连续高功率激光辐照对55号钢组织和硬度的影响
下载: 506次
We uses the high-energy heat source of a high-power laser (20 kW) to address the problems of surface oxidation, decarburization behavior, uneven surface hardness, high costs, and environmental pollution in the conventional quenching process of 55 steel surface. By promoting the application of high-power lasers and technological innovation, the study aims to meet practical working conditions for high efficiency, low cost, energy conservation, and environmental protection. The single-variable principle is employed to obtain laser remelting and laser quenching process parameters with different laser power ranges, and the effects of both on the surface morphology, microstructure, and hardness of 55 steel are investigated. The goal is to enhance the working surface properties of 55 steel, thereby increasing its service life and safety, and reducing the likelihood of engineering accidents.
In the study of laser quenching on the surface of 55 steel, we found that neither an excessively high laser power nor an excessively quick scanning speed could improve the surface properties of the steel, particularly under high-power laser quenching with fast scanning. The hardened surface expanded with increased laser power, and despite remelting, the hardness value remained relatively constant. Consequently, a comparative study of laser remelting and laser quenching was proposed. First, to investigate the hardening effect of laser remelting and laser quenching on the working surface of 55 steel, the variation of surface hardness in specimens with increasing laser power at different laser ranges was investigated using the single variable principle, and the surface morphology and boundary thermal diffusion of each specimen were analyzed. Second, the effect of scanning speed on the surface hardness of 55 steel was investigated at medium laser power ranges to further characterize the hardening differences between laser remelting and laser quenching on that material. To characterize the intrinsic hardening difference between these processes, XRD analysis was performed on both low and high-laser-power specimens to study the variation of each physical phase with laser power and to obtain the physical phase difference between remelted and quenched specimens. Finally, the microstructure morphology and hardness changes of the cross-section of the high-laser-power specimens were analyzed, while the EDS line scan of the cross-section of the 2.1 kW specimen was performed to compare the diffusion of elements in the remelted and hardened layers, and based on the above analysis, the intrinsic hardening mechanisms of laser remelting and laser quenching were summarized and analyzed.
By comparing the laser-quenched and laser-remelted specimens within different laser power ranges, we found that the surface hardness has a similar variation pattern. The laser-remelted specimens exhibit a better hardening effect and a significant increase in the cross-sectional hardening layer depth with the increase in the laser power range (Figs. 9 and 10). In addition, the XRD analysis reveals that the laser-remelted specimens had fewer unfused carbide phases than the laser-quenched specimens at different laser power ranges (Fig. 8), and this conclusion is further verified by the microstructure morphology (Fig. 7). Moreover, in the XRD spectra of medium and low laser powers, the peak level of the strongest peak of martensite decreases first and then increases, which indicates that similar lattice distortion occurs with the increase of power in different laser power ranges. In the microstructure comparison, we found that the laser-remelted specimens exhibit more uniform and dense martensite (Fig. 6), and the carbon elements in the remelted layer show stronger diffusion ability than other alloying elements (Fig. 5).
In this study, the effects of different laser power and scanning speeds on the surface hardening effect of 55 steel are investigated. Additionally, the surface hardening differences between remelted and quenched specimens are compared, and the intrinsic hardening mechanism is also analyzed. We found that laser remelting exhibits a superior hardening effect than laser quenching. The surface hardness range of laser-quenched specimens is 446-520 HV, the depth range of the hardened layer is 621-709 μm, with a maximum cross-sectional hardness of 720 HV. For laser-remelted specimens, the surface hardness range is 480-613 HV, the depth range of the hardened layer is 709-813 μm, and the maximum cross-sectional hardness is 755 HV. Carbide particles in the remelted specimens gradually dissolve and diffused with increased power, forming a smoother grain boundary structure. Notably, the remelted layer contains higher carbon atom content and achieves higher hardness, while the quenched specimen still has visible unfused carbide particles in the microstructure of the hardened layer, limiting the acquisition of its high-hardness hardened layer. In addition, the microstructure of both quenched and remelted samples consists of lamellar and slate-like martensite, residual austenite, and some unmelted carbides, but the remelted sample has a more uniform and denser microstructure, a higher content of martensite, and fewer unmelted carbides, and the microstructure is free of pores, cracks, and other defects, which obtains a better hardening effect and provides guidance for the study of surface laser hardening of 55 steel.
