Light touch in quantum: an interview with Prof. Serge Haroche
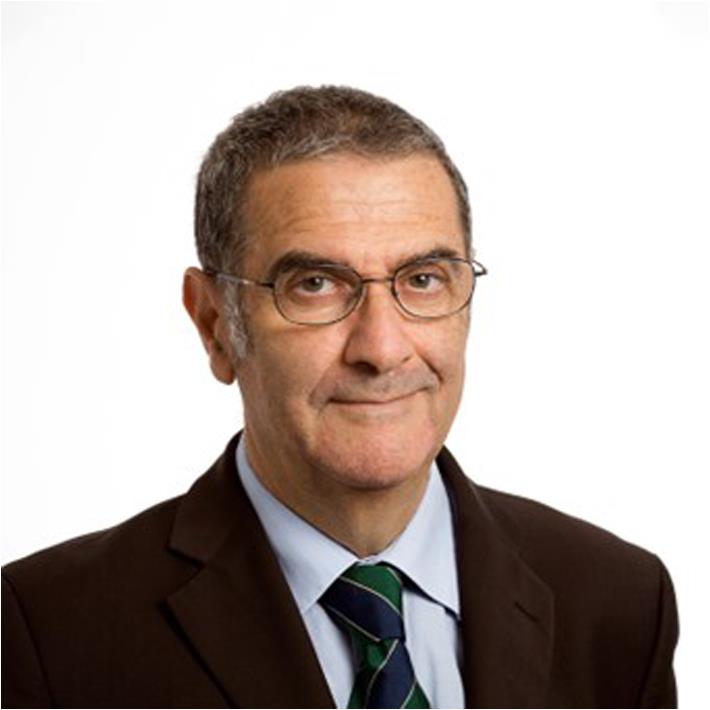
Fig. 1. Professor Serge Haroche, Collège de France and École Normale Supérieure, Paris, France
Hello, Prof. Haroche, nice to see you again! Let us start the interview. In 2012, you and David Wineland won the Nobel Prize in Physics. Can you describe your work in simple terms to a general audience? And can you tell us how you were originally interested in this field and got into this study?
Maybe I should start with the second part of the question. I got interested in atomic physics when I was a student at École Normale Supérieure (ENS) in Paris in the 1960s. My mentors, Alfred Kastler, Jean Brossel, and Claude Cohen-Tannoudji, were outstanding physicists and very charismatic teachers. Their lectures introduced me to atomic physics, a domain in which light is used to investigate the properties of atoms. I found that the world of atoms was fascinating because it was obeying the very counterintuitive rules of quantum physics. The first experiments I did were performed with classical spectral lamps. Soon we were able to replace them with laser light, whose special features—monochromaticity, high intensity—opened novel ways to manipulate atoms. At the beginning of my career, we were working with a huge number of atoms: our samples were contained in glass cells with billions of atoms inside. I was intrigued by the fact that each of these atoms obeyed quantum laws, but the strange features of these laws were hidden by statistical effects, by the fact that we were working with a huge collection of atoms. Starting in the 1970s, my goal became to try to carry out experiments involving a smaller and smaller number of atoms. The ultimate goal was to investigate effects occurring when you had only one atom in the system, a situation in which quantum physics can be directly observed in action.
I was working with atoms and photons in a cavity, a box with highly reflecting mirror walls able to store microwave photons for a long time, of the order of a tenth of a second. It took my research group a long time to build such a cavity, using superconducting materials to achieve the high reflectivity required for the mirrors. We also had to engineer special atoms which were strongly interacting with microwave photons in the cavity. These were very exciting species, called Rydberg atoms, in which one electron is orbiting at a large distance from the atomic core. Laser beams are used to prepare these atomic excited states which behave as antennas extremely sensitive to microwave fields. In the end, we came to the situation where a single atom crossing the cavity was interacting with a single photon, which is the simplest kind of light–matter interaction you can think of.
We then started to observe what happens in this simple situation. We studied many phenomena which had been predicted by the founders of quantum physics a hundred years ago, but which could not have been observed before, because they were veiled in large samples by statistical effects. A measurement on a Rydberg atom after it had exited the cavity had an immediate effect on the state of the field left behind, a phenomenon called “quantum entanglement.” We could also prepare field states made of a few photons which had at the same time two different phases, a situation reminding us of the famous cat that Schrödinger had imagined to be at the same time alive and dead after having interacted with a single atom. We were also able to see a photon without destroying it, a feat called “quantum non-demolition (QND) measurement” which had never been done before. Usually, the detection of light destroys the photons, whereas in QND experiments the photons leave a subtle imprint on an atom without being annihilated.
