双金属锥形光纤表面增强拉曼散射探针拉曼增强特性
Surface-enhanced Raman scattering (SERS) is a powerful analytical tool that can provide molecular vibrational fingerprint information. Due to its unique analytical advantages, SERS has been widely applied in various fields such as biomedical research, environmental monitoring, and food analysis. During the development of SERS technology, silver nanoparticles have often been used as SERS substrates due to their excellent localized surface plasmon resonance properties. However, their susceptibility to oxidation poses a significant limitation in the construction of nano-probes for practical applications. In contrast, gold nanoparticles have better chemical stability and lower biotoxicity but relatively weaker Raman signal enhancement capability. Therefore, bimetallic SERS substrates combining gold and silver are characterized by high sensitivity and stability. Compared with traditional substrates, tapered optical fibers not only have the advantages of in-situ detection and remote signal transmission but also have great potential for enhancing laser reflection and improving light collection capability, which is beneficial for collecting weak Raman signals and achieving lower detection limits. Therefore, we proposed a simple and cost-effective bimetallic tapered optical fiber SERS probe. This probe utilized a light-induced method to deposit gold and silver nanoparticles on the surface of the tapered optical fiber, providing high sensitivity and good stability.
The bimetallic tapered optical fiber SERS probe was prepared by using a light-induced method in this study. First, the tapered fiber was prepared by using the fiber fusion tapering machine. After clamping the processed fiber onto the fiber holder, the fiber fusion tapering machine was started to initiate the tapering process. Once the machine stopped operating, two tapered optical fibers were obtained. Second, gold and silver nanoparticles with an approximate diameter of 50 nm were prepared by using a chemical reduction method. Finally, the bimetallic tapered optical fiber SERS probe was prepared through the light-induced method. The laser beam emitted by a helium-neon laser was coupled into the tapered optical fiber. Subsequently, the tapered end was immersed in a mixed solution of Ag sol and Au sol (volume ratio of 1∶1, both 0.3 mL) for 60 seconds. Then, the tapered end was moved from the solution to the air and kept in that position for 90 seconds while the laser was still operating. This process was repeated 15 times [Fig. 1(a)]. At the end of the entire procedure, the bimetallic tapered optical fiber SERS probe was obtained. The surface morphology of the tapered optical fiber was characterized by scanning electron microscopy (SEM), and the performance of the optical fiber probe was tested by a confocal Raman spectrometer.
In this study, SEM analysis [Fig. 2(a1) and (a2)] of the prepared samples revealed a uniform distribution of metal nanoparticles on the fiber surface, exhibiting a mostly monolayer arrangement. The relative mass percentages of Ag and Au elements were found to be 2.36% and 9.21%, respectively [Fig. 2(b) and (c)]. The average particle size of the metal particles on the sample was 49 nm, with an average gap of 6.8 nm [Fig. 2(e1) and (e2)]. In the paper, Rhodamine 6G (R6G) was selected as the analyte molecule to evaluate the SERS performance of the prepared samples. The bimetallic tapered optical fiber SERS probe was immersed in R6G solutions with concentrations ranging from 10-8 to 10-10 mol/L for three minutes respectively. After removal from the solution and natural drying, Raman signal detection was performed, and the obtained enhancement factor (AEF) for the samples reached 2.07×108. To demonstrate the capability of detecting non-single molecule analytes, the samples were immersed in a mixed solution of 10-6 mol/L R6G and 10-4 mol/L crystal violet (CV) for three minutes. After removal from the solution and natural drying, the detection was conducted, and the results indicated that the unique Raman peaks of different probe molecules could be detected in the mixed solution [Fig. 6(b)]. To demonstrate the excellent stability of the bimetallic tapered optical fiber SERS probe, it was placed for different durations of 24 hours, 48 hours, 72 hours, and 96 hours. Afterward, a comparison was made by using Raman testing of 10-7 mol/L R6G. The results indicated that the sample exhibited good stability (Fig. 7).
