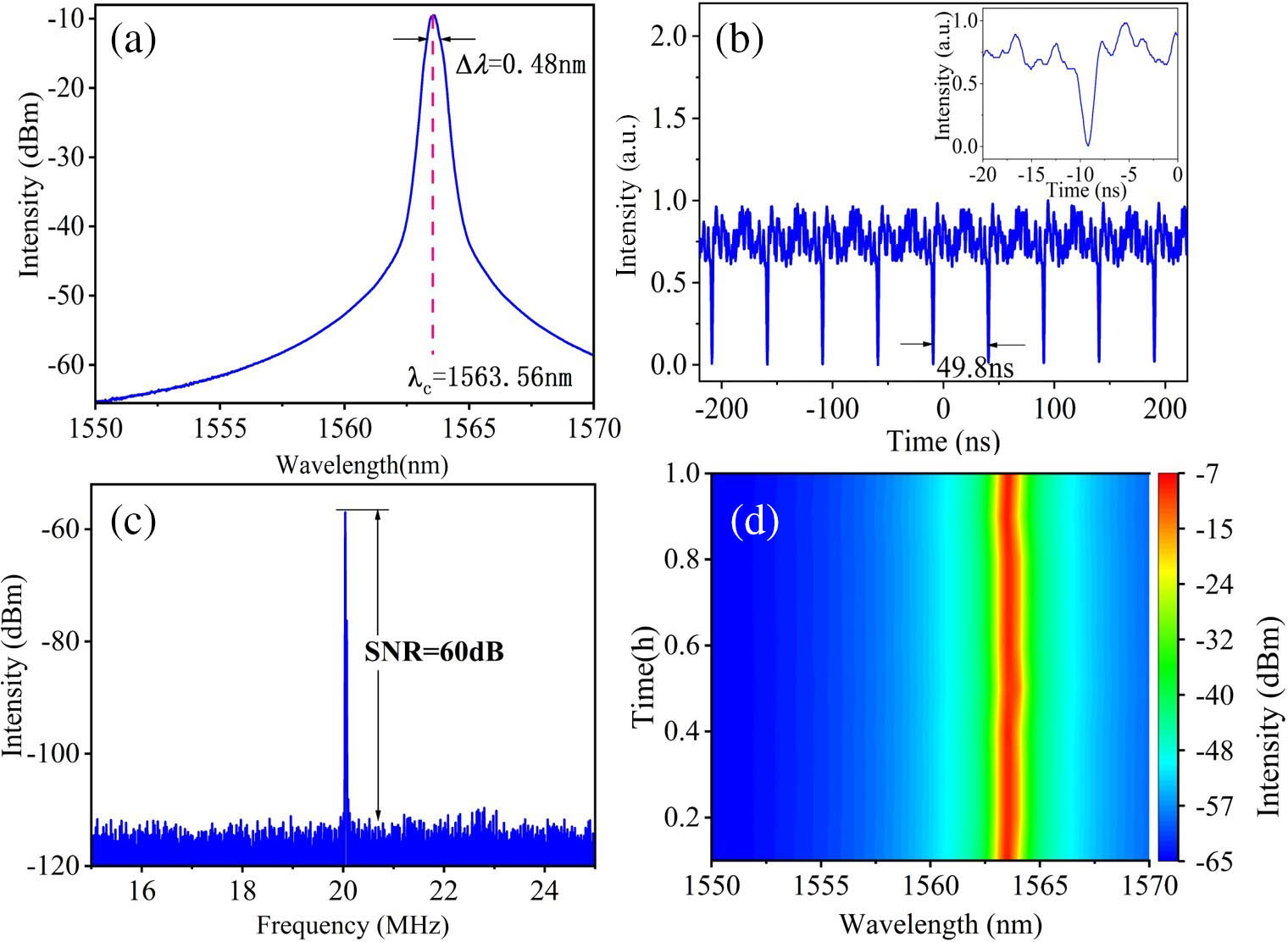
Fig. 5. Typical characteristics of the dark-pulse operation at the pump power of 180 mW. (a) Output optical spectrum; (b) corresponding pulse train in different time ranges (inset, the zoom-in single-pulse profile); (c) corresponding RF spectrum with 1 kHz RBW; (d) stability record of the output spectrum.
Finally, to further confirm the mode-locking function of alcohol in this soliton laser, we test the operation characteristic without inserting the alcohol–SA. In this case, no mode-locking operation was observed, no matter how we adjusted the pump strength and the cavity polarization state in a full range, which further verifies the effectiveness of the alcohol–SA as a mode locker.
4. Conclusion
In the paper, a bright/dark switchable passively mode-locked fiber laser in the anomalous dispersion regime is reported and demonstrated. Using alcohol as the SA in the cavity, the laser operation can be flexibly switched among a bright soliton, a bright/dark soliton pair, and a dark soliton by suitably tuning the polarization state. The laser operates in the communication band, with the fundamental frequency of 20.05 MHz. Our results show that alcohol can serve as an excellent SA for bright and dark pulse generation and also has broad application prospects in the field of nonlinear optics.
Article Outline
Wenyan Zhang, Huijie Jiang, Kun Yang, Nannan Liu, Lijie Geng, Yunqi Hao, Tianhao Xian, Li Zhan. Bright/dark switchable mode-locked fiber laser based on alcohol[J]. Chinese Optics Letters, 2024, 22(3): 031403.