At that time, we did these experiments motivated by curiosity, because we wanted to know how far we could dive into the microscopic world of atoms and photons, how precisely we could manipulate these microscopic entities without destroying them. Since then, it has become a field of research called quantum information science. At the beginning, I can say that we were doing quantum information without knowing it. We were just doing these experiments for fun and as a challenge. Schrödinger had famously said in 1952 that we would never be able to experiment with a single electron or a single atom and he had added that if it were possible, it would have ridiculous consequences. When we embarked on our experiments, we were motivated by the challenge to prove Schrödinger wrong. Scientists like Einstein and Schrödinger are famous because they have contributed so much to modern physics. To challenge some of their statements or ideas was a very exciting aspect of our work.
I must add that my group was far from being the only one to work in the field of manipulating single quantum objects. Other groups, instead of trapping photons in cavities, developed traps for charged atoms, so-called ion traps, and observed effects similar to the ones we studied with our system, namely, state superpositions, quantum entanglement, Schrödinger-cat-like states, etc… David Wineland was heading one of the pioneering group in ion trap physics in Boulder, Colorado. I am very glad to have shared the Nobel Prize with David, because he has been a longtime friend and colleague. We did not work with the same system, but we explored the same problems from two different angles. He was probing ions with light, and I was probing light with atoms.
Your supervisor and your supervisor’s supervisor, sometimes we call him your “grand supervisor,” all won a Nobel Prize. How do you feel about this extraordinary academic tree? Is there any interesting story you wish to share with young researchers?
I have been very lucky in my scientific career. It has been really a matter of luck to join the physics lab in which I was trained as a young graduate student. ENS, the institution to which this lab belongs, is an institution where a lot of very good research in science and studies in humanities have been achieved over a long time period. The ratio of Nobel Prizes per alumnus is very large at ENS, which admits less than a hundred students per year and counts since the beginning of the 20th century a dozen Nobel laureates in physics, chemistry, and literature, not including the prizes in economics. The atmosphere in the physics lab in which I graduated and did subsequently the essentials of my research, which is now called the Kastler–Brossel Laboratory, was—and still is—a fantastic place. The professors were charismatic and enthusiastic teachers. They trusted the young students and gave them all the freedom and environment needed to develop their creativity and imagination.
In this lab, Kastler and his student and colleague Brossel invented the optical pumping method, in which light beams are employed to change and control the internal state of atoms. The experiments were originally done on large macroscopic samples with classical spectral lamps of weak intensity. There were no lasers when the method was invented, but the idea of optical pumping blossomed once lasers came of age in the 1960s. The principle, initiated by Kastler, of manipulating atoms with light, inspired all my subsequent research work. The invention of lasers, extremely monochromatic, directive, and intense light sources, has tremendously expanded the experimental possibilities. I can say that I have benefitted from double luck: to be trained in such an inspiring laboratory and to have started my career in physics at the time an extraordinary source of light had been invented.
I learned that Kastler had won the Nobel Prize on a day in October 1966, when I was working in the lab, and the moment was captured in a photo. I usually show it in my talks, and it can be found in my Nobel Lecture published in the Reviews of Modern Physics. This was a fantastic day. The only regret we had was that the prize had not been split between Kastler and Brossel. Kastler has said many times that he would have preferred to share it with his former student and colleague. Kastler had the original idea, but Brossel built the special lamps and atomic cells which were used to perform the experiments. Brossel was not only a gifted experimentalist. He was also a real gentleman because he never complained nor said anything about the fact that he did not get the prize. I still do not know why he did not share it with Kastler.
I think that things are changing. Nowadays, Nobel Prizes tend to be given to junior people.
Yes, the Nobel committee has since then tried to correct some errors. In spite of our regret, the announcement of Kastler’s prize was nevertheless a fantastic event. I still remember my first contact with journalists. They flocked the lab on that occasion. One of them insisted on the idea that optical pumping and the laser were basically the same thing and we had a hard time telling him that Kastler had not invented the laser! I remember the discussion with this journalist, trying to convince him that he should not oversell what Kastler had done.
I heard that on the midnight of your 62nd birthday, you were still in the lab and, with your students, observed the birth and the death of a single photon for the first time. Can you share with us the excitement at that time?
