亚音速切向气流下连续激光对玻璃纤维增强树脂基复合材料的穿孔效应
Glass fiber reinforced polymer (GFRP) with excellent wave-transparent properties are the preferred choice for optoelectronic devices and microwave dielectric materials owing to their high strength, light weight, and excellent electrical properties. Traditional cutting techniques for processing GFRP have problems such as severe tool wear, low efficiency, and low accuracy. The application of laser processing can solve these problems and has broader application prospects. However, some problems remain in the processing of reduced materials by a single laser beam, such as the shielding of the subsequent laser by the pyrolysis gas, transformation of the target absorption mode due to incomplete pyrolysis of the residual carbon, and irregularity of the ablation morphology. The laser processing assisted by tangential air flow can solve these problems and improve the efficiency of material processing. In this study, a detailed investigation on the target perforation time, ablation morphology, and temperature distribution on the ablation surface under different power densities and tangential air flow was carried out. These results are helpful for improving the processing efficiency and profile of GFRP.
A fiber laser (wavelength of 1070 nm) with a maximum output power of 20 kW was used to interact with the GFRP in a relatively confined metal target chamber. Tangential air flow was provided by an air compressor and flowed out through a nozzle, and the air flow rate was measured using the Pitot tube method. A manometer was used to measure the pressure of the tangential air flow output from the nozzle; therefore, the stability of the air flow was monitored. The range of air flow rate used in the experiment was 0-1.0 Ma. The temperature evolution from the front and rear surfaces of the target was recorded using an infrared thermometer imager. The temperature data of the perforation point was extracted to draw a temperature change curve with time, and the perforation time was obtained from the falling edge of the temperature curve.
The perforation effects of GFRP are investigated at different laser power densities (848-1556 W/cm2) and tangential air flow velocities (0-1.0 Ma). It is found that the effect of increasing the laser power density on the ablation rate of GFRP is more significant than that of varying the tangential air flow rate (Figs.4 and 8). With an increase in the tangential air flow rate, the perforation time shows a decreasing trend and then a slow increase (Fig.8). This behavior is related to three effects caused by the tangential air flow: reducing the surface residual carbon content to promote the bulk absorption of the target (Fig.5), enhancing the heat convection on the target surface to accelerate the cooling (Fig.7), and providing tangential shear force to produce a mechanical erosion effect.
The perforation effect of GFRP at different laser power densities (848-1556 W/cm2) and tangential air flow rates (0-1.0 Ma) is investigated using a continuous laser with a wavelength of 1070 nm. The experimental results show that the perforation time decreases with increasing power density. A large amount of pyrolysis gas is generated in a shorter time period at higher power densities, which further results in a higher pore pressure and promotes the exfoliation process of the target. The effects of tangential air flow on the GFRP perforation process include reducing the surface residual carbon content to promote the bulk absorption process of the target, enhancing the cooling effect on the target surface, and providing a tangential shear force to produce a mechanical erosion effect. The three effects caused by tangential air flow have an obvious competitive relationship in the perforation process of the target. For the laser power density of 848 W/cm2, when the tangential air flow rate is ≤ 0.4 Ma, the effect of tangential air flow is mainly used to promote resin pyrolysis, reduce the residual carbon content, and change the target absorption mode. Hence, the target perforation time decreases with an increase in the air flow rate. When the tangential air flow rate is in the range of 0.8-1.0 Ma, the cooling effect is more obvious. Therefore, the perforation time of the target material increases slowly with an increase in the air flow rate. In addition, compared with the tangential air flow rate, the effect of the power density on the perforation time of the GFRP is more significant.