In this study, to enhance the enriched density of metal nanoparticles on the surface of optical fibers and improve the stability of the Raman-enhancing properties of the optical fiber SERS composite structure, we proposed a bimetallic (gold and silver) tapered optical fiber SERS probe structure. First, gold and silver nanoparticles with uniform morphology were prepared using the chemical reduction method. Then, the bimetallic particles were enriched on the tapered optical fiber using light-induced methods. The prepared optical fiber SERS probe exhibited excellent experimental performance. In this study, the lowest detected concentration of R6G was as low as 10-10 mol/L, and the enhancement factor was 2.07×108; compared with single-metal silver optical fiber SERS probes, the stability of the bimetallic sample was improved by seven times (after 96 hours). The bimetallic tapered optical fiber SERS probe is expected to be applied in in-situ and remote detection in the future. The next research direction is to explore the key process of controllable double metal modified optical fiber, so as to further optimize the detection sensitivity and stability of the sample.
1 引 言
表面增强拉曼散射(SERS)是一种强大的分析工具,能够提供分子的振动“指纹”信息。由于其独特的分析优势,SERS在生物医学[1-2]、环境保护[3-4]、食品检测[5-6]等领域得到了广泛的应用。
1974年,Fleischmann等[7]发现当有机分子吡啶被吸附在粗糙的金属表面时,拉曼信号会被显著增强。此后,科学家们开始研究如何用各种贵金属纳米粒子基底放大拉曼信号。虽然银纳米粒子由于其出色的局域表面等离子体共振特性常被用作SERS基底,但其易被氧化的缺点使得在构建纳米探针的应用中受到很大限制。相比之下,金纳米粒子的化学稳定性更好、生物毒性更低,但拉曼信号增强能力相对较弱[8]。因此,很多科学家将研究重点放在了双金属基底方面。例如:Bian等[9]通过一步法制备了SiO2@Au/Ag合金核壳纳米粒子并实现了对结晶紫(CV)拉曼信号的有效放大;Shinki等[10]利用共聚焦磁控溅射系统共溅射Au和Ag,在锥体硅表面沉积Au和Ag比例为1∶1的合金薄膜,实现了对浓度为10-9 mol/L的罗丹明6G(R6G)的检测;Li等[11]通过化学合成法制备了Au-Ag核壳纳米金字塔,用于生物相关小分子的SERS鉴别。为了进一步将SERS技术应用于原位监测和远程检测方面[12],光纤是一种很好的选择。
光纤作为信号远程传输的良好载体,将其与SERS结合起来,可以在远程检测方面提供更便利的条件。此外,锥形光纤不仅可以将倏逝场传递到周围环境中,而且增加了倏逝场作用范围。因此,它具有增强激光反射和提高集光能力的巨大潜力,这对于收集弱拉曼信号、获得更低的检测极限非常有帮助[13]。基于这些优点,大量的锥形光纤SERS探针被制备出。例如:Yu等[14]在锥形光纤上富集了银纳米粒子,并结合腔增强技术成功地检测到浓度为10-11 mol/L的R6G;Liu等[15]制备了环状锥形光纤SERS探针,对CV的检测极限为2×10-10 mol/L;Cao等[16]利用硅烷化处理后的锥形光纤合成了光纤SERS探针,成功检测到4-氨基苯硫酚(4-ATP),检测极限为10-9 mol/L。尽管上述锥形光纤实现了低检测极限和信号远程传输,但均在光纤尖端上沉积单金属纳米粒子来增强拉曼信号,忽略了双金属的卓越光学性能。因此,有必要制备一种双金属锥形光纤SERS探针,以获得更低的检测极限和更高的稳定性。
针对上述问题,本文提出一种简单经济的双金属锥形光纤SERS探针,该探针利用自组装的方式在锥形光纤表面上沉积金银纳米粒子,具有高灵敏度和良好的稳定性。
2 实验部分
2.1 锥形光纤制备
利用拉锥机(FSCW-3000,中国山东福寿光电子通信有限公司生产)制备锥形光纤,如

图 1. 实验流程图。(a)光纤SERS探针制备流程示意图;(b)光纤SERS探针实物图及电镜图;(c)拉曼检测示意图
Fig. 1. Experimental flowchart. (a) Schematic diagram of the preparation process of the fiber SERS probe; (b) real image and SEM image of the fiber SERS probe; (c) schematic diagram of Raman detection
2.2 金属纳米粒子制备
采用一步还原法制备银纳米粒子[17],其中,氢氧化钠和氯化银均为2.5 mL,在黑暗条件下持续搅拌2 h,即可得到粒径约为50 nm的银纳米粒子。采用氯金酸还原法制备金纳米粒子[18],具体步骤如下:首先,配置质量分数为1%的氯金酸溶液;其次,配置质量分数为1%的柠檬酸钠溶液;然后,将1 mL质量分数1%的氯金酸溶液加入99 mL去离子水中,再向其中加入1 mL 1%的柠檬酸钠溶液;接着,将溶液加热至沸腾并保持15 min;最后,自然冷却即可得到平均粒径为50 nm的金纳米粒子。
2.3 锥形光纤SERS探针制备
