自然骨曲面对激光诱导空泡演化行为规律的影响
The liquid-assisted infrared laser ablation of hard biological tissue in clinical surgery is superior to the use of mechanical tools in terms of accuracy and compatibility. Owing to the pressure gradient inside and outside the bubble, the laser-induced bubbles expand, contract, and eventually collapse in the liquid environment. As the bubble collapses, the released energy causes the surface of the biological hard tissue to ablate. Moreover, the dynamic behavior of laser-induced bubbles is affected by boundary constraints. During pulsation, the nearby boundary can significantly affect the shape of the bubble and make it deviate from the original spherical shape, which results in the generation of a high-speed microjet when the bubble collapses. Therefore, the existence and shape of the boundary directly affect the ablation of the bubble microjet on the material. Bone tissue has a natural curvature and often imposes requirements associated with the drilling and cutting of curved surfaces during orthopedic surgery. Moreover, studying effects of curved surfaces of natural bones on the evolution behavior of nearby bubbles and generated microjets can improve the accuracy and rate of bone ablation and contributes to the practical application of laser ablation in orthopedic surgery. Therefore, this paper presents research on the effect of the curved boundary of natural bones on the bubble evolution behavior and generation of microjets and relative mechanisms, which is significant for guiding the improvement of laser water-assisted bone ablation.
The experimental system used in this study is shown in Fig. 1. Laser pulses with a wavelength of approximately 2 μm are transmitted from a fiber to a bone target immersed in distilled water to induce a bubble, and a high-speed camera is used to capture the transient features of the bubble. Fresh pork bones are used as the target material; the curved boundary of the joint (curvature radius r≈10 mm) is used as the target position, and distilled water is used as the liquid environment. Moreover, the bone target is fixed on the jig to ensure the target position is immersed in distilled water at a depth of 10 mm, and the distance between the end of the fiber and target surface is adjusted to obtain bubbles at different distances from the boundary. To study and compare the evolution characteristics of bubbles with different Rmax, the dimensionless distance
The shape of the bubble is asymmetric during stage Ⅰ owing to the extrusion of the bubble by the boundary of the bone surface ( column 2 in Fig. 2). During stage II, the bubble deviates from nearly spherical ( column 5 in Fig. 2) and becomes attached to the curved surface of the bone with a disc shape ( column 6 in Fig. 2) until its disappearance at the end of stage IV. During stage II, the bubble appears to shift to one side. As shown in Table 1, the time period of stages I and II of the bubble decreases with a decrease in the value of γ, and the time period of stage II is shorter than that of stage I. The time period of stages III and IV first decreases and then increases with the value of γ, reaching a minimum when γ=0.4. During stage II, the centroid of the bubble significantly shifts toward the higher side of the natural bone curved boundary. By analyzing the change in the position of the bubble centroid, the fiber preset offset for the positioning error compensation of the microjet effect is obtained. The numerical simulation results indicate that the pressure gradient at the bubble wall near the higher side of the surface boundary is smaller owing to the asymmetry of the natural bone curved boundary, and it is larger on the lower side of the surface boundary. This results in different moving speeds on the different sides of the bubble wall, causing the centroid of the bubble to shift to the higher side of the surface boundary. The direction of deflection of the micro-jet generated by the bubble is also similar to that of the displacement of the bubble centroid in late stage II of bubble formation. The micro-jet velocity generated by the bubble near the natural bone surface with 0.2≤γ≤0.6 is obtained, and the velocity of the micro-jet generated by the bubble increases as γ decreases.
In this study, the evolutionary behavior and mechanism of a 2 μm wavelength laser-induced bubble with 0.2≤γ≤0.6 near the boundary of a natural bone curved surface are investigated. Based on the results, the time period of stages I and II of bubble pulsation near the boundary of the curved surface of the natural bone decreases with a decrease in γ, the time period of stages III and IV first decreases and then increases with γ, and the periods of the bubble expansion and contraction phases are different. Owing to the irregular shape of the natural bone curved boundary, the shape and evolutionary behavior of the bubble may appear asymmetrical. When the bubble contracts, the centroid of the bubble is shifted to the higher side of the natural bone curved boundary, and the microjet generated by the bubble deflects in the same direction. The numerical simulation results show that the asymmetry of the natural bone curved boundary causes a pressure gradient and different moving speeds near the two sides of the bubble wall when the bubble shrinks, causing the centroid of the bubble and bubble wall to shift to the higher side of the natural bone curved boundary. Owing to the deviation in the microjet direction, the ablation accuracy in certain applications such as laser water-assisted bone ablation is affected. Moreover, the fine error compensation is performed in this study for related operations by presetting the offsets of the fiber at a natural bone surface position and using different γ values. Laser water-assisted bone ablation can actually be applied in surgery when the micro-jet velocity increases with a decrease in γ, and the ablation rate of bone tissue can be improved by appropriately reducing γ.