1 引言
55号钢是一种常见的优质碳素结构钢,具有良好的综合性能,已被广泛应用于航空航天、电力、石油化工、船舶、机械和电子等行业[1-3]。55号钢零件,如电梯轨道、曲轴、齿轮等,在服役过程中经常会承受各种冲击载荷和冲击磨损,尤其是在复杂环境下服役的零件,其表面可能还要承受侵蚀氧化、热软化及脱碳软化等[4],加速了工作表面的失效,导致零件的使用寿命缩短。硬度和耐磨性能是影响零件表面性能的关键指标,直接关系到运动系统的运行稳定性、安全性和使用寿命[5-6]。因此,提高零件的表面硬度和耐磨性能以实现较长的使用寿命,具有重要的工程意义[7]。热处理是改善零件表面硬度和耐磨性能的最常用方法[8-9]。Wang等[10]利用激光表面淬火技术得到了高于铸铁圆盘基体硬度4倍以上的表面,崔宸等[11]采用传统热处理方式使Stellite-6钴基涂层硬度达到了未处理涂层的1.5倍。
目前,我国很多企业主要采用传统感应淬火技术对55号钢进行表面强化。传统感应淬火技术主要利用电磁感应在零件内部产生趋肤效应,加热零件表层,实现表面淬火的目的。该技术高输入、大范围加热的特点,不仅增大了能耗,还加剧了零件形变和零件表面的氧化程度,严重影响了零件的可靠性。为了获得优质的零件表面,研究人员提出了一种小形变、高效、高质量和节能环保的表面热处理技术——激光重熔与激光淬火技术[12-14]。该技术通过激光与金属材料交互作用产生的热能改变材料内在的晶体结构和元素分布,从而达到改善零件表面性能的目的。该技术在高激光功率下的快速扫描可以提高加工效率,缩短生产周期。
近几年,国内外研究人员为了获得更加卓越的零件表面性能,对电磁感应复合激光淬火[15-16]、水下激光淬火[17]、超声波复合激光淬火[18]以及激光重熔复合传统热处理[19]等复合工艺进行了一系列研究,并取得了相应成果。虽然零件在辅助设备及复合工艺下获得了比单一工艺更加优异的表面性能,但却提高了生产成本,尤其是表面强化效率受到了极大限制。所查资料显示,目前对淬硬性较差的55号钢表面进行高功率、高扫描速度激光硬化的报道甚少。目前,大功率激光器已成为发展趋势,采用高功率激光束对55号钢进行表面强化,不仅可以提高工作效率,还有望提高其表面性能。但是,随着激光功率的增大,55号钢的表面状态会从激光淬火转变为激光重熔,而这二者对55号钢硬化效果的影响目前尚无定论。基于此,笔者采用高斯分布的多模激光束作为热源,对55号钢表面进行强化处理,对比研究激光重熔与激光淬火对55号钢的硬化效果。通过控制工艺参数得到了不同硬度和深度的硬化层,对比了激光重熔与激光淬火硬化效果的差异,进而研究了相应的微观组织演变和硬化机理,并分析了硬化层差异的原因,以期为55号钢表面激光重熔与激光淬火技术的应用提供参考。
2 材料和实验方法
2.1 材料
实验所用基材为55号导轨冷拉型钢,主要用于制造电梯轨道,其化学成分列于
表 1. 55号钢的化学成分(质量分数)
Table 1. Chemical composition of 55 steel
|
