相干涡旋和刃型位错在海洋湍流中的相互作用【增强内容出版】
It is well known that there are two typical phase singularities in the fully coherent beams, i.e., the optical vortex and the edge dislocation. Although much of research has explored properties of the fully coherent beams, there are practical uses of the partially coherent beams because they are more resistant to degradation with propagation through turbulent medium than the former. The propagation of the partially coherent beams carrying coherence singularities in oceanic turbulence has attracted much attention due to its application in underwater wireless communication. It is interesting to ask how oceanic turbulence can affect the interaction of coherence vortex and edge dislocation carried by partially coherent beams. Because the Gaussian Schell-model beam is a typical example of partially coherent beams, the interaction of the coherence vortex and edge dislocation carried by the Gaussian Schell-model beams in oceanic turbulence is studied in detail.
By making an analogy with definition of the edge dislocation in coherent beams, the coherence edge dislocation is shown to be in existence in partially coherent beams. Based on the extended Huygens-Fresnel principle, the analytical expression of the cross-spectral density for the Gaussian Schell-model beams carrying the coherence vortex and edge dislocation propagating through oceanic turbulence is derived, which is used to study the interaction of them in oceanic turbulence. The position of correlation singularities of the partially coherent beams at the z plane can be determined by the curves of the real component and imaginary component, as well as phase distribution of the spectral degree of coherence of the Gaussian Schell-model beams.
There should exist another type of coherence singularities, namely the coherence edge dislocation with π-phase jump located along a line in the transverse plane of the correlation function, which is different from the edge dislocation in fully coherent beams (Fig.1), because the transverse edge dislocation with π-phase shift is located along a line in the transverse plane. The coherence edge dislocation is split into two optical vortices by the coherence vortex if the edge dislocation is off-axis, while it is broken into one optical vortex as it is on-axis. The result is similar to the interaction of the phase vortex and edge dislocation in free space. The coherence edge dislocation is translated into one coherence vortex or two vortices with propagation of the beams in oceanic turbulence (Fig.3). The total topological charge is not conserved with propagation of the initial beams with the coherence vortex and off-axis edge dislocation in oceanic turbulence, because appearance or disappearance of a coherent vortex may take place with propagation. The result is different from the interaction of a phase vortex and an off-axis edge dislocation in free space, because the total topological charge is conserved in the latter case. The evolution of the coherence singularities speeds up with increasing the value of the rate of dissipation of mean-square temperature χT and the relative strength of salinity and temperature fluctuationω, as well as decreasing the rate of dissipation of turbulent kinetic energy per unit mass ε (Fig.4). The physical reason can be explained by the theoretical expression of the strength of oceanic turbulence. It is seen that the strength of the oceanic turbulence becomes stronger with increasing the rate of dissipation of mean-square temperature and the relative strength of salinity and temperature fluctuation, as well as decreasing the rate of dissipation of turbulent kinetic energy per unit mass. When the initial beam parameters, such as the spatial correlation length δ0, the off-axis distance and the slope of the edge dislocation of the coherence edge dislocation change, the changes of positions and number of coherence singularities in the fields take place with propagation of the beams. It is found that not only creation and annihilation of a pair of coherent vortices, but also appearance and disappearance of a vortex take place with varying the initial beams parameters (Figs.5?7).
In the present study, we have firstly introduced the definition of the coherence edge dislocation in accordance with previous researches. Then, the analytical expression of the cross-spectral density for the Gaussian Schell-model beams carrying the coherence vortex and edge dislocation propagating through oceanic turbulence is derived, which is then used to study the interaction of them in oceanic turbulence. It has been shown that the interaction depends on propagation distance, oceanic turbulence parameters, and the beam parameters such as the spatial correlation length and the slope and off-axis distance of the coherent edge dislocation. The creation and annihilation of pairs of coherence vortices occur and the appearance and disappearance of a coherent vortex may also take place by changing these influencing factors. The total topological charge is not generally conserved with propagation of the initial beams. Furthermore, the stronger the oceanic turbulence is, the faster the decrease of the distance for the conservation of the topological charges is.