1 引言
液体辅助红外激光消融硬生物组织已成为提高手术精确度和增强兼容性的重要技术[1-2],越来越受到研究人员的关注。激光在液体环境中诱导产生空泡,空泡会在泡内外压力梯度的作用下膨胀和收缩,直至溃灭,空泡溃灭过程中释放出的能量导致材料表面发生消融[3-4]。激光诱导空泡的动力学行为受到液体黏度[5]、表面张力[6]、可压缩性[7]和边界约束[8]等的影响,在其脉动过程中,附近的边界会显著影响空泡的形状[9-10]。骨组织具有自然的曲率和形状,研究其对空泡运动特性的影响以及由此产生的微射流,可以提高消融的准确性和速率,有助于推动激光消融在骨科手术中的实际应用。
刚性边界附近空泡的动力学已有一些相关研究。Zhang等[11]给出了空泡脉动周期的无量纲时间长度与无量纲距离(γ)的关系,发现边界约束会延长空泡的脉动过程。有研究认为,当空泡接近刚性直角边界时,由于边界阻碍了附近水层的运动,边界附近空泡的溃灭时间比理想瑞利空泡溃灭时间长22%[12]。Požar等[13]研究了凹曲面边界附近的空泡动力学,发现在相同γ值下,相较于平面边界,凹边界更能阻碍空泡周围的液体流动。Tomita等[14]发现,当曲面边界凸出时,空泡脉动周期变短,而在凹曲面边界处空泡脉动周期变长。Aziz等[15]研究了偏离曲面对称轴的弯曲边界附近的空泡演化过程。本课题组前期以平面骨为对象,分析了平面骨附近的空泡微射流对骨组织的消融效果及其影响因素,给出了微射流消融骨组织的机理[16],并提出了一种快速、安全的激光骨科手术方案[17]。Andrews等[18]发现,壁面形貌会对边缘附近的空泡演化行为及其破裂微射流行为产生直接影响,从而影响非平面壁材料的消融效果。现有关于边界约束激光空泡的研究大都选择理想的规则几何形状边界,而骨组织具有非规则曲面形状,曲面边界形状会对激光水辅助消融骨组织过程中的空泡演化行为产生影响,进而影响消融效果。
本文针对骨的自然曲面,探究了激光水辅助消融骨组织过程中自然曲面边界附近的空泡演化过程,系统捕捉和分析了水环境下激光诱导骨组织曲面边界附近空泡的形状、大小和演化过程及微射流形成等,研究了自然骨曲面边界对空泡运动特性和微射流的影响及其机制,为激光水辅助消融骨手术提供了指导。
2 实验材料与方法
采用新鲜的离体猪胫骨用于激光消融实验,选取了曲率半径与人掌骨头和尺骨头相似的自然曲面骨作为消融靶材(曲率半径r≈10 mm)[19],离体骨靶材按照标准的生物骨样品处理方法获得[16]。实验中,将离体骨靶材固定在调节精度为0.01 mm的夹具上,以调节光纤端部到离体骨靶材的垂直距离。离体骨靶材浸没在无杂质的蒸馏水中,骨靶材顶部到蒸馏水液面的距离为10 mm。每组实验后更换靶材。为了便于研究和对比具有不同最大半径(Rmax)的空泡的演化特性,定义无量纲距离
采用基于液体体积(VOF)模型的两相流求解器对非对称曲面附近空泡收缩阶段的演化过程进行仿真,以空泡演化过程中偏移现象较为显著的γ=0.5的情况为例进行描述。该求解器的控制方程包括连续性方程、动量方程、能量方程、状态方程。基于流体不可压缩假设,空泡中只包含可视为理想气体的水蒸气,忽略空泡与液体环境间的传热传质以及温度对空泡体积的影响[20]。由于空泡初始半径达到了0.3 mm,因此水的黏度和表面张力的影响可以忽略[14,21]。
3 实验结果与分析
由于自然骨曲面并非规则几何形状,因此其表面轮廓不对称。如
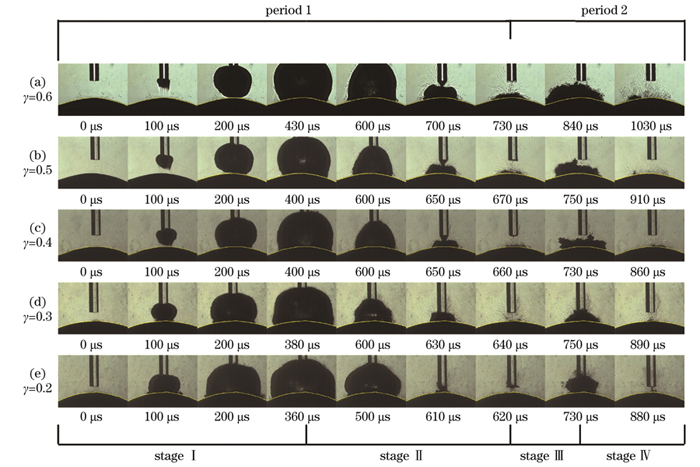
图 2. 自然骨曲面边界附近空泡演变的时间序列图
Fig. 2. Time series diagrams of evolution of cavitation bubble near boundary of natural curved bone surface
当无量纲距离较大(γ=0.6,0.5)时,如
表 1. 不同γ值下空泡各演化阶段的时间长度
Table 1. Duration of each evolution stage of cavitation bubbles with different γ values
|
表 2. 不同γ值下空泡溃灭微射流作用位置的偏移
