大面积图案化电场增强薄膜的设计及制备研究【增强内容出版】
Currently, there are various technologies for controlling the electric field using the micro-nano structure of noble metal films, including electron beam lithography (EBL) and focused ion beam (FIB) etching. Most of these processing methods are complicated, with limited processing areas and high costs. These limitations significantly restrict the development of photoelectric functional films with electric field enhancement characteristics. According to current theories, the enhancement mechanism of surface-enhanced Raman scattering (SERS) technology can be divided into electromagnetic enhancement mechanism (EM) and chemical enhancement mechanism (CM). Compared to CM, EM generally exhibits stronger increases in the Raman scattering signals. Therefore, it is important to develop EM-based SERS substrates. The enhancement of the electromagnetic field mainly originates from the local surface plasmon resonance (LSPR) of the metal nanoparticles, which is independent of the adsorbed molecules and is an inherent property of metal nanoparticles. Therefore, classical electronic dynamics methods such as the finite difference time domain (FDTD) method can be used to describe the electromagnetic field. The purpose of this study is to develop a large-area, low-cost, and reproducible SERS substrate using FDTD simulations combined with mechanical grating ruling and electron beam evaporation deposition.
In this study, Silver (Ag) films with different blazed grating structures are simulated using FDTD method. The LSPR of the Ag films with blazed grating structures is significantly enhanced by adjusting the period. Under excitation at 633 nm, we find that Ag films with a grating period of 1/1200 mm generate a significant LSPR effect. Large-area electric-field-enhanced Ag grating films that can be produced in batches are realized using a mechanical grating ruling process and electron beam evaporation deposition. We successfully apply this electric field-enhanced Ag film to SERS to detect methylene blue dye. The Ag grating films significantly enhance the SERS intensity, and the test results show good uniformity and reproducibility of the substrate, consistent with the FDTD simulation results.
As shown in Fig. 1, FDTD software is used to design and simulate two types of blazed grating film structures, and the electric field intensity and absorption spectra of the Ag films are compared. The results of the FDTD simulation (Fig. 2) show that compared to the Ag film with a grating structure, the pure Ag film exhibits lower electric field intensity and light absorption. Compared with other two types of structures, the Ag films on Grating 1 exhibit the strongest electric field intensity and absorbance in the local area, indicating that the Ag films with a grating structure exhibit a stronger LSPR effect at 633 nm. Blazed Grating 1 and Grating 2 structures are prepared using a mechanical scribing process and the required diffraction gratings are produced in batches through grating replication. Finally, the processed gratings are coated with Ag films through electron beam evaporation deposition to obtain Ag grating films with the required electric field enhancement effect. The prepared Ag films with grating structures are characterized using X-ray diffraction (XRD) (Fig. 4), atomic force microscopy, and optical microscopy (Fig. 5). The microscopic images and XRD results illustrate that the large-area Ag films with a grating structure are successfully prepared. The surface uniformity of the prepared gratings is good with equal spacing between the lines. A Raman spectrometer is used to verify the electric-field enhancement ability of films on Grating 1. As shown in Fig. 6(a), the Ag thin films with a grating structure produce more 'hot spots,' which significantly enhances the local electromagnetic field on the surfaces of the grating samples, thus substantially improving the Raman scattering signal of methylene blue. Figures. 6(b)‒(d) show that the corresponding relative standard deviation (RSD) values of the characteristic peaks at 1623 cm-1 and 1394 cm-1 for methylene blue in different sites of the Grating 1 sample are less than 17%, confirming the good reproducibility of the SERS substrate.
In this study, a simple and low-cost technology for fabricating large-area patterned electric-field-enhanced Ag films is proposed. First, Ag films with a blazed grating structure is simulated using the FDTD method, and a film structure with strong electric field enhancement at 633 nm wavelength is obtained by adjusting the period, which effectively tunes the LSPR effect on the film surface. Ag thin films with corresponding structures are prepared using mechanical grating ruling and electron-beam evaporation deposition. Through verification by a Raman spectrometer, the Ag films with a blazed grating structure improve the SERS intensity, consistent with the simulation results. This electric-field-enhanced film provides an efficient substrate for the enhancement of Raman scattering signals and an idea for the actual detection of trace molecules.