2.2 激光硬化实验
对试样进行激光辐照硬化处理,工作原理如
表 2. 激光工艺参数
Table 2. Laser technological parameters
|
2.3 表面裂纹检测和金相实验
激光相变硬化后进行表面裂纹检测和金相分析。利用液体的渗透现象、毛细作用和吸附原理观察硬化层表面是否产生裂纹。探伤时,先用无水乙醇和丙酮清洗待检测表面,接着将红色渗透剂喷涂在待测表面,着色8~10 min后清洗掉渗透剂,然后在检测区域喷涂显像剂,待显像剂干燥后观察白色区域是否显现红色条纹,以此来判断淬火表面是否有裂纹缺陷。对试样进行线切割、研磨、抛光(单喷射抛光机)后,用3%硝酸酒精溶液腐蚀5~11 s,然后利用光学显微镜观察硬化层次表层的微观形貌和组织致密性。需要说明的是,不同区域需要腐蚀不同时间:基体的腐蚀时间为9~11 s,淬硬层的腐蚀时间为7~8 s,重熔层的腐蚀时间为5~6 s。为了观察板条状马氏体和片状马氏体的组织结构,使用JSM-7001F扫描电镜在50 nm微距下对淬硬层进行电子衍射分析。
2.4 硬度检测及物相分析
采用能量色散X射线能谱仪(EDS)分析硬化层中的元素种类、元素含量及分布情况。由于表层会受到杂质元素的影响,因此采用DB Advace X-射线衍射仪(XRD)对试样的次表层进行物相分析,即:沿激光束扫描方向截取块体试样,然后用240目到1500目砂纸逐级打磨,之后用无水乙醇清洗(自干)、无尘纸轻微擦拭后,利用XRD分析淬火次表层的物相。在XRD分析时,加速电压为35 kV,电流为25 mA,阳极选用铜靶,扫描速度为2(°)/min,扫描角度范围为20°~90°。利用HVS-1000数字显微硬度计对硬化层进行显微硬度测试,选择载荷为1.96 N,保压时间为10 s;硬度测试从距离样品表层0.1 mm处开始,沿硬化层相变方向每隔50 μm获取硬化层横截面同一高度处6个点的硬度值,并将其平均值作为最终的硬度值,以展现硬化层更准确的硬度变化趋势。
3 实验结果与分析
3.1 激光辐照前55号钢的内部组织
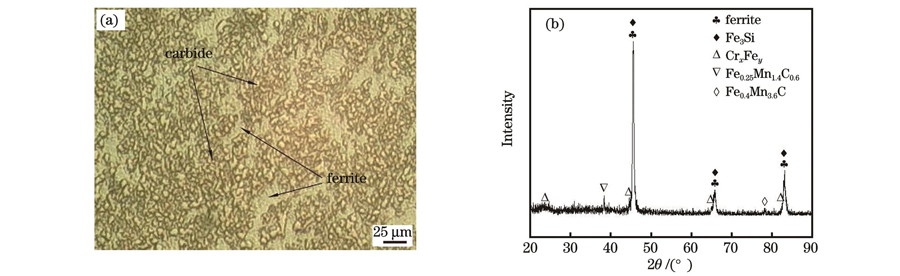
图 2. 基体的金相组织和XRD图谱。(a)金相组织;(b)XRD图谱
Fig. 2. Metallurgical structure and XRD profile of the matrix. (a) Metallurgical structure; (b) XRD profile
3.2 激光辐照对55号钢表面宏观形貌的影响
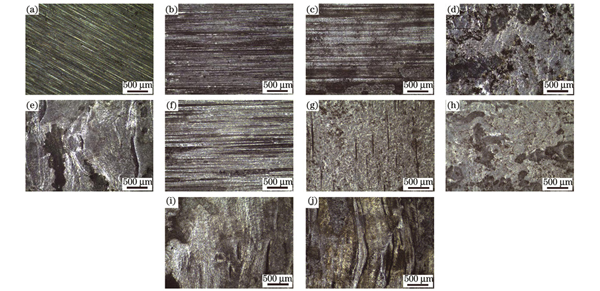
图 3. 不同参数下的表面宏观形貌。(a)基体表面;(b)1.6 kW,5 mm/s;(c)1.7 kW,5 mm/s;(d)1.75 kW,5 mm/s;(e)1.8 kW,5 mm/s;(f)1.9 kW,9 mm/s;(g)2.0 kW,9 mm/s;(h)2.1 kW,9 mm/s;(i)2.2 kW,9 mm/s;(j)2.3 kW,9 mm/s