Table 2. Deviation of impact position of cavitation bubble collapse micro-jet with different γ values
|
表 3. 不同γ值空泡形成的微射流的速度
Table 3. Velocity of micro-jet formed by cavitation bubble with different γ values
|
空泡膨胀至最大时,由于惯性,空泡内部压强远小于外部液体环境压强,在压力梯度的作用下,空泡随即进入第一周期的收缩阶段。骨边界表面的摩擦力导致空泡与骨边界接触的部分收缩较慢,空泡上部收缩更快,因此收缩初期的空泡呈钟形,如
空泡在第一周期脱离光纤端面时(
使用Image-Pro Plus软件逐帧测量高速摄影图像中空泡的质心坐标位置,以骨曲面顶点为原点,图像中从左至右为X轴正方向,从下至上为Z轴正方向,获得的质心位置随时间的变化规律如

图 3. 不同γ值下空泡质心位置随时间的变化。(a)γ=0.6;(b)γ=0.5;(c)γ=0.4;(d)γ=0.3;(e)γ=0.2
Fig. 3. Position of cavitation bubble centroid versus time under different γ. (a) γ=0.6; (b) γ=0.5; (c) γ=0.4; (d) γ=0.3; (e) γ=0.2
在空泡的整个脉动过程中,γ=0.6和γ=0.5的空泡质心明显向左偏移,如
由于运动特性的不对称,无法利用Rayleigh-Plesset方程对自然骨曲面附近空泡的形状尺寸与泡壁速度进行预测。选取VOF模型对非对称曲面附近空泡的收缩过程进行数值模拟,探究自然骨曲面附近空泡质心偏移的原理,数值模拟结果如

图 4. 非对称曲面边界附近γ=0.5的空泡非对称收缩时周边压力与速度的数值模拟图及对应的高速摄影图。(a)当空泡演化至650 μs时空泡附近的压力分布;(b)当空泡演化至650 μs时空泡附近的流体速度分布;(c)当空泡演化至650 μs时的高速摄影图;(d)当空泡演化至660 μs时空泡附近的压力分布;(e)当空泡演化至660 μs时空泡附近的流体速度分布;(f)当空泡演化至660 μs时的高速摄影图
Fig. 4. Numerical simulation of pressure and velocity when cavitation bubble shrinks asymmetrically near asymmetric boundary with γ=0.5 and corresponding high speed photographies. (a) Pressure distribution near cavitation bubble as bubble evolves to 650 μs; (b) fluid velocity distribution near cavitation bubble as bubble evolves to 650 μs; (c) high speed photography as bubble evolves to 650 μs ; (d) pressure distribution near cavitation bubble as bubble evolves to 660 μs; (e) fluid velocity distribution near cavitation bubble as bubble evolves to 660 μs ; (f) high speed photography as bubble evolves to 660 μs
通过前述分析可知,当空泡在曲面骨靶材壁面附近溃灭时,空泡在收缩与溃灭过程中的演化是不对称的。在溃灭初期,空泡收缩为扁球状,然后远离骨靶材一侧的空泡泡壁向内凹陷,凹陷持续发展,形成指向骨靶材壁面的溃灭微射流。最后,微射流穿透空泡并冲击骨靶材表面。这是激光诱导空泡消融骨组织的主要原因,微射流的速度与方向会直接决定骨组织的消融效果。从微射流形成的那一刻起,微射流的速度几乎保持不变[16]。因此定义微射流的速度(vj)为微射流从形成时到作用于骨靶材表面时的平均速度:
式中:t0和t1分别为微射流形成和微射流冲击空泡底部接触到骨靶材的时刻;ΔH为微射流尖端在t0和t1时刻的距离。通过在高速摄影照片中测量ΔH以及对应的帧间隔,可得到不同γ值时的微射流速度vj,如
4 结论
研究了激光(波长~2 μm)诱导空泡在自然骨曲面边界附近的演化规律及其机制。自然骨曲面边界附近空泡脉动的第Ⅰ、Ⅱ阶段的时间长度随γ值的降低而减小,第Ⅲ、Ⅳ阶段的时间长度随γ值的降低先减小后增加,且空泡的膨胀阶段与收缩阶段的时间长度不同;由于自然骨曲面边界的形状不规则,自然骨曲面边界上的空泡形状和演化行为也出现了明显的不对称,空泡收缩时质心向自然骨曲面边界较高一侧发生偏移,空泡产生的微射流方向也向曲面边界较高一侧偏移。同时,得到了自然骨曲面附近0.2≤γ≤0.6的空泡产生的微射流速度。空泡收缩过程的数值模拟分析结果表明,自然骨曲面边界两侧高度不同使得附近空泡收缩过程中左右两侧泡壁处的压力梯度不同,泡壁移动速度不同,导致空泡质心和两侧泡壁向曲面边界较高的一侧偏移。由于微射流方向发生偏移,在激光水辅助骨消融手术等应用中,这一现象会影响消融精度,通过给出不同γ下光纤向自然骨曲面较低位置的预置偏移量,为相关术式提供精细的误差补偿。由于微射流速度随着γ的减小而提高,当激光水辅助骨消融技术应用于手术时,可以通过适当减小γ来提高骨组织消融的速率。
[1] 吕茗萱, 石晓卫, 薛建伟, 等. 激光诱导液体微射流效应及其在医学领域的应用[J]. 激光与光电子学进展, 2021, 58(11): 1100004.
Lü M X, Shi X W, Xue J W, et al. Laser-induced liquid micro-jet effect and its application in medical field[J]. Laser&Optoelectronics Progress, 2021, 58(11): 1100004.
[2] 薛建伟, 吴灵锦, 石晓卫, 等. 9.3 μm脉冲CO2激光辐照牙硬组织的消融特性研究[J]. 中国激光, 2022, 49(15): 1507105.
[3] Gu J Y, Luo C H, Lu Z B, et al. Bubble dynamic evolution, material strengthening and chemical effect induced by laser cavitation peening[J]. Ultrasonics Sonochemistry, 2021, 72: 105441.
[4] Giglio N C, Hutchens T C, South A A, et al. Dynamic properties of surfactant-enhanced laser-induced vapor bubbles for lithotripsy applications[J]. Journal of Biomedical Optics, 2021, 26(1): 018001.
[5] Long J Y, Eliceiri M H, Ouyang Y X, et al. Effects of immersion depth on the dynamics of cavitation bubbles generated during ns laser ablation of submerged targets[J]. Optics and Lasers in Engineering, 2021, 137: 106334.
[6] Senegačnik M, Kunimoto K, Yamaguchi S, et al. Dynamics of laser-induced cavitation bubble during expansion over sharp-edge geometry submerged in liquid-an inside view by diffuse illumination[J]. Ultrasonics Sonochemistry, 2021, 73: 105460.