1 引言
当外部入射光作用于贵金属薄膜表面时,如果其频率和波矢量与金属薄膜表面自由电子振荡频率和波矢量相匹配,就会产生电子的集体共振现象,从而出现局域表面等离子体共振(LSPR)效应[1-2]。这种集体振荡效应可使薄膜表面电场显著放大[3]。LSPR效应在生物传感[4]、荧光增强[5]、非线性光学[6]、表面增强拉曼散射(SERS)[7]等众多光电子学领域中得到广泛应用。金、银、铂等贵金属材料因介电常数为负且绝对值较大,常被设计为特定的微纳结构以形成电场增强[8]。目前,制备微纳结构金属薄膜的工艺多种多样,其中以电子束曝光(EBL)[9]和聚焦离子束刻蚀(FIB)[10]等方法为代表。这些加工方法大多较为复杂,加工面积有限,成本相对高昂,这大大限制了具备电场增强特性的光电功能薄膜的发展。因此,开发制备工艺简单、成本低廉、能够大面积生产的电场增强金属薄膜具有重要的意义。
与上述过程繁琐、价格昂贵的加工方法相比,基于机械刻划和复制技术制备衍射光栅[11]的工艺流程相对简单,生产成本也大幅降低。通过设计合适的光栅结构,可以获得具有电场增强效果的贵金属薄膜。文献[12]报道,贵金属薄膜的电场增强效果不仅取决于材料的选择,还取决于薄膜表面微结构设计。通过改变每毫米光栅划线数、光栅平面与槽面夹角、金属薄膜厚度等参数,可以高效、便捷地对不同波段电场增强金属薄膜进行研究。
本文首先通过时域有限差分(FDTD)法进行仿真,建立了银光栅薄膜模型,研究了在633 nm入射光下不同结构模型的电场增强效果。根据仿真结果,使用机械刻划工艺制备所需的大面积高精度衍射光栅母板,再通过光栅复制的方式批量生产所需的衍射光栅,最后在加工好的光栅微结构上,通过电子束蒸发镀膜法,镀上相应厚度的金属薄膜,即可得到具有电场增强效果的金属光栅薄膜。
2 FDTD仿真
表面等离子体激元是一种沿着金属表面传播的波。当入射光的偏振方向平行于入射面时,在合适的边界条件下求解麦克斯韦方程组[13],可以得到等离子体激元的传输波矢量大小(
式中:
根据Drude模型[14],当满足共振条件时,有
式中:
式中:
由
为了实现633 nm波段大面积电场增强的图案化银薄膜,使用FDTD法设计和模拟了
在仿真中设置闪耀角均为8°,周期分别为1/1200 mm与1/2400 mm。将周期为1/1200 mm与1/2400 mm的闪耀光栅分别标记为Grating 1和Grating 2。xy方向采用周期边界条件。同时,633 nm的平面光垂直入射在仿真样品的xy面上。
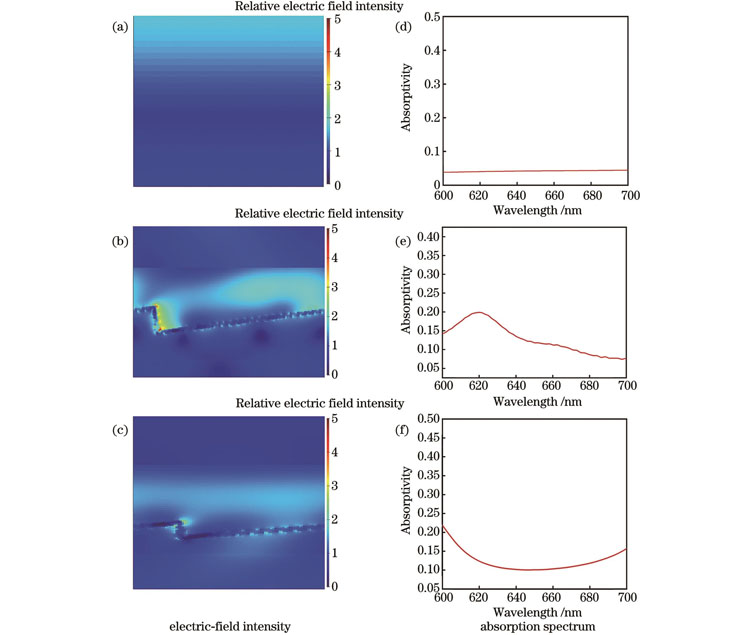
图 2. 采用FDTD法仿真获得的在633 nm入射光下的电场强度和吸收图谱。(a)(d)15 nm厚的Ag薄膜;(b)(e)Grating 1上的Ag薄膜;(c)(f)Grating 2上的Ag薄膜
Fig. 2. Electric field intensity and absorption spectra obtained by FDTD simulation at 633 nm incident light. (a)(d) 15 nm thick Ag film; (b)(e) Ag film on Grating 1; (c)(f) Ag film on Grating 2

图 3. 不同Ag薄膜在633 nm入射光下的电场强度与吸光率
Fig. 3. Electric field intensity and absorptivity of different Ag films at 633 nm incidence light
3 实验
3.1 样品制备
