Objective visual performance evaluation with visual evoked potential measurements based on an adaptive optics system
Download: 819次
Visual performance tests [visual acuity (VA) and contrast sensitivity (CS), etc.] provide important supplementary data for diagnosing visual pathology or other pathological involvement of the visual pathway. However, it is well known that visual performance is governed by optical, retinal, and neural factors. At present, several successful attempts have been directed at bypassing the optical limits. For example, the history can be traced back to when Campbell and Green[1] measured the contrast sensitivity function (CSF) without optical limits using interference fringes formed on the retina. Aside from interference fringes, a powerful tool to bypass the optical limits was through adaptive optics (AO). Since Liang
However, all of the studies mentioned above were the optical limits to subjective visual performance. The subjective test is measured by the psychophysiological method. It always needs subjects to judge by a perceived pattern stimulus. Unfortunately, the subjective visual performance benefits obtained through AO correction were confounded with neural limits. Artal
Aside from subjective tests, visual performance can be measured by objective tests, which only need subjects to keep fixated on the stimuli, using the electrophysiological techniques of visual evoked potential (VEP)[6], electroretinogram (ERG), and electrooculogram (EOG). Obviously, the objective tests have some advantages over subjective tests especially for infants, mental retardation, mental disorders, malinger, etc. Among these objective tests, VEP that represents the response of the visual vortex to visual stimuli has been shown to be valuable in assessing VA[7] and CS[8] objectively, especially in infants and individuals with short attention spans. In addition, the VEP is a useful tool to help with diagnosing visual pathway pathology objectively and is widely used in forensic practice.
But, as in the subjective tests, in order to measure retinal or visual pathway limits to vision by the objective VEP and other electrophysiological techniques, the optical limits should be first bypassed. As far as we know, using AO to bypass the optical limits in VEP measurement is a new technique. It has been found that using the VEP measurements in visual performance tests appears to improve diagnostic power[9,10]. It prompts us to consider the new technique of integrating VEP measurements into an AO system.
In this Letter, we report a setup of an objective visual performance evaluation system with VEP based on the AO system. The system will provide a platform for accurate assessment of visual performance with VEP measurements. Obviously, the new technique adds a new and reliable dimension to study the development of vision.
The preliminary research on VEP measurements without and with AO correction has been carried out on this system. Figure
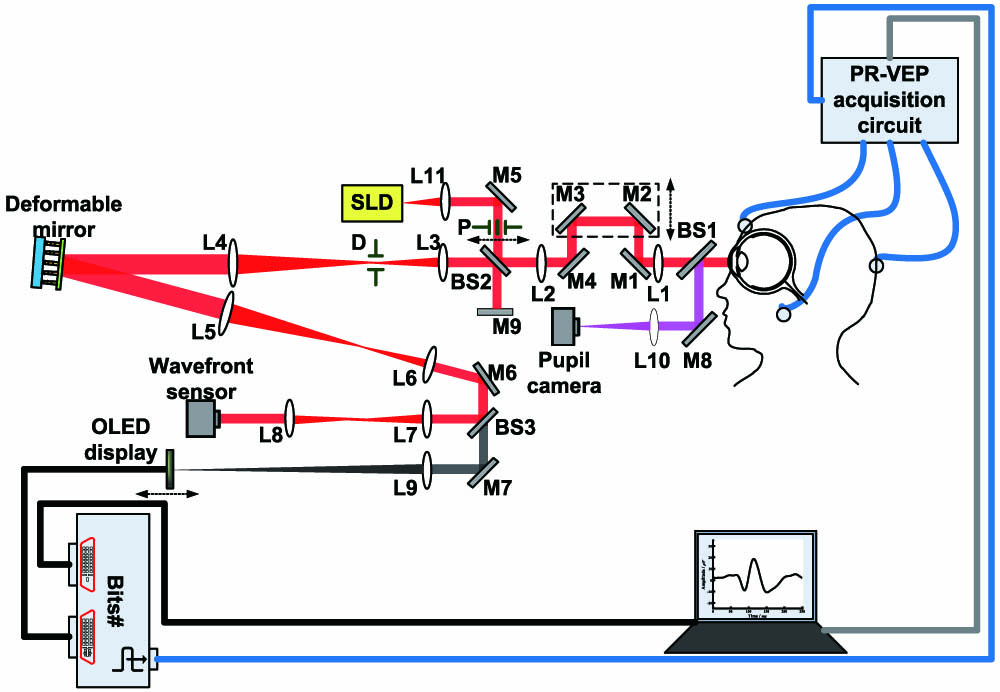
Fig. 1. AO system integrated with pattern reversal VEP (PR-VEP) measurements. SLD, superluminescent diode; BS, beam splitter; M, mirror; L, lens; P, artificial entrance pupil; D, diaphragm.