1 引言
复合材料因为轻质量、低成本、易成型、高比强度和比模量、高抗疲劳性和抗腐蚀性、低导热系数和低膨胀系数等优势被广泛运用在航空航天、交通运输、电子产品、医疗器具、体育器材、工业建筑等方面[1]。其中,透波性能优异的玻璃纤维增强树脂基复合材料(GFRP)因为强度高、质量轻和电性能优异而成为光电子学器件、微波介电材料和低马赫飞行器天线罩材料的首选[2]。随着高能激光技术的发展,研究激光对玻璃纤维增强树脂基复合材料的作用机理具有十分重要的意义。
国内外研究者对激光作用下复合材料的烧蚀机理进行了大量的研究。Henderson等[3]实验探究了高温下复合材料的热物性参数的变化规律,根据大量实验数据得到了复合材料的导热系数随温度的变化规律。Dimitrienko等[4-5]研究了高温下复合材料的温度场、热应力分布,发现高温下树脂热解碳化产生的大量气体会使靶材的力学性能发生显著的变化。梁晗等[6]基于各向异性传热原理研究了激光扫描角度对复合材料去除率的影响规律,结果表明,激光扫描角度越大,预热效应越弱,去除效率越低,去除质量越差。郭亚林等[7]利用CO2激光器研究了不同激光参数下硅基复合材料的质量损失速率,实验发现靶材的质量损失速率受入射激光强度和光斑直径的影响较大,受辐照时间的影响较小。彭国良等[8-9]通过仿真计算发现力学剥蚀极大地降低了GFRP穿孔所需的能量,激光功率密度较低时靶材烧蚀效率较高。相对于金属材料,激光作用于树脂基类复合材料时具有明显不同的能量耦合过程,初始阶段的能量耦合率较低,材料以沉积式的体吸收为主,随着材料热解的进行,大量不完全热解的残炭附着在材料表层,只有较少的能量到达材料内部,此时材料的吸收方式则以面吸收为主[10-12]。
激光作用于GFRP时外部气流的存在会对靶材的烧蚀机制产生极大的影响。陈敏孙等[13-14]实验发现:一方面,切向空气流的加载会削弱热解气体对激光的屏蔽作用,提供充足的氧气助燃,加速树脂基体的热解;其次,切向气流的存在会加强对流换热,进而加速材料表面的冷却。此外,气流的流速、种类都会对材料的质量损失、温度分布以及材料的加工效率产生一定的影响[15-18]。