We had for many years been trying to achieve the situation where you have a cavity containing only one photon, which is bouncing back and forth between the mirror, staying in it long enough to interact with many atoms crossing the cavity one by one. We knew that this could in principle be achieved but we needed an exceptionally good cavity for that. It took us several years to get it, after many trials and errors. Finally, in the summer of 2006, we obtained and tested a cavity with the required properties, and we were feverishly preparing the experiment.
I remember it all converged on my birthday, on September 11 of that year. We had a family party at home. Stefan Kuhr, a postdoc in our group, was working that night in the lab. He called me at 10pm, telling me that the experiment was ready. I told him “just wait, I am coming” and I left the party. Less than 10 minutes later, I arrived in the lab, in time to witness the long-expected signal. Hundreds of atoms were crossing the cavity one by one, each leaving its trace on a computer screen, all atoms agreeing that there was one photon between the mirrors. Suddenly, the photon disappeared in the walls of the cavity, then all the following atoms left a different signal, all agreeing that the cavity was empty. This signal change, a sudden quantum jump, was the kind of trace we had been looking for for many years and our feeling of exhilaration is difficult to describe. We stayed in the lab for a long time, watching with fascination the birth and death of photons in our cavity. There is a big difference between recognizing that you are doing a nice experiment and feeling that you have achieved really groundbreaking work.
In fact, with my colleague Jean-Michel Raimond, we had just finished writing a book called Exploring the Quantum: Atoms, Cavities and Photons in which we predicted what should be observed in this experiment. Unfortunately or fortunately, depending on how you see it, the book was finished and in press before the experiment, so it describes the non-destructive detection of photons and many other effects as theoretical projects which remained to be illustrated by real experiments. We have since kept telling ourselves that we should write a sequel to this book to describe these real experiments, but we still have not had the time to do it.
It sounds like the best birthday present. Maybe your experimental setup waited until your birthday to send you these quantum jump signals.
Maybe, but I want to add that it’s not only my experiment and my birthday present, it’s the experiment of all the people who were around on that night. I have a feeling which is a little bit similar to the one of Kastler. I think it would have been fair to share the prize with Jean-Michel Raimond and Michel Brune, my two senior coworkers. I mention in passing that Michel Brune is visiting Shanghai now.
Yes, he will visit us tomorrow at USTC (University of Science and Technology of China).
The work recognized by the Nobel Prize is a collective achievement. I have had during my career a long sequence of very bright and dedicated students and postdocs. Jean-Michel Raimond and Michel Brune have accompanied me during all this time and we made sure that each student had the possibility to contribute to our long term project by a nice piece of work during his or her PhD.
Speaking of collaborations, here is the next question. You have had some former students who have turned into long-term collaborators and colleagues. For example, Jean-Michel Raimond and Michel Brune. Actually, Michel is visiting China now. Somehow, I feel that the culture is in France slightly different from, for example, in the United States, where professors are usually single principal investigators. How does this collaboration work? How do you feel about it?
You are right. The tradition was different in France, at least at the time when our group formed. It was then possible to have several people staying together working on one big long-term project. In fact, Jean-Michel Raimond was one of my first students and he got a position at an early age at Paris VI University where he was teaching, while doing research with me at ENS. Then Michel Brune came 10 years later as a graduate student. He too stayed later in the group and got a senior scientist position at the CNRS (French National Center for Scientific Research). This allowed us to collaborate and to train generations of students and postdocs together. As I was the most senior person in the group, I had extra administrative tasks. Being for some years the chairman of the ENS physics department, I was then somewhat distracted from research activity, but I still could share with Michel and Jean-Michel the task of training the students and the postdocs in the lab. We discussed all our ideas and decided on the successive steps of our research together, in a friendly and very efficient collaboration.
As you mentioned, the situation is generally very different in the US universities where principal investigators are supposed to work independently. There are exceptions though. David Wineland at NIST (US National Institute of Standards and Technology) also worked with senior colleagues who could stay with him on permanent positions. The fact that NIST is not a university but a research institute has probably played a role to make such a situation possible in the US. I believe that such a collaborative work employing several senior scientists over a long time period is a very productive way of doing research, provided the co-principal investigators get along well with each other, and provided everyone gets enough recognition.