采用光诱导的方法将金纳米粒子(AuNPs)和银纳米粒子(AgNPs)富集在锥形光纤尖端,具体的实验流程如
2.4 拉曼测试
选择R6G作为分析物分子,以检测制备样品的SERS性能。将锥形光纤SERS探针分别浸入浓度为10-8~10-10 mol/L R6G溶液中3 min,然后从溶液中取出,自然干燥后进行拉曼信号的检测。进行多分子检测时,将样品浸入浓度为10-6 mol/L R6G和10-4 mol/L CV的混合溶液中3 min,然后从溶液中取出,自然晾干后进行检测。检测原理如
2.5 材料与仪器
样品表面的形态用扫描电子显微镜(SEM,Quattro S,赛默飞世尔公司)表征,拉曼测试实验在共聚焦拉曼光谱仪(LabRAM HR Evolution,Horiba Jobin Yvon S.A.S)上进行,激发波长为532 nm,功率为2.5 mW,积分时间为10 s。使用LapSpec软件去除原始拉曼光谱的基线。
3 结果与讨论
3.1 SEM和EDS表征
对制备的样品进行SEM分析,结果如
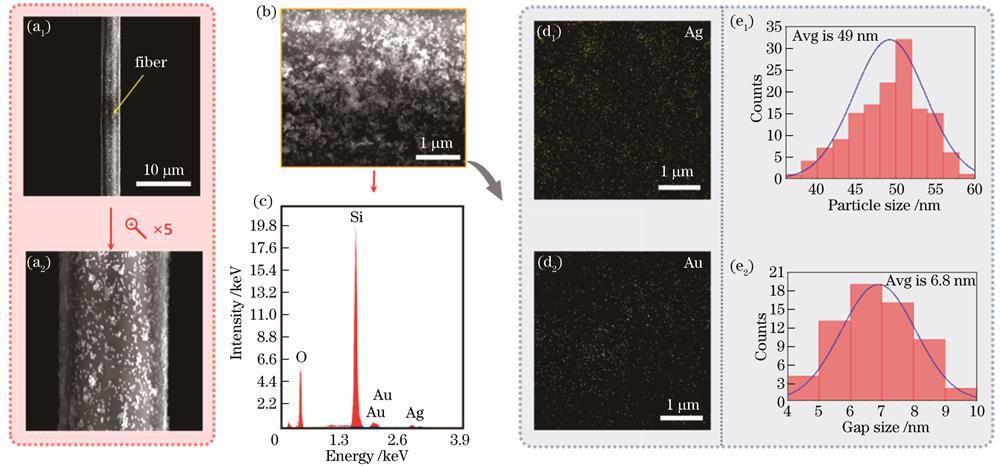
图 2. 锥形光纤SERS探针表征图。(a1)(a2)SEM图;(b)进行EDS分析的SEM图;(c)对锥形光纤SERS探针表面的元素类型进行EDS分析;(d1)(d2)元素分布;(e1)粒径统计;(e2)间隙统计
Fig. 2. Characterization of the tapered optical fiber SERS probe. (a1)(a2) SEM images; (b) SEM image for EDS analysis; (c) EDS analysis of the element types on the surface of the tapered optical fiber SERS probe; (d1)(d2) element distribution; (e1) statistical results of particle size; (e2) statistical results of gap size
为了更好地观察基底上AgNPs和AuNPs的富集情况,使用energy dispersive spectrometer(EDS)对如
3.2 双金属富集机理
当锥形光纤尖端浸没在双金属纳米粒子混合溶液中时,激光通过光纤芯传播到锥形端,大部分功率从光纤尖端锥形区逃逸形成倏逝场,倏逝场与周围介质中的纳米粒子相互作用,产生散射力和梯度力。当梯度力远远大于散射力时,形成的合力便会将金属纳米粒子拉向锥形光纤表面[19-21],如
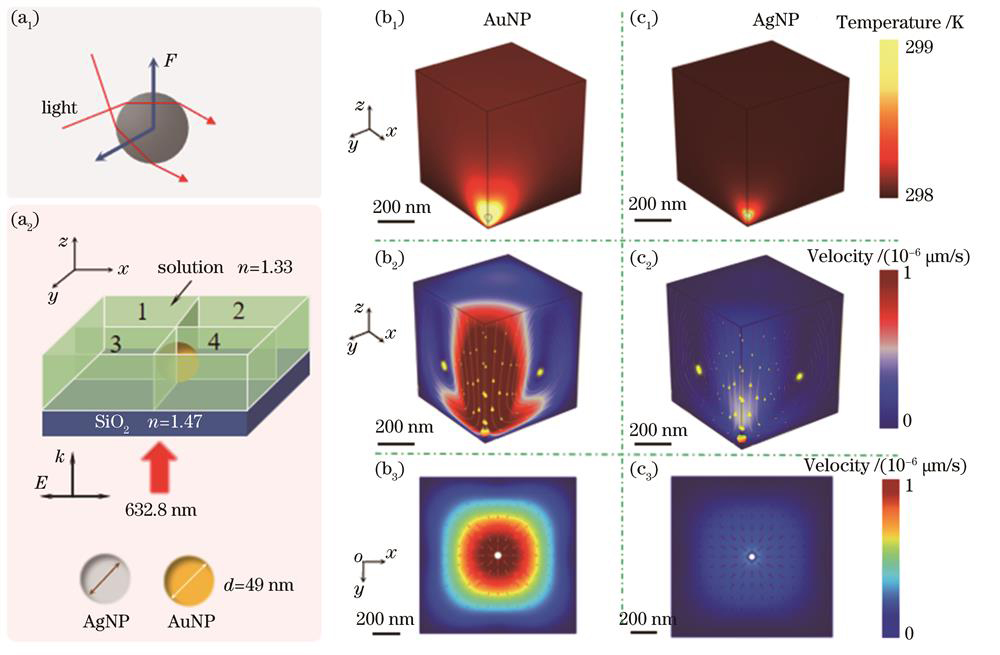
图 3. 纳米粒子富集原理图。(a1)粒子受力示意图;(a2)模型示意图;(b1)AuNP温度场;(b2)AuNP三维流速场;(b3)AuNP二维流速场;(c1)AgNP温度场;(c2)AgNP三维流速场;(c3)AgNP二维流速场