激光去除材料具有非接触、效率高、加工精度高等其他加工技术无法比拟的诸多优势,目前已被广泛应用于激光打孔、激光切割、激光烧蚀、激光光刻等领域[19]。目前国内外关于激光烧蚀复合材料的研究主要集中于材料的质量损失速率、烧蚀形貌、力学性能变化、靶材的烧蚀机制转变等,对切向空气流作用下材料的穿孔效应的研究较少。本文通过实验研究了切向空气流作用下连续激光(波长为λ=1070 nm)辐照GFRP的穿孔效应,分析了不同激光功率密度和不同切向空气流速下GFRP的温度分布、烧蚀形貌和穿孔规律。
2 实验装置
实验装置如
采用上述实验装置分别研究切向空气流速不变(0.5 Ma)时激光功率密度(848~1556 W/cm2)以及激光功率密度不变(848 W/cm2)时切向空气流速(0~1.0 Ma)对材料烧蚀形貌、温度场分布和穿孔时间的影响。每组参数下进行三次重复实验以确保实验数据的准确性。
3 实验结果与分析
3.1 激光功率密度对GFRP穿孔的影响
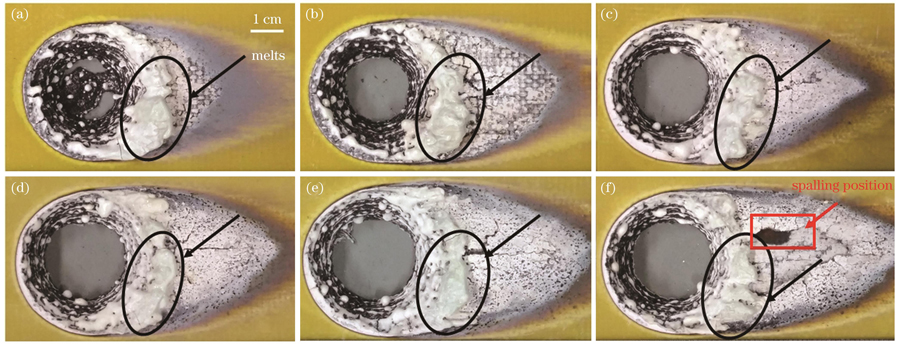
图 2. 切向空气流速为0.5 Ma时不同激光功率密度下的GFRP烧蚀形貌。(a)848 W/cm2;(b)990 W/cm2;(c)1131 W/cm2;(d)1273 W/cm2;(e)1414 W/cm2;(f)1556 W/cm2
Fig. 2. Ablation morphologies of GFRP under different laser power densities at tangential air flow rate of 0.5 Ma. (a) 848 W/cm2;(b) 990 W/cm2; (c) 1131 W/cm2; (d) 1273 W/cm2; (e) 1414 W/cm2; (f) 1556 W/cm2
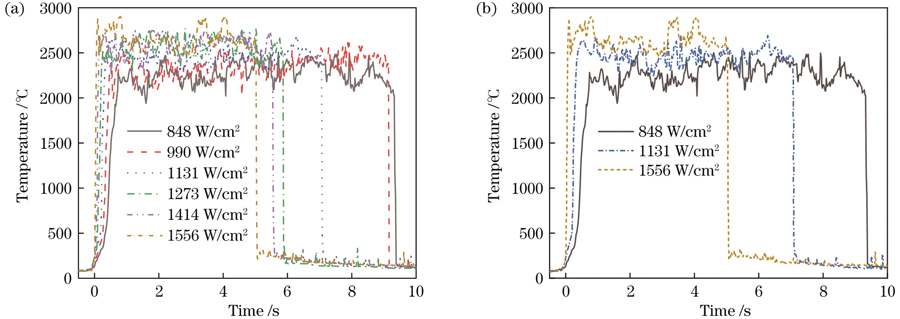
图 3. 切向空气流速为0.5 Ma时的GFRP穿孔点温升曲线。(a)激光功率密度为848~1556 W/cm2;(b)图3(a)的局部放大
Fig. 3. GFRP perforation point temperature rise curves when tangential air flow rate is 0.5 Ma. (a) Laser power density is 848-1556 W/cm2; (b) partial enlargement of Fig. 3 (a)
切向空气流速为0.5 Ma时GFRP穿孔时间随激光功率密度的变化如