Unfortunately, such a situation has become more difficult to maintain, even in France. The fact that principal investigators (PIs) have to stand alone on a project has become the general rule. Research has become more expensive and cannot be carried out without applying for grants given by agencies that want to see, as a general rule, only one PI per project. Our group has had to adapt itself to that rule. Some of our students and postdocs have become very bright young investigators. We have made sure that each of them has their own independent project. I became a few years ago an emeritus professor, as well as Jean-Michel Raimond. Michel Brune is now the group leader and, in spite of the new rule of PI independence, he maintains to some degree the collaborative atmosphere of the early days, making sure that the students and postdocs working on different projects keep interacting and exchanging ideas with each other.
When you and Jean-Michel and Michel worked together, you also make more efficient use of the resources (for example, lasers), right? And I think that’s quite a good idea which we should promote.
Yes, we shared equipment of course and this is a big advantage of the collaborative work. We also shared the task of writing our papers together and I want to say a few words about that. We have kept very good memories about that time. We exchanged ideas, we tried to present the paper in the right way, with the right perspective, putting it in the context of what other people have done. Writing papers together is a very important aspect of collaborative work and contributes to making it successful.
As you have already mentioned, we feel that France has a very rich scientific tradition and, as a nation, has received about 70 Nobel Prizes in total. When you interact with Chinese researchers, and when you visit China, do you feel there’s any difference in the scientific culture between China and France? If you do, is there any advice you would give to the Chinese researchers and even maybe to some funding agency decision-makers?
I think I could also give some advice to the French decision makers, taking as an example some good aspects about how research is being carried out in China. I will come to this point later. But let me answer the first part of your question. There is indeed a long tradition of French excellence in science, especially in optics and related fields. Fresnel, Fizeau, and Foucault in the 19th century, and Fabry, Pérot, and Kastler in the 20th century have illustrated this tradition, which has survived until today. My prize in 2012 was preceded by that of Alfred Kastler in 1966 and that of my mentor and thesis advisor Claude Cohen-Tannoudji in 1997. Then, Gerard Mourou in 2017, Alain Aspect last year, and Pierre Agostini and Anne L’Huillier last October also won a Nobel Prize. All these prizes are related to optics. Anne L’Huillier had been a student in my graduate class at ENS and I have been very glad to see her outstanding laser work recognized at the highest level.
I would like to insist on one aspect of the way science is being done in France which I think is essential. Science is a part of a more general cultural activity. Science thrives in a context in which arts, humanities, philosophy, and literature are also blossoming, creating an atmosphere where creativity and imagination develop freely. In France and more generally in Europe, great scientists have been influenced by ideas coming from philosophers, writers, and artists. ENS alumni in philosophy, literature, and economics have got Nobel Prizes. The atmosphere of academic freedom which has nurtured the minds of these people has led other alumni to reach Nobel excellence in physics and chemistry. I think that achieving the highest standards in science cannot be separated from working in an environment in which other scholars reach the highest level of creativity in arts and humanities and this requires academic freedom. Since we are talking about Nobel Prizes in general, we should not forget the spirit in which these prizes have been created by Alfred Nobel. He wanted to recognize excellence for the good of humanity and he put at the same level the need to excel in science, but also in literature and in the defense of human values, via the Nobel Peace Prize. The spirit of the Nobel Prize is to celebrate all forms of creativity, not only on the side of “hard science.” This is something that China should take more into consideration, by opening more of the universities to humanities and allowing more exchanges between scientists and scholars in other areas of knowledge.
I would like to mention another aspect of science. In China and in other Asian countries, there is a tradition of hierarchy, requiring respect for senior people and highly valuing their wisdom. Having reached an old age myself, I cannot certainly complain about this! But this respect for seniority should not lead to the fact that old scientists control too much what young scientists want to do. The creativity of scientists is often at the highest at a young age. Junior people should be trusted and given more freedom in the academic world. I have the feeling that things are changing in the right direction now in China, but this evolution should go further.
Let me now turn to the advice I give to French policy-makers when I compare research in China and in France. I tell them to look at China, which gives much more money for science and research, especially in my domain of quantum physics and quantum information. And that’s good. It is essential to understand that the most important wealth for a country resides in the brains and imagination of young people and it is the duty of every country to nurture that wealth by giving to trusted young people the financial support required to achieve great things. I think it is essential for China, essential for Europe, and anywhere else in the world.