实验首先制备母版光栅,采用K9玻璃作为光栅的毛胚,利用真空镀膜机在表面镀上铬作为底层,然后镀铝以增加K9玻璃与铝层的黏结牢固度,最后利用光栅刻划机进行刻划,刻划完毕后利用光栅复制工艺得到闪耀角为8°、周期分别为1/1200 mm和1/2400 mm的透射式闪耀光栅。在所得光栅表面利用电子束蒸发技术分别沉积15 nm 厚的Ag薄膜。薄膜沉积时本底真空度为5×10-4 Pa,电子束电压为9.3 kV。最终周期为1/1200 mm与1/2400 mm的闪耀光栅上的Ag薄膜样品分别标记为Grating 1样品和Grating 2样品。为了方便对比,没有光栅结构的15 nm Ag薄膜也被用于对照。最后在不同样品上分别滴加1×10-4 mol/L浓度的亚甲基蓝(MB)染料以测试其SERS性能。
3.2 样品表征
采用X射线衍射仪分析薄膜样品的晶相结构,扫描角度为10°~70°。采用原子力显微镜表征样品的表面形貌。采用共聚焦拉曼显微镜表征薄膜的染料探针分子的拉曼散射光谱,其激发波长为633 nm。所有样品测试均在室温下进行。
4 结果分析
4.1 X射线衍射图谱
4.2 原子力显微镜图谱和光学显微镜图谱
4.3 SERS图谱
当染料探针分子与Ag薄膜结构的距离足够小时,LSPR效应导致的电场增强会放大拉曼散射信号,从而出现表面增强拉曼散射,即SERS效应[18]。为了进一步验证这种光栅结构上的电场增强Ag薄膜的潜在应用,我们采用拉曼光谱仪研究了纯Ag薄膜和光栅结构上的Ag薄膜的SERS效应。采用亚甲基蓝作为SERS探针分子,其浓度为1×10-4 mol/L,激发波长为633 nm。测试结果如
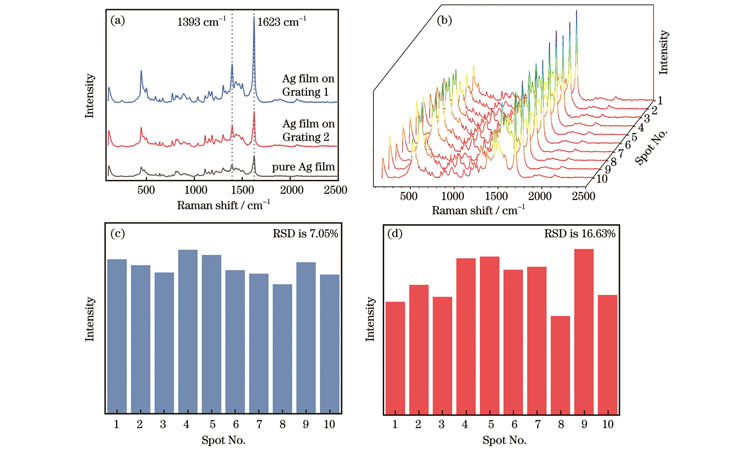
图 6. 实验结果。(a)MB在不同样品上的SERS图谱;(b)Grating 1样品上10个随机位点收集的MB分子的SERS图谱;(c)1623 cm-1和(d)1394 cm-1处特征峰的RSD值
Fig. 6. Experimental results. (a) SERS spectra of MB on different samples; (b) SERS spectra of MB molecules collected at 10 random sites on Grating 1 sample; RSD values of characteristic peaks at (c) 1623 cm-1 and (d) 1394 cm-1
5 结论
提出了一种工艺简单、成本低廉的制备大面积图案化电场增强薄膜的技术。首先通过FDTD法仿真了闪耀光栅结构上的Ag薄膜,通过调整周期在633 nm波长下获得了强烈电场增强的薄膜结构,该结构有效调谐了薄膜表面的LSPR效应。然后采用机械刻化和电子束蒸发工艺大面积制备了相应结构的Ag薄膜。在633 nm激发光下通过拉曼光谱仪验证发现,闪耀光栅结构上的Ag薄膜有助于提升SERS强度,与仿真结果一致。这种电场增强薄膜可增强拉曼散射信号,是一种高效的基底,为实际微量分子检测提供了一种思路。
[1] Ozbay E. Plasmonics: merging photonics and electronics at nanoscale dimensions[J]. Science, 2006, 311(5758): 189-193.
[2] Barnes W L, Dereux A, Ebbesen T W. Surface plasmon subwavelength optics[J]. Nature, 2003, 424(6950): 824-830.