Fig. 3. Schematic of silver nanoparticle enrichment. (a1) Stress of nanoparticle; (a2) schematic diagram of the simulation model; (b1) temperature field of AuNP; (b2) 3D velocity field of AuNP; (b3) 2D velocity field of AuNP; (c1) temperature field of AgNP; (c2) 3D velocity field of AgNP; (c3) 2D velocity field of AgNP
当锥形光纤从溶液拉至空气中后,由于上述力及表面张力的作用,锥形光纤表面存在携带大量金属纳米粒子的溶胶[15]。为了深入探究锥形光纤表面金属纳米粒子的自组装机理,利用Comsol Multiphysics多物理软件进行模拟分析。在波长为632.8 nm、x方向偏振的激光照射下,将直径为49 nm的金属纳米球置于水环境中的二氧化硅表面上,并选择第1部分进行分析,如
3.3 电磁场数值计算
为了探究双金属锥形光纤SERS的理论机理,使用Comsol Multiphysics计算二维光纤上的电磁场分布。根据之前金属纳米粒子尺寸和间隙的统计数据,设置金属粒子的直径为49 nm,粒子间隙为6.8 nm。根据EDS分析结果以及单个金、银纳米粒子的密度(金为19.3 g/cm3,银为10.53 g/cm3),将光纤表面的金和银排列为规则的Au-Au-Ag结构(金和银粒子数比为2∶1)。此外,设置入射激光波长为532 nm,沿x轴偏振,并沿z轴传播,将周围介质设置为空气,并使用完美匹配层(PML)作为边界条件,如
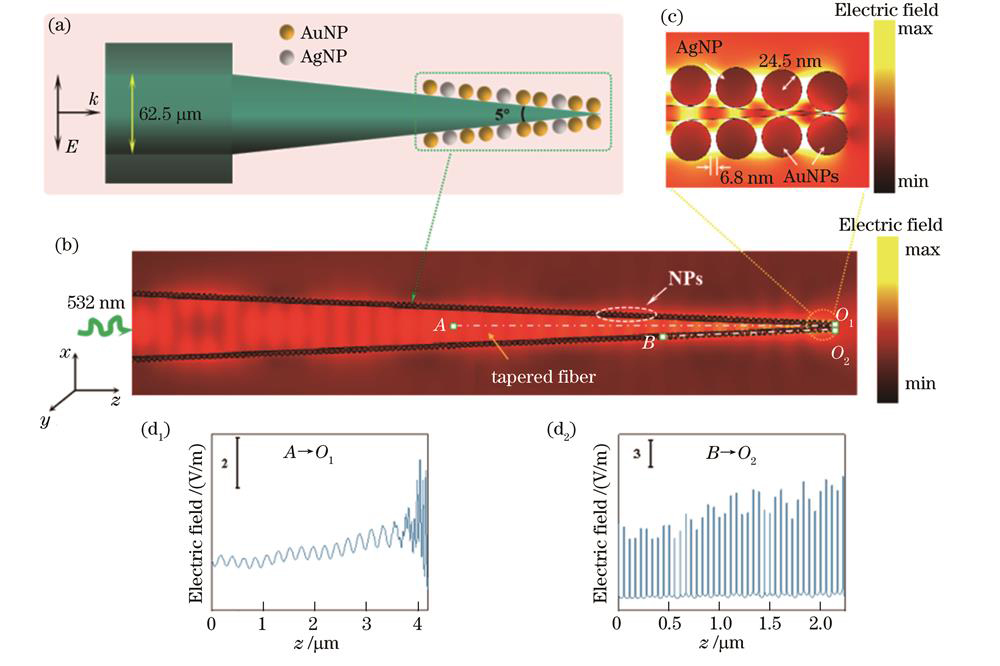
图 4. 电磁场仿真图。(a)锥形光纤SERS探针示意图;(b)光纤前端电场强度仿真图;(c)尖端部分放大的电场图;(d1)(d2)A-O1和B-O2的电场强度
Fig. 4. Simulation of electromagnetic field. (a) Schematic diagram of the tapered optical fiber SERS probe; (b) simulation of electric field intensity at the front end of the fiber; (c) enlarged electric field map at the tip; (d1) (d2) electric field intensity from point A to O1 and B to O2
为了表征拉曼增强效果,采用理论的拉曼增强因子(FEF)来表征,其表达式为
式中:
进一步,为了研究金银纳米粒子的间隙、直径以及锥形光纤的锥尖角度对局域电场增强的影响,先对粒子间隙(固定锥形光纤角度为5°,金属纳米粒子直径49 nm,粒子间隙取2、5、6.8、8、10 nm)进行扫描,再对金属纳米粒子直径(固定锥形光纤角度为5°,金属纳米粒子间隙6.8 nm,粒子直径取20、30、49、60、80 nm)进行扫描,最后对锥形光纤角度(固定金属纳米粒子间隙6.8 nm,粒子直径49 nm,锥形光纤角度取3°、4°、5°、8°、10°)进行扫描,得到的最大电场强度如
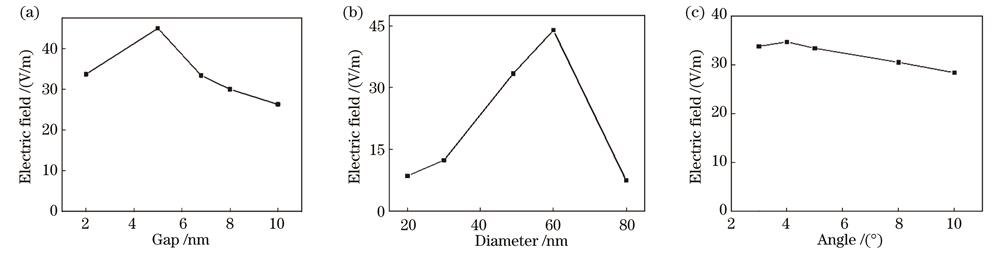
图 5. 最大电场强度图。(a)不同粒子间隙;(b)不同粒子直径;(c)不同锥形光纤角度