Let me mention a last point. I am worried today by the fact that the exchanges between scientists in China and in western countries including France are made more difficult by geopolitical tensions. I think that this is very unfortunate because science must be a global activity knowing no boundaries. Exchanges between scientists working in different environments and the healthy competition it induces are essential. And the challenges that science has to meet to solve the problems facing the planet and mankind are global. If we don’t try to face these challenges together, we are going towards a very difficult time.
Yes, very luckily, I think I myself and some of my colleagues actually carry some of the French science legacy. For example, the second president of our university (University of Science and Technology of China), Yan Jici, is the first Chinese person who obtained the PhD in physics from France. He was a student of Fabry, and when he returned to China, he contributed a lot to our university. Also, two of my colleagues and myself are recipients of the Fresnel Prize from EPS (European Physical Society).
Let’s move on. Because you are the pioneer of the cavity-QED as you have mentioned, can you describe to the general audience, the state of the field when you started, and its current state of the art? What are your predictions for future developments in the field?
When I started 40 years ago, I had no idea where my research would lead me. What we were doing was trying to do precise microwave spectroscopy of Rydberg atoms. We had a cavity in our experiments but it was there only to define the area of space where the microwave field was applied to the atoms. Then we realized that if we improved the quality of our cavity, we could get to the regime where one atom would become sensitive to a single photon. So, the idea to study light matter interaction at the level of single atom and single photon came progressively. This was the beginning of the domain called cavity quantum electrodynamics (QED). It became a very active sub-field of atomic physics, which was soon extended to the optical domain, microwave photons being replaced by optical ones and microwave cavities by very small Fabry–Pérot cavities with microscopic sizes.
Later on in this research, real atoms were replaced by artificial ones, superconducting quantum bits, interacting with radiofrequency cavities or waveguides. The method and the mathematics are the same as in cavity QED and this new domain had been called circuit QED, because the real atoms were replaced by superconducting circuits. Circuit QED had a lot of advantages, one of them being that the artificial atoms are manmade and can be produced by the techniques of lithographic deposition on wafers which are commonly used in the silicon technology. A lot of beautiful experiments have been done in this field.
It is now possible to come back to cavity QED with real Rydberg atoms, because one can keep them at well-defined locations with optical tweezers. It will now become possible to put many atoms at a well-defined position inside a cavity, and have these atoms interact collectively with the cavity field. So, the field of cavity QED still holds many promises. It’s very exciting to see how the field of quantum information is evolving, with a competition between circuit QED and Rydberg atoms in optical lattices.
I think when you first started to perform these pioneering experiments in 1970s and 1980s, you had no idea what the profound implications of your experiment can be. For example, today, when we walked into this corridor (of the Department of Physics in Fudan University), we saw these posters of people doing Rydberg atoms in optical tweezers. In addition, as you just mentioned, the cavity QED evolved into circuit QED, which is now a heavily invested area of superconducting quantum computing. I think these are two examples of the promise in quantum computing systems. Can you comment on these two systems?
I agree that superconducting qubits and Rydberg atom systems are still competing. Which one will be better remains an open question. If you had asked me the question 15 years ago, I would not have believed that circuit QED would become so productive. And if you had asked me 5 years ago, I would have said that circuit QED was far better than Rydberg atom systems. I think now that these Rydberg systems can become competitive again. Quantum science is evolving very fast and this makes the beauty and excitement of research. Scientists in related domains are competing, hopefully in a friendly way.
Like you and David Wineland.
Yes, the friendly competition between Dave and me is a good example. The ideal situation is when good research is accomplished when scientists respect each other and mutually appreciate the beauty of what they are doing. I want to mention in this respect that, one pioneer in the field of Cavity QED was Herbert Walther, who was working in Germany. Unfortunately, he passed away almost twenty years ago.
A highly respected scientist.
He was a pioneer in my field of research. He invented the Rydberg atom micromaser, with an experimental set-up which was very similar to the one we developed for our cavity QED studies. He worked in a different perspective, but it involved the same kind of technology. Walther was a very creative and imaginative physicist. He was also a very powerful and a very influential scientific director at the Max Planck Institute for Quantum Optics in Garching.
I don’t know how the field will evolve in the future. I am very excited by the progress of quantum metrology, using single quantum system to perform measurements more precise than could be achieved with classical devices. We have done some quantum metrology in our group. We used Rydberg atoms to detect small electric and magnetic fields, making use of state superposition to beat the classical sensitivity. But in the field of quantum metrology, the most spectacular progress has been achieved by realizing optical atomic clocks which have now a precision of . This accuracy in the measurement of time is fantastic and will certainly have applications to basic science and to the building of practical devices such as an improved GPS.