Fig. 3. Surface macroscopic morphology under different parameters. (a) Matrix surface; (b) 1.6 kW, 5 mm/s; (c) 1.7 kW, 5 mm/s; (d) 1.75 kW, 5 mm/s; (e) 1.8 kW, 5 mm/s; (f) 1.9 kW, 9 mm/s; (g) 2.0 kW, 9 mm/s; (h) 2.1 kW, 9 mm/s; (i) 2.2 kW, 9 mm/s; (j) 2.3 kW, 9 mm/s
从
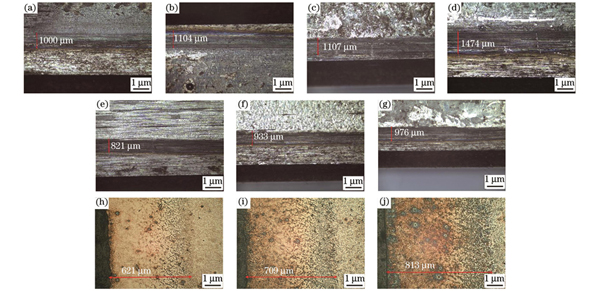
图 4. 不同参数下淬火面宽度的变化以及部分截面的宏观形貌。(a)1.6 kW,5 mm/s;(b)1.7 kW,5 mm/s;(c)1.75 kW,5 mm/s;(d)1.8 kW,5 mm/s;(e)1.9 kW,9 mm/s;(f)2.0 kW,9 mm/s;(g)2.1 kW,9 mm/s;(h)1.9 kW,截面;(i)2.0 kW,截面;(j)2.1 kW,截面
Fig. 4. Change of quenching surface width and macroscopic morphology of partial cross-sections under different parameters. (a) 1.6 kW, 5 mm/s; (b) 1.7 kW, 5 mm/s; (c) 1.75 kW, 5 mm/s; (d) 1.8 kW, 5 mm/s; (e) 1.9 kW, 9 mm/s; (f) 2.0 kW, 9 mm/s; (g) 2.1 kW, 9 mm/s; (h) 1.9 kW, cross-section; (i) 2.0 kW, cross-section; (j) 2.1 kW, cross-section
3.3 激光辐照对55号钢微观组织的影响
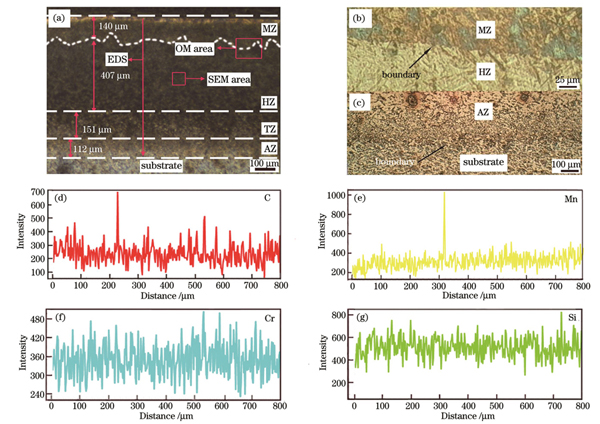
图 5. 2.1 kW重熔试样截面的宏观形貌和EDS线扫描结果。(a)截面宏观形貌;(b)重熔分界线;(c)热影响区分界线;(d)碳元素分布;(e)锰元素分布;(f)铬元素分布;(e)硅元素分布
Fig. 5. Macroscopic appearance and EDS line scanning analysis of 2.1 kW remelted sample cross-section. (a) Macroscopic appearance of the sample cross-section; (b) remelting boundary; (c) dividing line of thermal impact zone; (d) element C distribution; (e) element Mn distribution; (f) element Cr distribution; (g) element Si distribution