1 引言
奇点光学以前主要关注相干光束中强度为0而相位不确定的点或者线,光场中的这些点或者线称为相位奇点,其中的这类点称为光涡旋,这类线称为刃型位错[1-2]。何德(He)等[3]研究了高斯余弦光束携带的光涡旋和刃型位错在自由空间的相互作用,发现光束在传输过程中拓扑电荷一般不守恒。尽管过去的多数研究关注相干光束的传输特性,但是部分相干光因在通过湍流介质时不易退化而具有实际应用价值[4-6]。在部分相干光场中强度一般不等于0,但是光束中一对空间点的光谱相干度(SDC)可能存在零点,表明光束的相关函数可能拥有光学奇点(也叫相关奇点)。这类相干奇点称为相干涡旋,它被定义为垂直于光束传输方向的横平面内一对SDC为0而相位不确定的空间点[7]。根据相关研究文献[3,7-8],类似于相位奇点中的刃型位错奇点,我们认为部分相干光中存在着另一类相干奇点——相干刃型位错,将其定义为在垂直于传输方向的横平面内一对SDC为0的空间点的线,并且越过该线时相位发生π突变。
近年来,携带相干涡旋的光束通过海洋湍流的传输的研究由于在光通信方面的应用引起了广泛关注[9-15]。研究者们发现:涡旋光束通过海洋湍流传输过程中,相干涡旋的数量和位置会发生变化[8,13]。现在的问题是,部分相干光束携带的相干光涡旋和刃型位错在海洋湍流中的相互作用是怎样的?高斯-谢尔模(GSM)光束是典型的部分相干光束[16]。本文以GSM光束为例,采用扩展的惠更斯-菲涅耳原理,推导出携带相干涡旋和刃型位错的GSM光束在海洋湍流中的交叉谱密度传输表达式,数值模拟和分析了海洋湍流中相干涡旋和刃型位错的相互作用特点。
2 理论模型
2.1 携带相干刃型位错的GSM光束
完全相干光束波前可能含有相位奇点,其中典型的有光涡旋和刃型位错。在这些奇点处,光束的相位不确定而光强为0[1]。考虑携带刃型位错的高斯光束在初始平面z=0处的光场分布[17]
式中:
携带相位奇点的完全相干光束在z平面上相位奇点的位置可以由光场的相位图来确定,也可以由下列方程确定[2]:
部分相干光束因其空间和时间的随机起伏性,必须采用交叉谱密度W(
式中:I(
部分相干光束交叉谱密度相位的可分性决定了光谱相干度的相位奇点性质。在I(
与携带相干涡旋的部分相干光束模型类似,通过引入谢尔相关因子[19],携带相干刃型位错的GSM光束在初始平面处的交叉谱密度表示为
式中:δ0表示空间相关长度。
满足相干度定义式条件I(
![携带刃型位错的高斯光束的Re[E]=0和Im[E]=0曲线和携带相干刃型位错的GSM光束的SDC的Re[μ]=0和Im[μ]=0曲线。(a)高斯光束的Re[E]=0和Im[E]=0曲线;(b)GSM光束的SDC的Re[μ]=0和Im[μ]=0曲线](/richHtml/zgjg/2024/51/8/0805002/img_01.jpg)
图 1. 携带刃型位错的高斯光束的Re[E]=0和Im[E]=0曲线和携带相干刃型位错的GSM光束的SDC的Re[μ]=0和Im[μ]=0曲线。(a)高斯光束的Re[E]=0和Im[E]=0曲线;(b)GSM光束的SDC的Re[μ]=0和Im[μ]=0曲线
Fig. 1. Curves of Re[E]=0 and Im[E]=0 of Gaussian beam with edge dislocation and Re[μ]=0 and Im[μ]=0 of SDC for GSM beam with coherence edge dislocation. (a) Curve of Re[E]=0 and Im[E]=0 of Gaussian beam; (b) curve of Re[μ]=0 and Im[μ]=0 of SDC for GSM beam