The progress of atomic interferometry, including atomic gravimeters and atomic gyroscopes is also worth noting. But the field which everybody talks most about in this area of science is the search for a quantum computer which is still far away in the future, if even it can be one day realized.
Yes, I agree with you that quantum metrology will have more near-term exciting results. For the fields of quantum metrology and quantum computing, as you are the pioneer in both, can you anticipate any breakthroughs in these two fields within the next 5 years and 10 years?
I can safely predict breakthroughs in quantum simulation, the domain which studies quantum structures emulating condensed matter systems at a different scale. Scientists now become very good at trapping atoms or arranging artificial atoms in one-, two-, or three-dimension configurations and have them interact with each other at will. This is a field which is reaching maturity. There are many groups in the world which are competing in this domain, in the United States, in Europe and in China. These experiments are done either with real atoms or with superconducting qubits. It remains so far basic science and I do not know to what kind of practical applications leading to marketable devices it will lead. There are already several startup companies which are selling optical tweezer lattices of neutral atoms which could be used by researchers doing quantum simulation. These systems are not quantum computers because they are not yet able to avoid quantum decoherence.
I agree. I think quantum computers will be like lasers. First, they will be used as a laboratory tool for physicists, chemists, and so on. Then, maybe 10 or 20 years later, they might follow a similar history. In the first 20 years of lasers, I don’t think people have expected any real-world applications like the ones which have been developed in industry. In the meantime, the prospect of having a quantum computer is generating a lot of hopes. Sometimes, it turns into a quantum hype. What do you think about that?
Yes, there is a lot of hype in the research about the quantum computer. For the time being, only toy systems involving a few qubits have been realized, achieving computations which can easily be performed by a classical computer. When the media are talking about a fifty or even a hundred qubit quantum computer, they do not mention the fact that these qubits are not protected against decoherence, that the quantum error corrections required to perform quantum algorithms are far from being efficiently implemented on these systems. Quantum error corrections would require to code a logical qubit in a large ensemble of entangled physical qubits on which measurements diagnosing decoherence processes would be followed by corrections. To make a useful universal quantum computer, you would need many thousands of logical qubits, each of them consisting of thousands of entangled physical qubits. Actually, nobody knows how to achieve it in a scalable and realistic way.
So, I agree that there is a lot of hype, and there are many dangers coming from overselling the quantum computer. If you keep promising year after year things which won’t happen, policy makers and funding agencies will lose patience and there might be a backlash, with a strong decrease in the financing of this research. It is also counterproductive to make politicians believe that a strategic advantage will be given to the first country which will be able to build such a machine. The result is that this research is becoming classified by governmental authorities. When students, postdocs or senior scientists working in this field want to travel from China to Europe, they are often denied visas because politicians believe that the information about this research should not be shared. This is stupid, but I am sorry to say that it comes in part from the scientists themselves who have oversold what they are doing. When you talk to politicians who don’t understand science, if you tell them something which is exaggerated, they take it as if it were true, and then the consequence is this kind of silly restriction to the free exchange of information in a field which is still a topic of basic science.
What I want to say is that the quantum computer is not at the stage of a Manhattan project that you have to protect against competition. It should be an open domain, in which one can only profit from competition, and from sharing information and data. This is something that you can achieve only if you don’t oversell, giving the false impression that a quantum computer will be the solution to all the problems mankind is facing today.
Already in 1998 or so, David Wineland had published papers talking about two-ion quantum computers. He used the word “quantum computer” as a technical term. When describing to the general public this domain of research, with the development of the many startup companies, one has to be more cautious.
Yes, I think the word “quantum computer” should be taken as a kind of metaphor to describe more generally the domain of quantum information science. You have to take it and understand it in that way. If you take it literally and consider that the main goal of this domain of research must be to build this device, it becomes problematic. In fact, I published with Jean-Michel Raimond twenty-six years ago an opinion piece for Physics Today whose title was “Quantum Computing: a Dream or a Nightmare.” We wrote that the quantum computer was a dream for theorists, but a nightmare for experimentalists. It is still the case today. We don’t know how to scale up such a machine and to achieve quantum error correction to the degree which would make such a computer a reality. Some colleagues, including David Wineland, are more optimistic than me on this point, but this is okay. We must be able to disagree on scientific issues in a friendly way.