The AO aberration manipulation subsystem is mainly comprised of a wavefront sensor, a deformable mirror, and a closed-loop control system. The AO system design and layout analysis can be found elsewhere[11,12]. We only introduce the part of optical path. A beacon superluminescent diode (SLD) (shown in Fig.
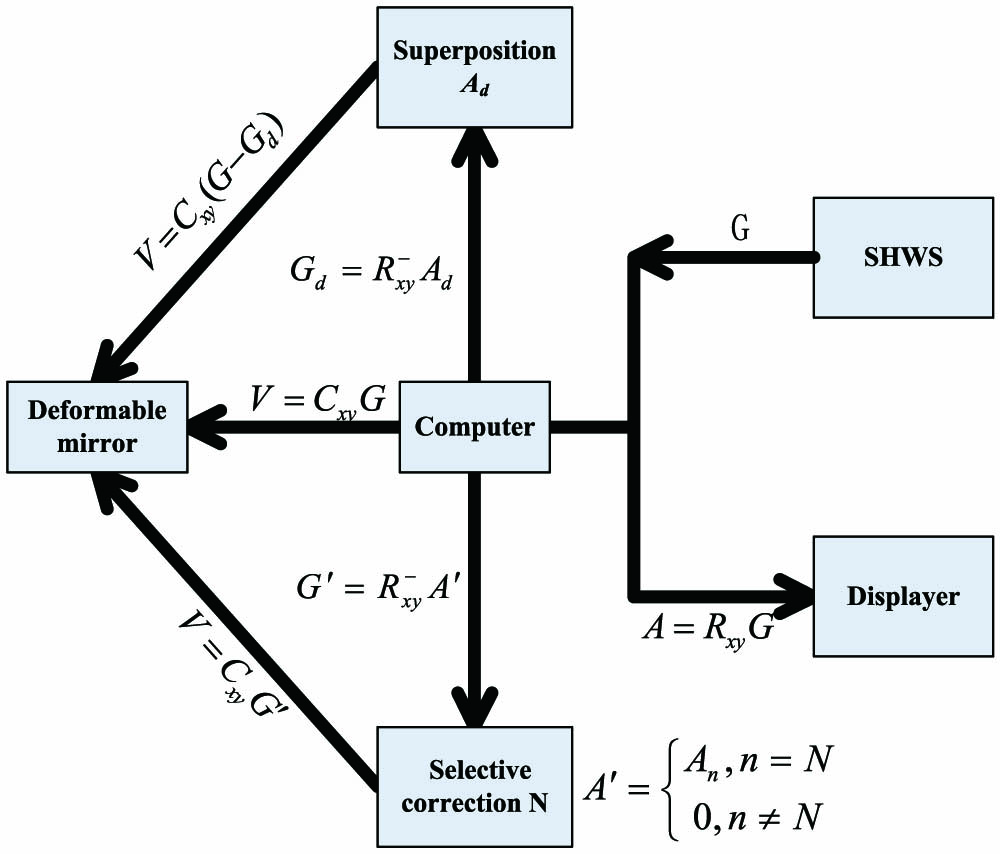
Fig. 2. Wavefront aberrations sensing and aberrations manipulation. , the pseudo inverse version of the reconstruction matrix; , selectively correcting the N th orders of Zernike aberrations; , the Zernike coefficient values of superposition aberrations; , the wavefront slope matrix corresponding to ; , the wavefront slope matrix corresponding to .
In the objective VEP measurements, the pattern stimuli can be presented in a pattern reversal way[14]. After pattern reversal stimulation, the electrophysiological activities can be recorded from electrodes attached to the scale in the visual cortex. The activities were amplified by the VEP acquisition circuit[15] (shown in Fig.
The stimuli were displayed by an organic light-emitting diode (OLED, EMA-100100, eMagin Corporation) monochrome black-and-white microdisplay with an effective size of
PR-VEP measurements were made in a dark room. The effective distance from the OLED’s screen to the eye was 200 mm. The filled size on the screen was 2°. For the VA or CS tests, the stimulus was a vertical sinusoidal grating with different frequencies. The subjects were instructed to look at a cross in the center of the screen.
To monitor the fixation status of a subject in real time, the system was miniaturized and mounted in a three-axis motorized translation platform, as shown in Fig.