2.2 携带相干涡旋和刃型位错的GSM光束
寄居于高斯光束的光涡旋和刃型位错在初始平面的光场分布为[3]
由
类似于携带相干刃型位错的GSM光束表达
由式(4)~
![携带相干涡旋和刃型位错的GSM光束的SDC的Re[μ]=0和Im[μ]=0曲线及相位分布。(a)Re[μ]=0和Im[μ]=0曲线;(b)相位分布](/richHtml/zgjg/2024/51/8/0805002/img_02.jpg)
图 2. 携带相干涡旋和刃型位错的GSM光束的SDC的Re[μ]=0和Im[μ]=0曲线及相位分布。(a)Re[μ]=0和Im[μ]=0曲线;(b)相位分布
Fig. 2. Curves of Re[μ]=0 and Im[μ]=0 and phase distribution of SDC for GSM beam with coherence vortex and edge dislocation. (a) Curves of Re[μ]=0 and Im[μ]=0; (b) phase distribution
2.3 携带相干涡旋和刃型位错的GSM光束在海洋湍流中传输
基于扩展的惠更斯-菲涅耳原理,部分相干光通过海洋湍流的交叉谱密度表达式为
式中:k为波数,k=2π/λ;ψ为复数相位的随机部分;*表示复共轭;
式中:ω为盐度和温度波动的相对强度;ε为单位质量湍流动能的耗散率;η为柯尔莫哥洛夫湍流微尺度;χT为均方温度耗散速率。
由
采用积分公式[24]
把GSM光束在初始平面处的交叉谱密度表达
式中:
由
3 海洋湍流中相干涡旋和刃型位错相互作用
利用式(4)~
3.1 相干涡旋对相干刃型位错的分裂
为了查明相干刃型位错的离轴距离对其被相干涡旋分裂的影响,

图 3. 携带相干涡旋和刃型位错的GSM光束传输到z=5 m处的SDC相位分布。(a)b=0.1w0;(b)b=0
Fig. 3. Contour lines of phase of SDC for GSM beam with coherence vortex and edge dislocation propagating to z=5 m. (a) b=0.1w0; (b) b=0
3.2 湍流参数的影响
为了查明湍流参数对相干涡旋和刃型位错作用的影响,

图 4. 湍流参数χT,ε和ω取不同值时GSM光束在海洋湍流传输中相干涡旋的位置。(a)χT=10-10 K2/s,ε=10-4 m2/s3,ω=-5;(b)χT=10-9 K2/s,ε=10-4 m2/s3,ω=-5;(c)χT=10-10 K2/s,ε=10-5 m2/s3,ω=-5;(d)χT=10-10 K2/s,ε=10-4 m2/s3,ω=-1
Fig. 4. Position of coherent vortices of GSM beam propagating through oceanic turbulence for different values of χT, ε and ω. (a) χT=10-10 K2/s, ε=10-4 m2/s3, and ω=-5; (b) χT=10-9 K2/s, ε=10-4 m2/s3, and ω=-5; (c) χT=10-10 K2/s, ε=10-5 m2/s3, and ω=-5; (d) χT=10-10 K2/s, ε=10-4 m2/s3, and ω=-1