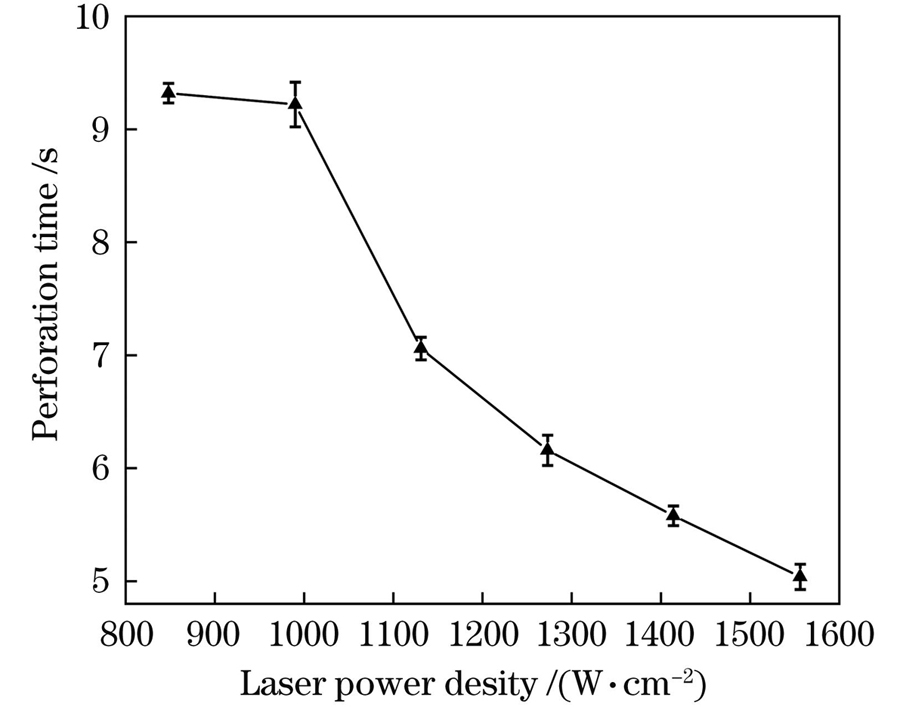
图 4. 空气流速为0.5 Ma不同功率密度作用下GFRP的穿孔时间
Fig. 4. Perforation time of GFRP under different power densities with air flow rate of 0.5 Ma
3.2 切向空气流速对GFRP穿孔的影响
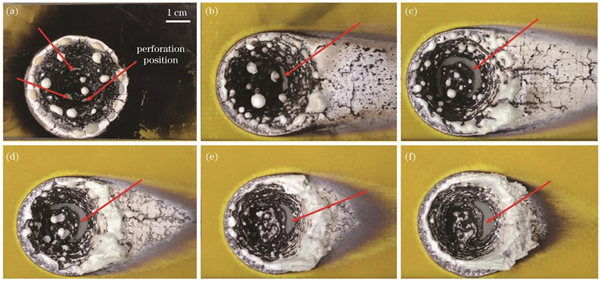
图 5. 激光功率密度为848 W/cm2时不同切向空气流速下的GFRP烧蚀形貌。(a)0 Ma;(b)0.2 Ma;(c)0.4 Ma;(d)0.6 Ma;(e)0.8 Ma;(f)1.0 Ma
Fig. 5. Ablation morphologies of GFRP under different tangential air flow rates with laser power density of 848 W/cm2. (a) 0 Ma;(b) 0.2 Ma; (c) 0.4 Ma; (d) 0.6 Ma; (e) 0.8 Ma; (f) 1.0 Ma
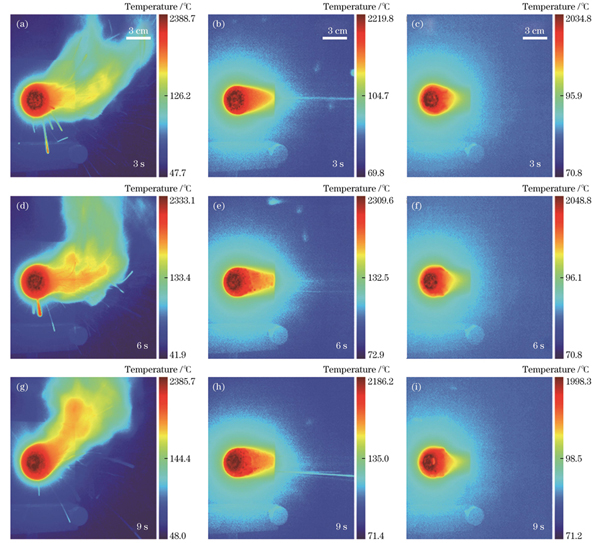
图 6. 激光功率密度为848 W/cm2时不同时刻的GFRP表面温度分布。(a)0 Ma;(b)0.4 Ma;(c)1.0 Ma
Fig. 6. Temperature distributions on GFRP surface under different moments with laser power density of 848 W/cm2. (a) 0 Ma;(b) 0.4 Ma; (c) 1.0 Ma
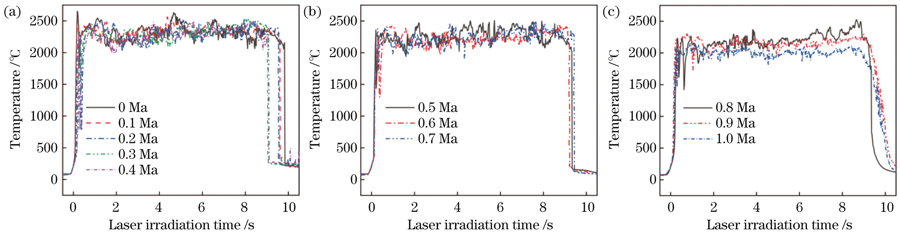
图 7. 激光功率密度为848 W/cm2时不同切向空气流速下的GFRP穿孔点温升曲线。(a)0~0.4 Ma;(b)0.5~0.7 Ma;(c)0.8~1.0 Ma
Fig. 7. Temperature rise curves of GFRP perforation point under different tangential air flow rates with laser power density of 848 W/cm2. (a) 0-0.4 Ma; (b) 0.5-0.7 Ma; (c) 0.8-1.0 Ma