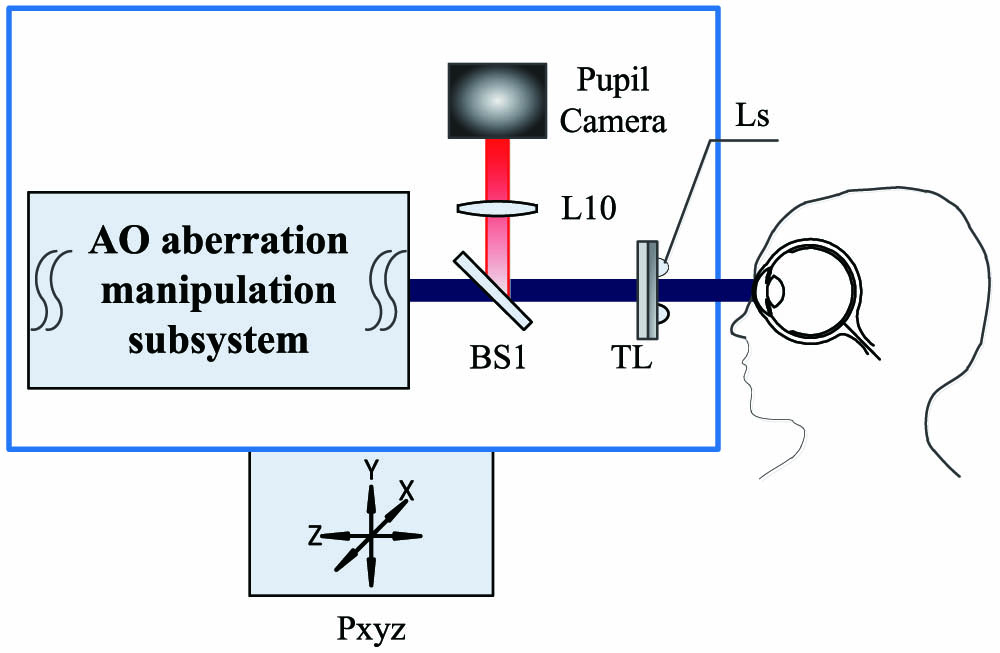
Fig. 3. Schematic of the miniaturized AO system. L, lens; BS, beam splitter; TL, trial lens holder; Ls, LED source; Pxyz, three-dimensional traveling platform.
Based on the developed AO system integrated with PR-VEP measurements, three volunteers (DP, ZZ, and CKY) were used to PR-VEP measurements without and with AO correction. The detailed information for all subjects is shown in Table
Table 1. Detailed Information for All Subjects
|
During the PR-VEP measurements, a vertical sinusoidal grating with a contrast of 0.9 and different spatial frequencies of 4, 8, and 16 cycles per degree (cpd) were used as the pattern stimulus. The reversal stimulation time frequency was 2 Hz or 4 reversals per second (rps). The PR-VEP waveforms at each spatial frequency were recorded without and with AO correction.
Figure

Fig. 4. (a), (b) Zernike coefficient values , and (c), (d) PSF of subject CKY without and with AO correction. (a), (c) without AO correction. (b), (d) with AO correction.
Figure
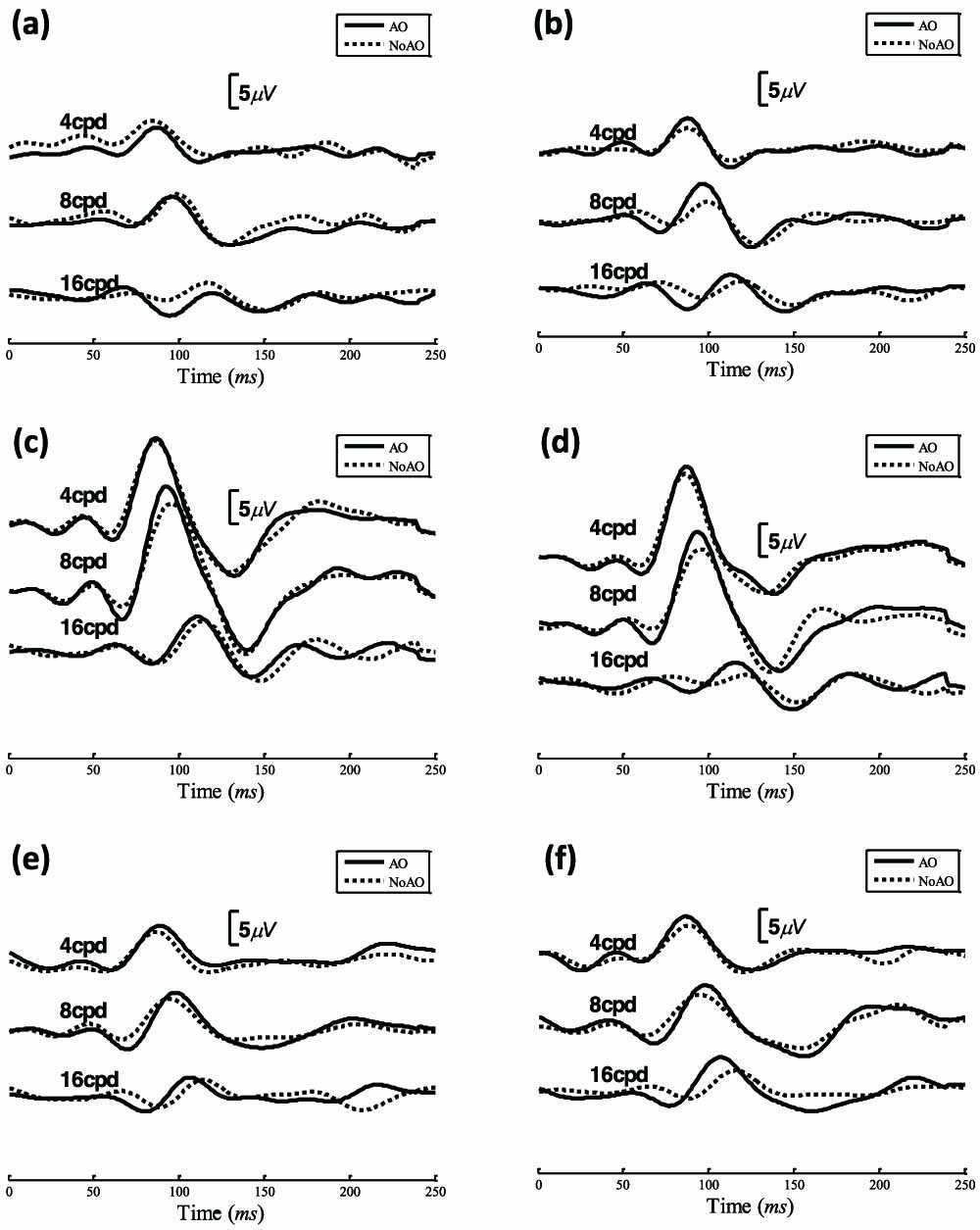
Fig. 5. PR-VEP waveform comparison of three subjects without and with AO correction. (a) and (b) are the right and left eye of DP, respectively. (c), (d) and (e), (f) correspond to ZZ and CKY, respectively.