I have two final questions, and the first one is a little bit like science fiction. If you were 20 years old and just about to start your PhD, and if you had all your memories about what has happened until now, what kind of advice would you give to yourself?
The advice I can give to young students who want to get into research is that they must have a passion for it. You need to recognize one domain, in which you think important discoveries might be made in the future, and tell yourself that you want to be part of this adventure. Once you are attracted into science by a specific goal, many unexpected things can happen which could lead you in new directions, but you should first be motivated by curiosity, find a good reason to start into a career that is very demanding.
If I started myself today with all the knowledge I have acquired in my life, I would be for instance attracted by the search for exoplanets which could sustain forms of life different from the ones we have on Earth. This is a fantastic quest which raises the issue of our place in the universe. It is basic science and at the same time it involves a lot of technological advances. One of the reasons I think about this topic is that it requires some technologies common to the domain of quantum optics that I am familiar with. For example, the analysis of exoplanets needs to develop frequency combs to stabilize lasers, in order to make ultrasensitive Doppler spectroscopy of the light coming from the stars around which these planets are orbiting. It is also useful to implement adaptive optics, which is similar to the technique used to prepare the arrays of optical tweezers my group is using in quantum simulation experiments. For the search of exoplanets, you need also a lot of knowledge in chemistry, biology, and spectroscopy. This is a domain of research which is interdisciplinary. It implies that you will never work alone, you would have to work in teams with people who share your passion and your enthusiasm for research, which is a great asset when you are a scientist. What I also personally like about this research is that it is a priori completely useless. We know that we will never be able to visit these planets, and still our curiosity is compelling us to acquire knowledge about these inaccessible worlds.
I like that. In China, we have an old saying that “the use of the uselessness is of the greatest usefulness,” which means that the most seemingly useless thing may turn out to be the most useful in the far future.
About this point, I should mention a famous essay written in the 1930s by Abraham Flexner, the founder of the Princeton Institute for Advanced Study. Its title is “The Usefulness of Useless Knowledge.” Flexner developed an idea which is obvious for all scientists, the fact that all the practical instruments used in our daily life come from discoveries in basic science, initially motivated by mere curiosity, without any specific application in the mind of the discoverers. The laser, magnetic resonance imaging, atomic clocks, and GPS are good examples. Very often these inventions have come in an unexpected way and would have been great surprises for the scientists who discovered the basic phenomena which led to them. Even if the search for exoplanets is a priori useless because we will never go there, it might indirectly be useful because it will lead us to develop instruments which could be useful to achieve some practical goals.
I have just considered a scientific domain involving observations and experiments. This is the kind of physics I am most familiar with. To young scientists who have a strong mathematical mind, I should of course mention also the holy grail which would be the discovery of the quantum theory of gravitation. It is an open problem whose solution has eluded the brightest theorists up to now. I would advise any young scientist wishing to pursue this ambitious goal to remain also open to other open problems in physics, for instance in condensed matter physics or in quantum information science. These problems might be less challenging, easier to tackle, and could lead to interesting discoveries. They could also give us some hints to reach the holy grail.
It’s a grand unification of physics.
Yes, it’s the grand unification. To achieve this goal theorists should remain close to experimentalists and to observation. The detection of gravitational waves which give us a lot of information about the physics of black holes brings us back to the kind of physics I love, based on observations requiring ever more precise and more sensitive instruments. One can think of the next generation of optical clocks which might become sensitive to the perturbations of the space time curvature induced by the passing of gravitational waves. I know that the pioneers of optical atomic clocks, people like Jun Ye, are interested in this possible application of their devices.
Yeah, these are all fascinating topics. Speaking of searching for intelligence outside us in the universe, I would like to invite you to extend your travel in China. You should visit Guizhou. Scientists have built a huge, world’s largest radio frequency receiver, called FAST (Five-hundred-meter Aperture Spherical radio Telescope) for its abbreviation. It’s doing some quite interesting experiments. And also, there is a very beautiful landscape surrounding, with many high mountains and so on.
Talking about that, there are projects of building gigantic telescopes by coupling optical telescopes with each other, with links using quantum technology. Professor Jianwei Pan is trying to take advantage of quantum features to lock devices together and extract information coherently from different locations. It’s also something very exciting. But let’s not forget that even if we gather a lot of information about regions of the universe far away from us, we are bound to remain on our planet. We must protect the Earth against the very bad things which are happening, including climate change and the threats to the environment and to the biodiversity. Working in these areas should also be the goals of new generations of scientists.