Fig. 5. The maximum electric field intensity maps. (a) Different gaps; (b) different diameters; (c) different angles
3.4 拉曼实验
3.4.1 最低检测浓度
为了探究双金属光纤SERS探针的增强效应,采用R6G作为探针分子并利用上述测试方法进行拉曼测试,结果如
式中:
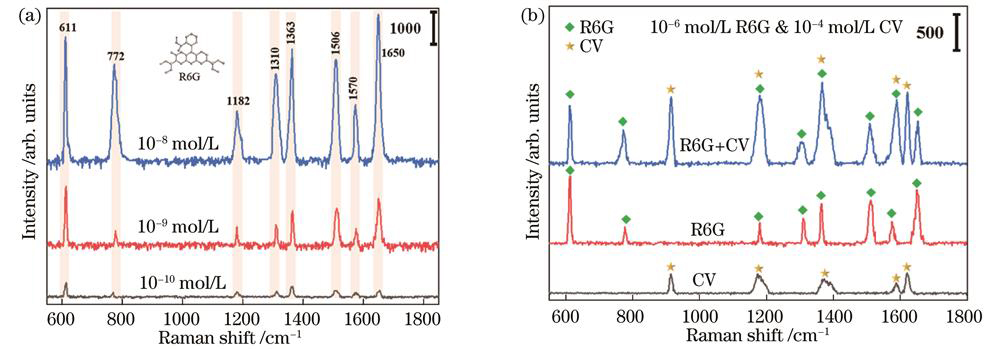
图 6. 拉曼实验结果。(a)R6G测试结果;(b)R6G和CV混合物测试结果
Fig. 6. Raman measurements. (a) Test results of R6G ; (b) test results of R6G and CV mixture
3.4.2 多分子检测
为了验证样品对非单一分子的检测效果,使用10-6 mol/L的R6G和10-4 mol/L的CV混合溶液作为探针分子进行实验,结果如
3.4.3 样品时效性测试
为了研究所提双金属光纤SERS探针的时效性(稳定性),将制备的双金属光纤探针放置24 h、48 h、72 h、96 h后,对10-7mol/L R6G的拉曼测试结果进行比较,结果如
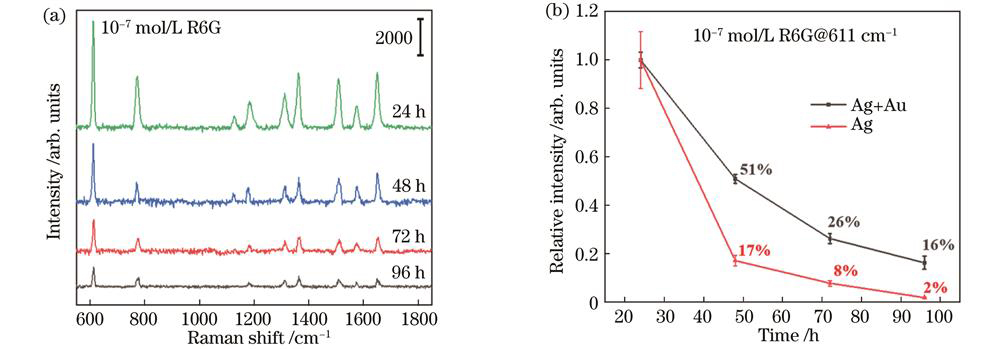
图 7. 时效性实验。(a)双金属光纤SERS探针的时效性测试结果;(b)单金属和双金属光纤SERS探针611 cm-1的峰强随时间变化的比较
Fig. 7. Time-sensitive experiments. (a) Results of bimetallic SERS probes; (b) relative results of single Ag and bimetallic SERS probes at 611 cm-1 with R6G as analyte
取611 cm-1的拉曼强度,其变化趋势作如
4 结 论
提出一种新型双金属纳米粒子修饰锥形光纤SERS探针,实现了对低浓度R6G的检测和多分子的识别,该样品具有良好的SERS增强性能(FAEF=2.07×108)和稳定性(96 h后,双金属光纤SERS 探针稳定性是单金属的8 倍)。该双金属锥形光纤SERS探针未来有望在原位和远程检测方面得到应用。下一步研究方向是探索可控的双金属修饰光纤关键工艺,以进一步优化样品的检测灵敏度和稳定性。
[1] Müller Molnár C, Cintă Pînzaru S, Chis V, et al. SERS of cylindrospermopsin cyanotoxin: prospects for quantitative analysis in solution and in fish tissue[J]. Spectrochimica Acta Part A: Molecular and Biomolecular Spectroscopy, 2023, 286: 121984.
[2] Kanioura A, Geka G, Kochylas I, et al. SERS determination of oxidative stress markers in saliva using substrates with silver nanoparticle-decorated silicon nanowires[J]. Biosensors, 2023, 13(2): 273.