由χT=10-9 K2/s时的
由ε=10-5 m2/s3时的
与海水均方温度耗散速率和单位质量湍流动能耗散率的影响不同,海水盐度和温度波动的相对强度ω增加时,GSM光束携带的相干涡旋和刃型位错演化速度加快。例如,ω=-1时的
3.3 光束初始参数的影响
为了查明初始相干刃型位错离轴距离对相干涡旋和刃型位错作用的影响,
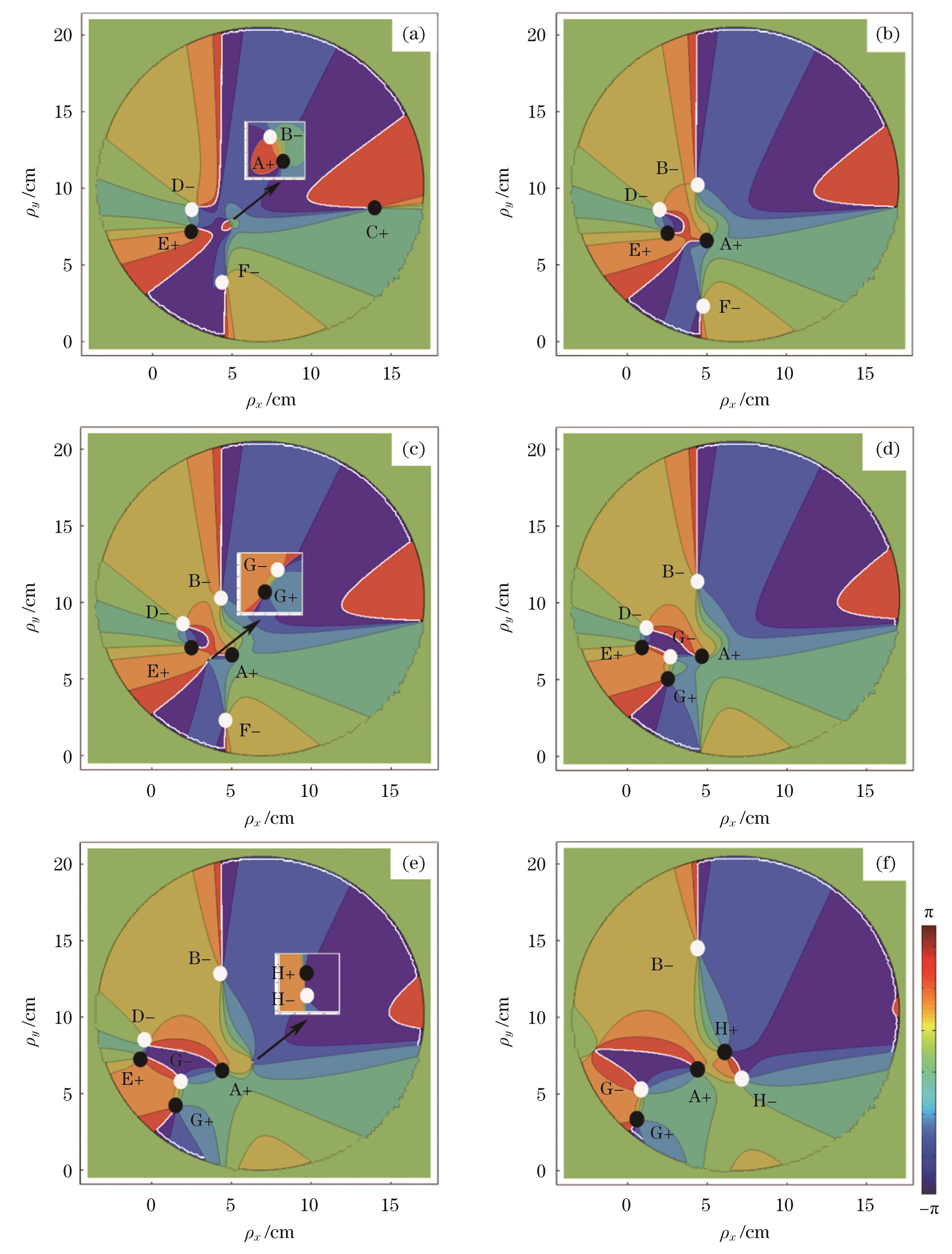
图 5. 海洋湍流中携带相干涡旋和不同离轴距离的刃型位错的GSM光束的SDC相位分布。(a)b=0;(b)b=0.31w0;(c)b=0.33w0;(d)b=0.51w0;(e)b=0.745w0;(f)b=0.99w0
Fig. 5. Contour lines of phase of SDC for GSM beam with coherence vortex and edge dislocation in oceanic turbulence for different values of off-axis distance. (a) b=0; (b) b=0.31w0; (c) b=0.33w0; (d) b=0.51w0; (e) b=0.745w0; (f) b=0.99w0
为了查明初始相干刃型位错斜率对相干涡旋和刃型位错作用的影响,

图 6. 海洋湍流中携带相干涡旋和不同斜率刃型位错的GSM光束的SDC相位分布。(a)a = 0;(b)a = 0.0024;(c)a = 0.007;(d)a = 0.013;(e)a = 0.015;(f)a = 0.0242
Fig. 6. Contour lines of phase of SDC for GSM beam with coherence vortex and edge dislocation in oceanic turbulence for different values of slope of edge dislocation. (a) a = 0; (b) a = 0.0024; (c) a = 0.007; (d) a = 0.013; (e) a = 0.015; (f) a = 0.0242
为了查明初始光束空间相关长度对相干涡旋和刃型位错作用的影响,

图 7. 海洋湍流中携带相干涡旋和刃型位错的GSM光束在不同相关长度情形下的SDC相位分布。(a)δ0=0.01 cm;(b)δ0=0.021 cm;(c)δ0=0.051 cm;(d)δ0=0.088 cm;(e)δ0=0.106 cm;(f)δ0=0.147 cm
Fig. 7. Contour lines of phase of SDC for GSM beam with coherence vortex and edge dislocation in oceanic turbulence for different values of spatial correlation length. (a) δ0=0.01 cm; (b) δ0=0.021 cm; (c) δ0=0.051 cm; (d) δ0=0.088 cm; (e) δ0=0.106 cm; (f) δ0=0.147 cm
4 结论