For further analysis, the eigenvalues of NPN waveforms in the PR-VEP were extracted; that is, the first negative wave N1, the first positive wave P1, and the second negative wave N2, including amplitudes and latencies. The amplitude of N1 is absolute, the amplitudes of P1 and N2 are P1-N1 and P1-N2, respectively; the latency of N1, P1, and N2 is the time from the stimuli presentation to the appearance of positive or negative waves.
Figure
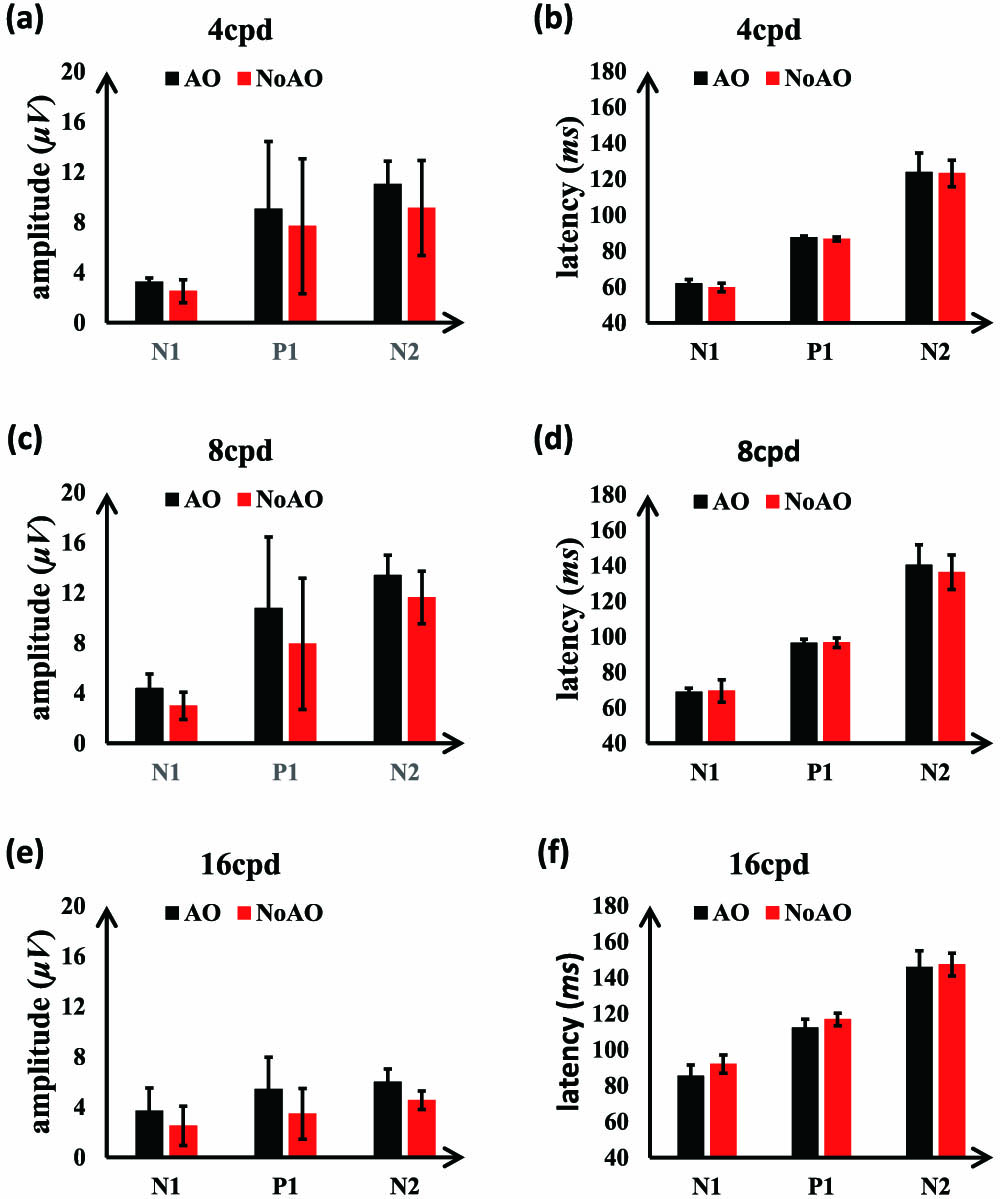
Fig. 6. Average NPN magnitude and latency of all eyes from three subjects. The first column (a), (c), (e) are the amplitudes at 4, 8, 16 cpd, respectively. The second column (b), (d), (f) are the latencies at 4, 8, 16 cpd, respectively.
These differences of NPN amplitudes and latencies after AO corrections could be interpreted that it improved retinal image quality and not changed the spatial frequencies and field size. While NPN amplitude reflected the number of excited nerve fibers that reached the visual cortex, NPN latency reflected the different transmission channel. As a result, more nerve cells were excited with AO corrections, and then the NPN amplitude was increased.
In conclusion, we integrated an objective visual performance evaluation with PR-VEP measurements into the AO system. It first used AO aberration manipulation technology in the PR-VEP measurements. The performance of the system was checked with objective PR-VEP measurements experiments without and with AO corrections. The results showed that the PR-VEP measurement was an objective and quantitative tool for studying the relationship between the ocular aberrations and visual performance that reflected the retinal and visual pathway limits to vision. Bypassing the optical and neural limits to vision, the PR-VEP measurements based on AO would be an accurate visual performance evaluation.
In addition, the subjective visual performance (VA and CS) tests and objective PR-VEP measurements can be taken under the same system. It was convenient and possible to compare between the two metrical methods. Also, the two metrics can be used to confirm each other. In these respects, the system provided the necessary technical and equipment support for future research on visual function.
The future work will be focused on using this system to study whether the subjective or objective evaluating method has the same sensitivity for visual performance changes and whether they reflect the same aspects of visual performance. Then, it is valuable to find subclinical markers of visual performance decline. Besides, this technique can be transited to binocular vision to assess binocular visual function with both subjective and objective metrical methods and in depth to understand the mechanism of binocular vision formation and binocular visual function maintenance.
[1]
[2]
[3]
[4]
[5]
[6]
[7]
[8]
[9]
[10]
[11]
[12]
[13]
[14]
[15]
Yanrong Yang, Junlei Zhao, Haoxin Zhao, Fei Xiao, Jiaxin Xie, Tiejun Liu, Yun Dai. Objective visual performance evaluation with visual evoked potential measurements based on an adaptive optics system[J]. Chinese Optics Letters, 2018, 16(5): 053301.