激光功率密度为848 W/cm2时GFRP穿孔时间随切向空气流速的变化如
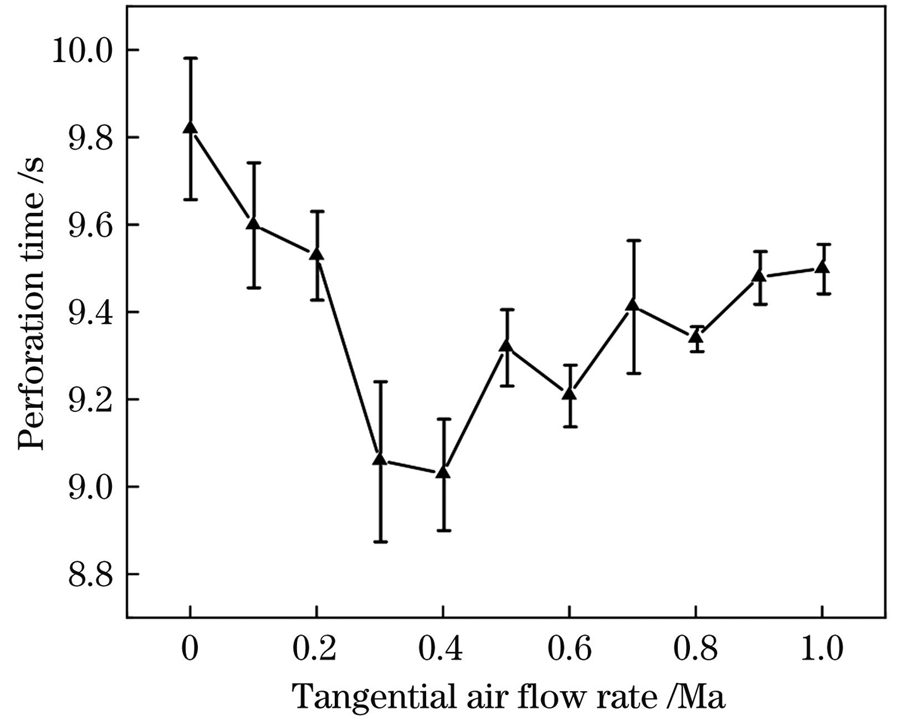
图 8. 激光功率密度为848 W/cm2时不同切向空气流速下GFRP的穿孔时间
Fig. 8. Perforation time of GFRP under different tangential air flow rates with laser power density of 848 W/cm2
流速改变导致穿孔时间差最大仅为0.78 s,相较于功率密度带来的时间差较小,因为GFRP穿孔主要是由靶材孔隙压力升高产生的层状剥蚀造成的,高功率密度下靶材能够达到更高的温度,GFRP在较短时间内产生大量热解气体,靶材孔隙压力升高。而切向空气流速的改变对温度的影响较小,仅当流速≥0.8 Ma时才出现较明显的温度波动。流速引起的改变更多表现在改变靶材吸收方式和切向剪切力大小,对穿孔时间的影响较小。
4 结论
采用波长为1070 nm的连续激光对不同激光功率密度(848~1556 W/cm2)和切向空气流速(0~1.0 Ma)下的GFRP穿孔效应进行了研究。实验结果表明:穿孔时间随功率密度的增加而下降,主要原因是在高功率密度下靶材更容易在较短时间内生成大量热解气体,进而产生较高的孔隙压力,促进了靶材的剥蚀过程。切向空气流对GFRP穿孔过程的影响包括:降低表面残炭含量,促进靶材的体吸收过程;加速靶材表面的对流换热;提供切向剪切力,产生力学剥蚀效应。切向空气流带来的上述三种影响在靶材的穿孔过程中存在着较明显的竞争关系。在激光功率密度为848 W/cm2的情况下,当切向空气流速≤0.4 Ma时,切向气流主要表现为促进树脂热解,降低残炭含量,转变靶材吸收方式,此时靶材穿孔时间随流速的增加呈现下降趋势;当切向空气流速处于0.8~1.0 Ma区间时,气流的冷却作用表现得较为明显,靶材的穿孔时间随流速的增加呈现缓慢上升的趋势。此外,与切向气流速度相比,功率密度的改变对GFRP穿孔时间的影响更为显著。
[1] 吴良义. 先进复合材料的应用扩展: 航空、航天和民用航空先进复合材料应用技术和市场预测[J]. 化工新型材料, 2012, 40(1): 4-9, 91.