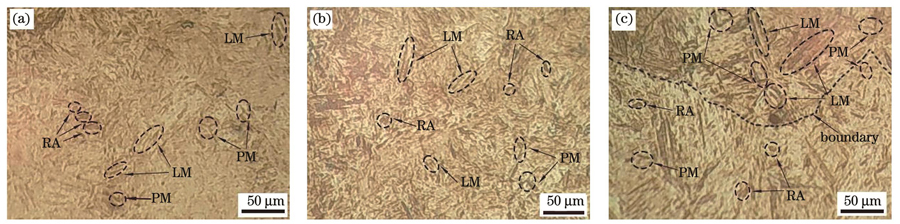
图 6. 中等功率试样次表层的金相组织。(a)1.9 kW,9 mm/s;(b)2.0 kW,9 mm/s;(c)2.1 kW,9 mm/s
Fig. 6. Metallographic structure of the sample subsurface with medium power. (a) 1.9 kW, 9 mm/s; (b) 2.0 kW, 9 mm/s; (c) 2.1 kW, 9 mm/s
此外,由于透射入材料的激光束随穿透距离的增加按指数规律递减,表层至下表层的温度梯度逐渐减小,因此试样中沿激光相变硬化方向的板条状马氏体和片状马氏体的致密度逐渐减弱,如
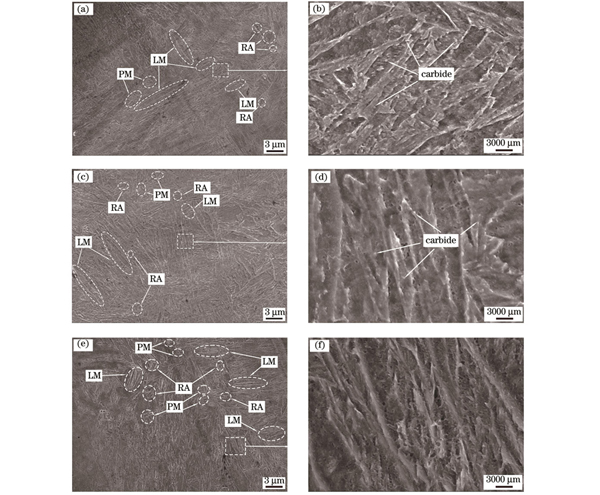
图 7. 中等功率试样淬硬区中部的SEM图。(a)(b)1.9 kW,9 mm/s;(c)(d)2.0 kW,9 mm/s;(e)(f)2.1 kW,9 mm/s
Fig. 7. SEM images of the middle of the intermediate power sample. (a)(b) 1.9 kW, 9 mm/s; (c)(d) 2.0 kW, 9 mm/s; (e)(f) 2.1 kW, 9 mm/s
3.4 55号钢激光辐照后的XRD分析
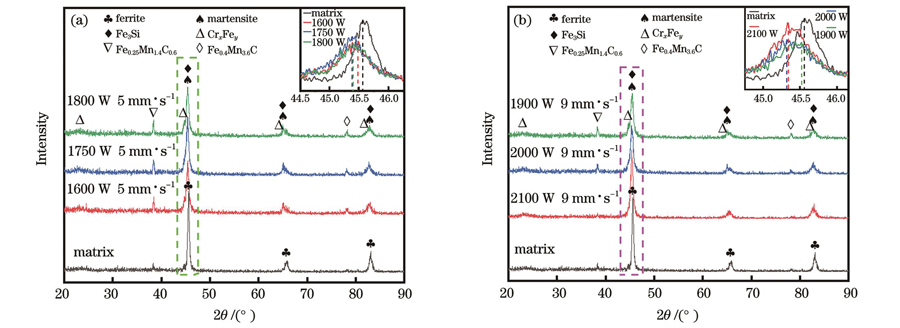
图 8. 不同功率下次表层的XRD图谱。(a)1600~1800 W;(b)1900~2100 W
Fig. 8. XRD profiles of the next surface layer under different laser powers. (a) 1600-1800 W; (b) 1900-2100 W
3.5 激光辐照对55号钢硬度的影响
利用正三边形拼接的均匀性,获得了不同试样表层的硬度均值,具体测量点如
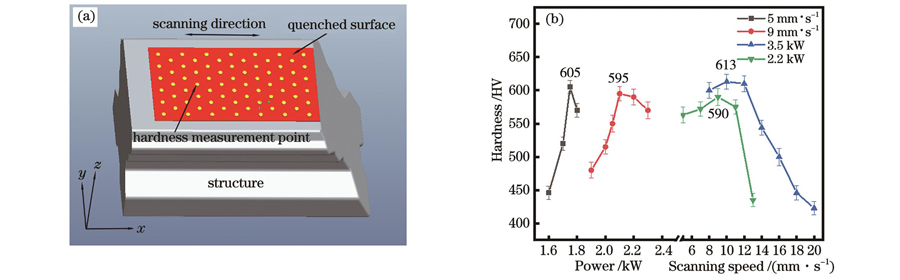
图 9. 试样表层硬度测量点示意图及硬度均值。(a)硬度测量点示意图;(b)表层硬度均值
Fig. 9. Schematic diagram of hardness measurement points and mean hardness. (a) Schematic diagram of hardness measurement points; (b) average surface hardness
中等功率下横截面硬化层的硬度值如
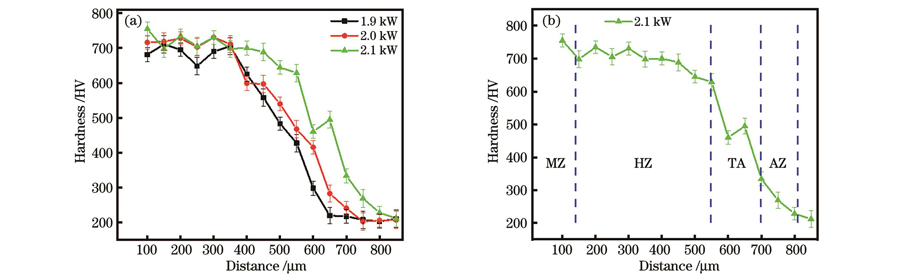
图 10. 中等功率下横截面硬化层的硬度均值。(a)硬度均值;(b)2.1 kW试样截面不同区域的硬度均值