[3] Yang C P, Kao W Y, Yu S H, et al. Environmentally friendly etchant of in situ plasmon-activated water to improve SERS sensing of pesticides[J]. Sensors and Actuators B: Chemical, 2023, 374: 132798.
[4] Ge K, Huang Y H, Zhang H Q. Fabrication of hierarchical β-Bi2O3/AuAg microspheres for sensitive, selective and rapid detection of environment pollutants by surface-enhanced Raman spectroscopy[J]. Spectrochimica Acta Part A: Molecular and Biomolecular Spectroscopy, 2023, 285: 121907.
[5] Wang H Q, Liu M J, Zhao H M, et al. Rapid detection and identification of fungi in grain crops using colloidal Au nanoparticles based on surface-enhanced Raman scattering and multivariate statistical analysis[J]. World Journal of Microbiology and Biotechnology, 2023, 39(1): 26.
[6] Wang X J, Zhu X P, Tao Y F, et al. ZnO nanorods decorated with Ag nanoflowers as a recyclable SERS substrate for rapid detection of pesticide residue in multiple-scenes[J]. Spectrochimica Acta Part A: Molecular and Biomolecular Spectroscopy, 2023, 290: 122277.
[7] Fleischmann M, Hendra P J, McQuillan A J. Raman spectra of pyridine adsorbed at a silver electrode[J]. Chemical Physics Letters, 1974, 26(2): 163-166.
[8] Gao C B, Hu Y X, Wang M S, et al. Fully alloyed Ag/Au nanospheres: combining the plasmonic property of Ag with the stability of Au[J]. Journal of the American Chemical Society, 2014, 136(20): 7474-7479.