Wu L Y. The application extend of advanced composite materials: technology markets of ACM application in aeronautics, astronautics and civil aviation[J]. New Chemical Materials, 2012, 40(1): 4-9, 91.
[2] 张加波, 张开虎, 范洪涛, 等. 纤维复合材料激光加工进展及航天应用展望[J]. 航空学报, 2022, 43(4): 132-153.
Zhang J B, Zhang K H, Fan H T, et al. Progress in laser processing of fiber composite materials and prospects of its applications in aerospace[J]. Acta Aeronautica et Astronautica Sinica, 2022, 43(4): 132-153.
[3] Henderson J B, Wiebelt J A, Tant M R. A model for the thermal response of polymer composite materials with experimental verification[J]. Journal of Composite Materials, 1985, 19(6): 579-595.
[4] Dimitrienko Y I. Internal heat-mass transfer and stresses in thinwalled structures of ablating materials[J]. International Journal of Heat and Mass Transfer, 1997, 40(7): 1701-1711.
[5] Dimitrienko Y I. Thermomechanical behaviour of composite materials and structures under high temperatures: 1. materials[J]. Composites Part A: Applied Science and Manufacturing, 1997, 28(5): 453-461.
[6] 梁晗, 赵树森, 姜璐, 等. 碳纤维复合材料激光热-机械剥蚀机制研究[J]. 中国激光, 2022, 49(10): 1002405.
[7] 郭亚林, 梁国正, 丘哲明, 等. 激光参数对碳纤维复合材料质量烧蚀率的影响[J]. 复合材料学报, 2006, 23(5): 84-88.
Guo Y L, Liang G Z, Qiu Z M, et al. Effect of laser parameters on mass ablative rate of carbon fiber reinforced composite[J]. Acta Materiae Compositae Sinica, 2006, 23(5): 84-88.
[8] 彭国良, 张相华, 高银军, 等. 激光辐照玻璃纤维/环氧树脂复合材料的力学剥蚀研究[J]. 中国激光, 2015, 42(1): 0106001.
[9] 陈敏孙, 江厚满, 刘泽金. 玻璃纤维/环氧树脂复合材料热分解动力学参数的确定[J]. 强激光与粒子束, 2010, 22(9): 1969-1972.
[10] 李雅娣, 吴平, 马喜梅, 等. 碳纤维/环氧树脂复合材料层板连续激光烧蚀试验研究[J]. 纤维复合材料, 2010, 27(2): 21-24.
Li Y D, Wu P, Ma X M, et al. The tests of carbon fiber/epoxy laminated composites under continuous laser irradiation[J]. Fiber Composites, 2010, 27(2): 21-24.
[11] 彭国良, 杜太焦, 刘峰, 等. 激光烧蚀玻璃纤维/环氧树脂复合材料的能量耦合率模拟研究[J]. 中国激光, 2014, 41(2): 0203001.
[12] Chippendale R D, Golosnoy I O, Lewin P L. Numerical modelling of thermal decomposition processes and associated damage in carbon fibre composites[J]. Journal of Physics D: Applied Physics, 2014, 47(38): 385301.