Fig. 10. Mean hardness of the cross-section hardened layer at medium power. (a) Mean hardness; (b) mean hardness of different areas of 2.1 kW sample cross-section
对比
3.6 激光辐照55号钢的硬化机理
激光重熔与激光淬火技术是一个热力学与动力学、传热与传质以及激光与材料交互等综合作用的过程,既与基体的表面粗糙度、热物理特性、组织类型有关,又与激光器类型、激光束模式有关。激光工艺参数决定着激光束与基体的交互时间,控制着温度梯度的形成,进而影响着淬硬层的硬度和深度。具体的硬化机理如
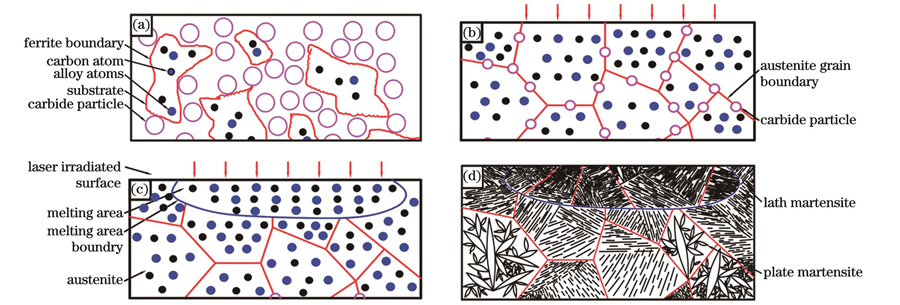
图 11. 硬化机理示意图。(a)激光辐照前的组织示意图;(b)激光淬火时的组织变化;(c)激光重熔时的组织变化;(d)冷却后的组织变化
Fig. 11. Schematic diagrams of the hardening mechanism. (a) Schematic diagram of the microstructure before laser irradiation; (b) microstructure changes during the laser quenching; (c) microstructure changes during the laser remelting; (d) microstructure changes after cooling
4 结论
本文主要研究了55号钢经激光重熔与激光淬火后的组织、表面与截面硬度、硬化层深度及硬化机理,得到的主要结论如下:
1)激光淬火试样的表面硬度范围为446~520 HV,硬化层深度为621~709 μm,横截面最高硬度为720 HV。虽然淬火获得了较好的硬化效果,但硬化层微观组织中依然可见未熔碳化物颗粒,这会限制高硬度硬化层的获得。
2)激光重熔试样的表面硬度范围为480~613 HV,硬化层深度为709~813 μm,横截面最高硬度为755 HV。此外,随着功率增加,重熔试样中的碳化物颗粒得以充分溶解和扩散,形成了更多晶界光滑的组织,尤其是重熔层具有更高的碳原子含量,获得了更高的硬度。
3)淬火试样与重熔试样的微观组织均由片状马氏体、板条状马氏体、残余奥氏体及一些未熔碳化物组成,但重熔试样具有更均匀致密的微观组织、更多的马氏体和更少的未熔碳化物,而且无孔隙、裂纹等缺陷,硬化效果更好。
[1] Li J A, Yan H Z, Li S B, et al. Structural characteristics and sliding friction properties of 40CrNiMo steel after broadband laser hardening[J]. Coatings, 2021, 11(11): 1282.