Now, let’s move to the last question. Besides research, you are also very passionate about science education. As you have mentioned, you have written a few scientific books. Can you introduce them to the audience? We have in it a lot of young students and potential readers.
I have written two main books. The first one, Exploring the Quantum written with Jean-Michel Raimond is aimed at graduate students in physics. It describes the physics of atoms interacting with photons in cavities and illustrates fundamental concepts of state superpositions, quantum entanglement, quantum non-destructive measurements, and so on.
Recently, I wrote a more popular book called La lumière révélée (English: The Science of Light) which has just been translated into Chinese (Fig. 1). By describing how mankind has over the centuries acquired its knowledge about light, I express my fascination for the progress of science in general. The scientific method started in the 17th century with the quantitative measurements of space and time, allowing the first evaluation of the velocity of light. Starting my book at that moment, I have tried to follow the lineage of ideas from Galileo to modern science. I am fascinated by the constant progress of scientific knowledge illustrated by the history of light, which has involved a constant exchange between observation, experiments, and theories accounting for them and making new predictions. Telling this story has also given me the opportunity to reflect on the lives of scientists and scholars, not only physicists, but also mathematicians, explorers, and philosophers who have contributed to progressively increasing our knowledge about light.
The English and Chinese editions of La lumière révélée by Serge Haroche.
I also wanted to show in this book that there is a permanent and fruitful interplay between basic and applied science. Basic discoveries about light have led to the invention of new instruments, telescopes, spectrometers, interferometers, lasers, atomic clocks etc. Conversely, these instruments, whose sensitivity has constantly improved, have allowed scientists to perform more precise observations and to discover new phenomena which have lead science in new directions, culminating with the theory of special and general relativity, quantum physics, and fascinating discoveries in cosmology. We are still in this process now. For example, if one day we discover a way to reconcile the general theory of relativity with quantum physics, it will come from more precise experiments or observations revealing new things about nature. This will involve new instruments, either more powerful accelerators or more precise atomic clocks or atomic interferometers. By writing this book, I just wanted to make a general audience aware of the big adventure of modern science. In order to make the book more personal, I have also talked about my own life as a scientist, from the early days of my training in physics, up to the experiments which I have done with my research group. I have tried to convey the idea that science is an adventure to which people coming from different backgrounds all contribute. Scientists all belong to the same community of people sharing the same passion for the truth. That’s the beauty of research which I wanted to express in this book.
One of the Chinese students in our Paris group, Dr. Haiteng Wu, was kind enough to review the Chinese translation and to write a preface for the Chinese readership. When I got the copy in Chinese, I was surprised to see that it is much thinner than the versions of the book in European languages. Chinese characters obviously carry more information per sign than the Latin alphabet!
Okay, thanks so much again, for taking precious time out of your busy schedule to accept our interview. I really enjoyed the discussion with you.
It was a pleasure. I thank you for asking very appropriate and stimulating questions.
Chao-Yang Lu is Chair Professor in Physics at the University of Science and Technology of China (USTC). He completed his BS and PhD degrees at the USTC and the University of Cambridge in 2011. He has been appointed as the Deputy Director of the Shanghai Center for Quantum Sciences and as the Executive Director of the Quantum Computing Division at the Hefei National Laboratory since 2022. His current research interest includes quantum computation, solid-state quantum photonics, multiparticle entanglement, quantum teleportation, superconducting circuits, and atomic arrays. His is the author of over 140 papers in major research journals which have attracted 28000 citations. His work was selected by Physics World as “Breakthrough of the Year” in 2015, by APS Physics as one of the top ten “Highlights of the Year” in 2021 and 2022, and by UNESCO as “World’s top 10 digital innovation technologies” in 2021. He is an OSA/Optica Fellow, and a recipient of the EPS Fresnel Prize, AAAS Newcomb Cleveland Prize, Nishina Asian Award, IUPAP-ICO Young Scientist Prize in Optics, OSA Adolph Lomb Medal, APS Rolf Landauer and Charles H. Bennett Award in Quantum Computing, CLEO James P. Gordon Memorial Speakership, and OCPA Achievement in Asia Award.
Chao-Yang Lu. Light touch in quantum: an interview with Prof. Serge Haroche[J]. Advanced Photonics, 2024, 6(1): 010503.