[3] 刘钰, 张海涛, 徐抒平, 等. 折射率和金属膜厚度对表面等离子体共振场增强表面增强拉曼散射的影响研究[J]. 中国激光, 2013, 40(12): 1207001.
[4] Shrivas K, Shankar R, Dewangan K. Gold nanoparticles as a localized surface plasmon resonance based chemical sensor for on-site colorimetric detection of arsenic in water samples[J]. Sensors and Actuators B: Chemical, 2015, 220: 1376-1383.
[5] Xie F, Centeno A, Ryan M R, et al. Au nanostructures by colloidal lithography: from quenching to extensive fluorescence enhancement[J]. Journal of Materials Chemistry B, 2013, 1(4): 536-543.
[6] Kumar P, Mathpal M C, Hamad S, et al. Cu nanoclusters in ion exchanged soda-lime glass: study of SPR and nonlinear optical behavior for photonics[J]. Applied Materials Today, 2019, 15: 323-334.
[7] Zhu C H, Meng G W, Huang Q, et al. Ag nanosheet-assembled micro-hemispheres as effective SERS substrates[J]. Chemical Communications, 2011, 47(9): 2709-2711.
[8] Austin L A, MacKey M A, Dreaden E C, et al. The optical, photothermal, and facile surface chemical properties of gold and silver nanoparticles in biodiagnostics, therapy, and drug delivery[J]. Archives of Toxicology, 2014, 88(7): 1391-1417.
[9] Chen Y F. Nanofabrication by electron beam lithography and its applications: a review[J]. Microelectronic Engineering, 2015, 135: 57-72.
[10] Qu M N, Shen Y L, Wu L Y, et al. Homogenous and ultra-shallow lithium niobate etching by focused ion beam[J]. Precision Engineering, 2020, 62: 10-15.
[11] 赵翠翠, 倪争技, 张大伟, 等. 刻划光栅制造技术研究进展[J]. 激光杂志, 2010, 31(6): 1-3.
Zhao C C, Ni Z J, Zhang D W, et al. Research progress in fabrication of ruling gratings[J]. Laser Journal, 2010, 31(6): 1-3.
[12] Xia Y N, Halas N J. Shape-controlled synthesis and surface plasmonic properties of metallic nanostructures[J]. MRS Bulletin, 2005, 30(5): 338-348.
[13] Sambles J R, Bradbery G W, Yang F Z. Optical excitation of surface plasmons: an introduction[J]. Contemporary Physics, 1991, 32(3): 173-183.
[14] Johnson P B, Christy R W. Optical constants of the noble metals[J]. Physical Review B, 1972, 6(12): 4370-4379.
[15] Hong R J, Shao W, Sun W F, et al. The influence of dielectric environment on the localized surface plasmon resonance of silver-based composite thin films[J]. Optical Materials, 2018, 83: 212-219.
[16] 吴伟, 王济洲, 熊玉卿, 等. 半球形基底镀膜膜厚均匀性理论分析[J]. 真空科学与技术学报, 2014, 34(4): 320-324.
Wu W, Wang J Z, Xiong Y Q, et al. Theory analysis of vacuum evaporation of thin film with uniform thickness hemispherical substrate[J]. Chinese Journal of Vacuum Science and Technology, 2014, 34(4): 320-324.
[17] Hong R J, Shao W, Sun W F, et al. Laser irradiation induced tunable localized surface plasmon resonance of silver thin film[J]. Optical Materials, 2018, 77: 198-203.
[18] Lü Y J, Sun H L, Lian X X, et al. Surface morphology evolution behavior and SERS performance of Mo-Ag-Cu-Co films[J]. Applied Surface Science, 2022, 604: 154594.
[19] Li C Y, Huang Y Q, Lai K Q, et al. Analysis of trace methylene blue in fish muscles using ultra-sensitive surface-enhanced Raman spectroscopy[J]. Food Control, 2016, 65: 99-105.
[20] Rodriguez R D, Sheremet E, Nesterov M, et al. Aluminum and copper nanostructures for surface-enhanced Raman spectroscopy: a one-to-one comparison to silver and gold[J]. Sensors and Actuators B: Chemical, 2018, 262: 922-927.
[21] 张垒, 张霞, 柳晓钰, 等. Fe3+对植酸封端的金纳米颗粒SERS性能的影响[J]. 中国激光, 2019, 46(3): 0311006.
孙伟, 洪瑞金, 陶春先, 张大伟. 大面积图案化电场增强薄膜的设计及制备研究[J]. 中国激光, 2023, 50(23): 2303101. Wei Sun, Ruijin Hong, Chunxian Tao, Dawei Zhang. Design and Preparation of Large-Area Patterned Electric Field Enhanced Films[J]. Chinese Journal of Lasers, 2023, 50(23): 2303101.