[2] Babu P D, Marimuthu P. Status of laser transformation hardening of steel and its alloys: a review[J]. Emerging Materials Research, 2019, 8(2): 188-205.
[3] 陈世鑫, 雷卫宁, 任维彬, 等. 激光熔覆与淬火再制造QT700球墨铸铁齿轮熔覆层的组织与性能[J]. 激光与光电子学进展, 2021, 58(5): 0514003.
[4] 林英华, 袁莹, 王梁, 等. 电磁复合场对Ni60合金凝固过程中显微组织和裂纹的影响[J]. 金属学报, 2018, 54(10): 1442-1450.
Lin Y H, Yuan Y, Wang L, et al. Effect of electric-magnetic compound field on the microstructure and crack in solidified Ni60 alloy[J]. Acta Metallurgica Sinica, 2018, 54(10): 1442-1450.
[5] Lu H, Ren Y P, Chen Y, et al. Wear resistance of 20Cr2Ni4A alloy steel treated by laser shock peening and implantation of diamond nanoparticles[J]. Surface and Coatings Technology, 2021, 412: 127070.
[6] Li Q L, Shi S M, Li X, et al. Study on low velocity cyclic impact wear of amorphous carbon films with different mechanical properties[J]. Surface and Coatings Technology, 2020, 402: 126339.
[7] Chen C L, Feng A X, Liu B J, et al. Effect of quench-tempering and laser quenching on the microstructure and properties of high-chromium cast iron[J]. Journal of Materials Research and Technology, 2022, 19: 2759-2773.
[8] Cecchel S, Ferrario D, Cornacchia G, et al. Development of heat treatments for selective laser melting Ti6Al4V alloy: effect on microstructure, mechanical properties, and corrosion resistance[J]. Advanced Engineering Materials, 2020, 22(8): 2000359.
[9] He B, Shao W P, Tian X J. Surface grain formation mechanism in the laser remelting molten pool of a near-β titanium alloy[J]. Materialia, 2022, 24: 101521.
[10] Wang S, Yan S, Lin J, et al. Study on the effects of laser quenching power and scanning speed on the property of cast iron[J]. Journal of Physics: Conference Series, 2022, 2198(1): 012050.
[11] 崔宸, 武美萍, 夏思海. 热处理对42CrMo钢表面激光熔覆钴基涂层性能的影响[J]. 中国激光, 2020, 47(6): 0602011.
[12] Rathmann L, Radel T. Influence of laser hardening on laser induced periodic surface structures on steel substrates[J]. IOP Conference Series: Materials Science and Engineering, 2021, 1135(1): 012024.
[13] Ping X L, Fu H G, Wang K M, et al. Effect of laser quenching on microstructure and properties of the surface of track materials[J]. Surface Review and Letters, 2018, 25(8): 1950030.
[14] 金荣植. 先进的激光淬火技术在机床零件上的应用[J]. 金属加工(热加工), 2016(17): 46-49.
Jin R Z. Application of advanced laser quenching technology in machine tool parts[J]. MW Metal Forming, 2016(17): 46-49.
[15] 张群莉, 林坚, 陈智君, 等. 基于MSC.Marc软件的电磁感应复合激光淬火相变研究[J]. 中国激光, 2021, 48(11): 1103002.
[16] 张群莉, 黄华, 唐泽浩, 等. 42CrMo钢激光-感应复合淬火滚动磨损与疲劳损伤行为研究[J]. 中国激光, 2022, 49(8): 250-261.
Zhang Q L, Huang H, Tang Z H, et al. Rolling wear and fatigue damage behavior of laser-induction hybrid quenching on 42CrMo steel[J]. Chinese Journal of Lasers, 2022, 49(8): 250-261.
[17] Maharjan N, Zhou W, Zhou Y, et al. Underwater laser hardening of bearing steels[J]. Journal of Manufacturing Processes, 2019, 47: 52-61.
[18] Hu X F, Qu S G, Chen Z T, et al. Rolling contact fatigue behaviors of 25CrNi2MoV steel combined treated by discrete laser surface hardening and ultrasonic surface rolling[J]. Optics & Laser Technology, 2022, 155: 108370.