[9] Bian X Y, Zhang G Y, Liu B, et al. One-pot synthesis of Au/Ag alloy@SiO2 core-shell nanoparticles and their metal-enhanced fluorescence and surface-enhanced Raman scattering spectroscopies[J]. Journal of Nanoparticle Research, 2022, 24(2): 20.
[10] Shinki, Sarkar S. Au0.5Ag0.5 alloy nanolayer deposited on pyramidal Si arrays as substrates for surface-enhanced Raman spectroscopy[J]. ACS Applied Nano Materials, 2020, 3(7): 7088-7095.
[11] Li H Y, Zhang J L, Jiang L L, et al. Chiral plasmonic Au-Ag core shell nanobipyramid for SERS enantiomeric discrimination of biologically relevant small molecules[J]. Analytica Chimica Acta, 2023, 1239: 340740.
[12] Li T, Yu Z N, Wang Z K, et al. Optimized tapered fiber decorated by Ag nanoparticles for Raman measurement with high sensitivity[J]. Sensors, 2021, 21(7): 2300.
[13] Chen Z Y, Dai Z M, Chen N, et al. Gold nanoparticles-modified tapered fiber nanoprobe for remote SERS detection[J]. IEEE Photonics Technology Letters, 2014, 26(8): 777-780.
[14] Yu Z N, Wang Z K, Zhang J E. Preparation optimization for a silver cavity coupled tapered fiber SERS probe with high sensitivity[J]. Optical Materials Express, 2022, 12(7): 2835-2843.
[15] Liu Y, Liu R M, Ai C W, et al. Stick-slip-motion-assisted interfacial self-assembly of noble metal nanoparticles on tapered optical fiber surface and its application in SERS detection[J]. Applied Surface Science, 2022, 602: 154298.
[16] Cao J, Zhao D, Mao Q H. A highly reproducible and sensitive fiber SERS probe fabricated by direct synthesis of closely packed AgNPs on the silanized fiber taper[J]. Analyst, 2017, 142(4): 596-602.
[17] Sha H Y, Wang Z K, Zhang J E. SiO2 microsphere array coated by Ag nanoparticles as Raman enhancement sensor with high sensitivity and high stability[J]. Sensors, 2022, 22(12): 4595.
[18] 田茂杰, 向小芳. 合成不同粒径大小的金纳米粒子的分析研究[J]. 浙江化工, 2018, 49(3): 32-34.
Tian M J, Xiang X F. Analysis and study on the synthesis of gold nanoparticles with different particle sizes[J]. Zhejiang Chemical Industry, 2018, 49(3): 32-34.
[19] Kaur N, Trevisanutto J O, Das G. Tweezing and manipulating the distribution of gold nanorods (GNRs) on a tapered optical fiber to develop a plasmonic structure[J]. OSA Continuum, 2020, 3(9): 2415-2422.
[20] Trevisanutto J O, Linhananta A, Das G. Plasmonic structure: fiber grating formed by gold nanorods on a tapered fiber[J]. Optics Letters, 2016, 41(24): 5789-5792.
[21] Kaur N, Trevisanutto J O, Das G. Plasmonic structure on a tapered optical fiber for application as a surface-enhanced Raman spectroscopy substrate[J]. Microwave and Optical Technology Letters, 2021, 63(11): 2776-2781.
[22] Zhou F, Liu Y, Wang H C, et al. Au-nanorod-clusters patterned optical fiber SERS probes fabricated by laser-induced evaporation self-assembly method[J]. Optics Express, 2020, 28(5): 6648-6662.
Article Outline
黄博, 汪正坤, 朱永, 张洁. 双金属锥形光纤表面增强拉曼散射探针拉曼增强特性[J]. 光学学报, 2023, 43(21): 2106003. Bo Huang, Zhengkun Wang, Yong Zhu, Jie Zhang. Raman Enhancement Characteristics of Bimetallic Tapered Optical Fiber Surface-Enhanced Raman Scattering Probe[J]. Acta Optica Sinica, 2023, 43(21): 2106003.