[7] Zhong X X, Eshraghi J, Vlachos P, et al. A model for a laser-induced cavitation bubble[J]. International Journal of Multiphase Flow, 2020, 132: 103433.
[8] Han B, Chen J, Shen Z H, et al. Investigation of the wedge-shaped propelled surface for laser propulsion in water environment[J]. Optics & Laser Technology, 2011, 43(3): 604-608.
[9] Zhao R, Xu R Q, Shen Z H, et al. Experimental investigation of the collapse of laser-generated cavitation bubbles near a solid boundary[J]. Optics & Laser Technology, 2007, 39(5): 968-972.
[10] Luo C H, Gu J Y, Tong Z P, et al. Dynamics of laser-induced cavitation bubbles near a short hole and laser cavitation processing with particles[J]. Optics & Laser Technology, 2021, 135: 106680.
[11] Zhang S, Zhang A M, Wang S P, et al. Dynamic characteristics of large scale spark bubbles close to different boundaries[J]. Physics of Fluids, 2017, 29(9): 092107.
[12] Zhang Y N, Qiu X, Zhang X Q, et al. Collapsing dynamics of a laser-induced cavitation bubble near the edge of a rigid wall[J]. Ultrasonics Sonochemistry, 2020, 67: 105157.
[13] Požar T, Agrež V, Petkovšek R. Laser-induced cavitation bubbles and shock waves in water near a concave surface[J]. Ultrasonics Sonochemistry, 2021, 73: 105456.
[14] Tomita Y, Robinson P B, Tong R P, et al. Growth and collapse of cavitation bubbles near a curved rigid boundary[J]. Journal of Fluid Mechanics, 2002, 466: 259-283.
[15] Aziz I A, Manmi K M A, Saeed R K, et al. Modeling three dimensional gas bubble dynamics between two curved rigid plates using boundary integral method[J]. Engineering Analysis With Boundary Elements, 2019, 109: 19-31.
[16] Zhang L T, Ji L F, Zhang H L, et al. Dynamics characteristics of a laser-induced non-spherical bubble collapsing micro-jet and its enhancement on hard tissue ablation[J]. Optics and Lasers in Engineering, 2022, 151: 106893.
[17] Ji L F, Zhang L T, Cao L J, et al. Laser rapid drilling of bone tissue in minimizing thermal injury and debris towards orthopedic surgery[J]. Materials & Design, 2022, 220: 110895.
[18] Andrews E D, Rivas D F, Peters I R. Cavity collapse near slot geometries[J]. Journal of Fluid Mechanics, 2020, 901: A29.
[19] Dumont C, Ziehn C, Kubein-Meesenburg D, et al. Quantified contours of curvature in female index, middle, ring, and small metacarpophalangeal joints[J]. The Journal of Hand Surgery, 2009, 34(2): 317-325.
[20] Mishra A, Mondal J, Roy A, et al. Effect of curved rigid surface on the collapsing cavitating bubble in cryogenic environment[J]. IOP Conference Series: Materials Science and Engineering, 2020, 755: 012067.
[21] Li S, Han R, Zhang A M. Nonlinear interaction between a gas bubble and a suspended sphere[J]. Journal of Fluids and Structures, 2016, 65: 333-354.
[22] Gonzalez-Avila S R, Denner F, Ohl C D. The acoustic pressure generated by the cavitation bubble expansion and collapse near a rigid wall[J]. Physics of Fluids, 2021, 33(3): 032118.
[23] 付磊, 王萍, 王斯佳, 等. 纳秒脉冲激光诱导的水中双空泡振荡研究[J]. 中国激光, 2022, 49(4): 0407001.
[24] Wang Q X. The evolution of a gas bubble near an inclined wall[J]. Theoretical and Computational Fluid Dynamics, 1998, 12(1): 29-51.
李学坤, 季凌飞, 张犁天, 张洪龙. 自然骨曲面对激光诱导空泡演化行为规律的影响[J]. 中国激光, 2023, 50(9): 0907203. Xuekun Li, Lingfei Ji, Litian Zhang, Honglong Zhang. Influence of Natural Curved Bone Surface on Evolution Behavior of Laser‑Induced Cavitation Bubble[J]. Chinese Journal of Lasers, 2023, 50(9): 0907203.