[19] Chen R, Wang H M, Li J, et al. Effect of laser remelting and heat treatment on microstructure and wear resistance of 2A97 Al-Li alloy[J]. Surfaces and Interfaces, 2022, 33: 102197.
[20] 甄延波, 程良, 常森, 等. 激光淬火工艺及应用研究[J]. 金属加工(热加工), 2018(12): 63-66.
Zhen Y B, Cheng L, Chang S, et al. Study on laser quenching technology and its application[J]. MW Metal Forming, 2018(12): 63-66.
[21] Xu J L, Zou P, Liu L, et al. Investigation on the mechanism of a new laser surface structuring by laser remelting[J]. Surface and Coatings Technology, 2022, 443: 128615.
[22] 张群莉, 童文华, 陈智君, 等. 光斑尺寸对42CrMo钢激光深层淬火硬化层几何特征的影响[J]. 表面技术, 2020, 49(1): 254-261.
Zhang Q L, Tong W H, Chen Z J, et al. Effect of spot size on geometrical characteristics of laser deep quenching hardened layer of 42CrMo steel[J]. Surface Technology, 2020, 49(1): 254-261.
[23] Xie J C, Raoelison R N, Zhang Y B, et al. Mesoscopic segregation in H13 steel molten pool during laser remelting: a combined influence of Marangoni convection and oxidation[J]. Journal of Materials Processing Technology, 2023, 316: 117956.
[24] Xie J C, Raoelison R N, Di R F, et al. Laser remelting of AISI H13 tool steel: influence of cooling rate on the surface properties[J]. Surface Topography: Metrology and Properties, 2022, 10(4): 044003.
[25] Liverani E, Ascari A, Fortunato A. Multilayered WC-Co coatings by direct energy deposition-based cladding: effect of laser remelting on interface defects[J]. Surface and Coatings Technology, 2023, 464: 129556.
[26] Stormvinter A, Hedström P, Borgenstam A. A transmission electron microscopy study of plate martensite formation in high-carbon low alloy steels[J]. Journal of Materials Science & Technology, 2013, 29(4): 373-379.
[27] Sugiyama M, Takei M, Sekida S, et al. Characterization of hierarchical lath martensite microstructure in low carbon steels using ultra-high voltage TEM and SEM-EBSD analysis[J]. IOP Conference Series: Materials Science and Engineering, 2022, 1249(1): 012020.
[28] 杨志翔, 王爱华, 熊大辉, 等. 钢轨表面宽带激光淬火工艺及其疲劳磨损性能[J]. 中国机械工程, 2019, 30(3): 254-260.
Yang Z X, Wang A H, Xiong D H, et al. Laser wide-band hardening of steel rails and corresponding fatigue wear property[J]. China Mechanical Engineering, 2019, 30(3): 254-260.
[29] 杨振, 樊湘芳, 邱长军, 等. 40CrNiMoA钢表面激光淬火后的组织和性能[J]. 激光与光电子学进展, 2020, 57(1): 011405.
[30] Li Z Y, Zhang J, Dai B Q, et al. Microstructure and corrosion resistance property of laser transformation hardening pre-hardened AISI P20 plastic die steel[J]. Optics & Laser Technology, 2020, 122: 105852.
[31] 刘其斌, 周芳, 徐鹏. 激光材料加工及其应用[M]. 北京: 冶金工业出版社, 2018.
LiuQ B, ZhouF, XuP. Laser material processing and its application[M]. Beijing: Metallurgical Industry Press, 2018.
[32] 米炫霖. 2738模具钢的工业机器热激光淬火工艺研究[D]. 天津: 天津职业技术师范大学, 2021: 56-65.
MiX L. Thermal laser quenching process of industrial machine in 2738 mold steel[D]. Tianjin: Tianjin Vocational Normal University, 2021: 56-65.
Article Outline
杨仁人, 林英华, 彭龙生, 黄伟, 王新林. 连续高功率激光辐照对55号钢组织和硬度的影响[J]. 中国激光, 2023, 50(16): 1602207. Renren Yang, Yinghua Lin, Longsheng Peng, Wei Huang, Xinlin Wang. Effect of Continuous High-Power Laser Irradiation on Microstructure and Hardness of 55 Steel[J]. Chinese Journal of Lasers, 2023, 50(16): 1602207.