[13] 陈敏孙. 切向气流作用下激光对纤维增强树脂基复合材料的辐照效应研究[D]. 长沙: 国防科学技术大学, 2012. 10.1117/12.2011232
ChenM S. Research on the laser irradiation effects on fiber reinforced resin composites subjected to tangential gas flow[D]. Changsha: National University of Defense Technology, 2012.
[14] 陈敏孙, 江厚满, 焦路光, 等. 切向气流作用下玻璃纤维复合材料的激光辐照效应[J]. 强激光与粒子束, 2013, 25(5): 1075-1080.
[15] 彭国良, 张相华, 高银军, 等. 气流环境中碳纤维/环氧树脂复合材料烧蚀羽烟对激光透射率的影响[J]. 中国激光, 2015, 42(2): 0206004.
[16] 高贺, 张智, 陈连忠, 等. 硅基防热材料表面流动特性研究[J]. 空气动力学学报, 2019, 37(3): 406-411.
Gao H, Zhang Z, Chen L Z, et al. Surface flow character study of silicone thermal protection material[J]. Acta Aerodynamica Sinica, 2019, 37(3): 406-411.
[17] 张检民, 马志亮, 冯国斌, 等. 切向空气流速度对玻璃纤维增强树脂基复合材料激光烧蚀热的影响[J]. 中国激光, 2015, 42(3): 0306004.
[18] 贺佳, 张黎, 张永强, 等. 表面气流环境下激光辐照碳纤维复合材料实验研究[J]. 应用激光, 2014, 14(2): 118-121.
He J, Zhang L, Zhang Y Q, et al. The test of laser ablation of carbon fiber composites materials in airflow environment[J]. Applied Laser, 2014, 14(2): 118-121.
[19] 胡晓冬, 李元龙, 白少状, 等. 激光在材料去除加工中应用的研究进展[J]. 激光与光电子学进展, 2021, 58(5): 0500008.
Hu X D, Li Y L, Bai S Z, et al. Research progress of laser application in material removal[J]. Laser&Optoelectronics Progress, 2021, 58(5): 0500008.
[20] 时圣波, 梁军, 刘志刚, 等. 高硅氧/酚醛复合材料烧蚀环境下的吸热机理[J]. 固体火箭技术, 2013, 36(1): 113-118.
Shi S B, Liang J, Liu Z G, et al. Endothermic mechanism of silica/phenolic composite under ablative environment[J]. Journal of Solid Rocket Technology, 2013, 36(1): 113-118.
[21] 徐善成. 切向空气流下连续激光辐照玻璃纤维增强树脂基复合材料烧蚀特性的研究[D]. 南京: 南京理工大学, 2020.
XuS C. Study on ablation characteristics of glass fiber reinforced resin matrix composites by CW laser irradiation under tangential air flow[D]. Nanjing: Nanjing University of Science and Technology, 2020.
[22] 张晓腾, 李泽文, 周义青, 等. 在亚音速气流作用下连续激光辐照铝合金的穿孔效应[J]. 红外与激光工程, 2022, 51(2): 20210883.
[23] Nan P Y, Li X, Pan Y X, et al. Shift of the laser ablation center on CFRP induced by the vortex in the boundary layer of tangential airflow[J]. The International Journal of Advanced Manufacturing Technology, 2020, 106(5): 2523-2533.
陈庚, 唐杰, 周义青, 潘云香, 张宏超, 李泽文, 沈中华, 陆健. 亚音速切向气流下连续激光对玻璃纤维增强树脂基复合材料的穿孔效应[J]. 中国激光, 2023, 50(14): 1401002. Geng Chen, Jie Tang, yiqing Zhou, Yunxiang Pan, Hongchao Zhang, Zewen Li, Zhonghua Shen, jian Lu. Perforation Effect of Continuous Laser on Glass Fiber Reinforced Polymer Matrix Composites Under Subsonic Tangential Airflow[J]. Chinese Journal of Lasers, 2023, 50(14): 1401002.