本研究通过类比完全相干光相位奇点的概念,提出了在部分相干光束中存在一种相干奇点——相干刃型位错。基于扩展的惠更斯-菲涅耳原理,推导出了携带相干涡旋和刃型位错的光束在海洋湍流环境下的交叉谱密度表达式,并用来研究该环境下相干涡旋和刃型位错的相互作用规律。结果表明:相干刃型位错在相干涡旋的作用下转化成相干涡旋。在光束传输过程中,光场中不仅可能有单个或成对相干涡旋的产生或消失,也有成对相干涡旋的产生和湮灭现象发生。相干涡旋和刃型位错的相互作用不仅与传输距离有关,还受光束初始参数和海洋湍流参数的影响。均方温度耗散速率χT和盐度温度波动的相对强度ω越大,或者单位质量湍流动能的耗散率ε越少,海洋湍流就越强,光束中相干涡旋的拓扑电荷守恒的距离就越小。本研究所得结果对于理解部分相干光及其携带的相干奇点在海洋湍流中的演化规律,寻求其在海洋光通信方面的潜在应用,有一定参考价值。
[1] SoskinM S, VasnetsovM V. Singular optics[M]∥Progress in optics, Vol. 42. Amsterdam: Elsevier, 2001: 219-276.
[2] DennisM R, O’HolleranK, PadgettM J. Singular optics: optical vortices and polarization singularities[M]∥Progress in optics, Vol. 53. Amsterdam: Elsevier, 2009: 293-363.
[3] He D, Yan H W, Lü B D. Interaction of the vortex and edge dislocation embedded in a cosh-Gaussian beam[J]. Optics Communications, 2009, 282(20): 4035-4044.
[4] Gu Y L, Gbur G. Reduction of turbulence-induced scintillation by nonuniformly polarized beam arrays[J]. Optics Letters, 2012, 37(9): 1553-1555.
[5] 张凯宁, 刘永欣, 蒲继雄. 涡旋光束在海洋湍流中传输的闪烁因子[J]. 中国激光, 2019, 46(7): 0705001.
[6] 陈康, 厉淑贞, 潘玉琪, 等. 部分相干径向偏振旋转对称幂指数相位涡旋光束紧聚焦特性研究[J]. 光学学报, 2022, 42(22): 2226002.
[7] Schouten H F, Gbur G, Visser T D, et al. Phase singularities of the coherence functions in Young’s interference pattern[J]. Optics Letters, 2003, 28(12): 968-970.
[8] Palacios D M, Maleev I D, Marathay A S, et al. Spatial correlation singularity of a vortex field[J]. Physical Review Letters, 2004, 92(14): 143905.
[9] Huang Y P, Zhang B, Gao Z H, et al. Evolution behavior of Gaussian Schell-model vortex beams propagating through oceanic turbulence[J]. Optics Express, 2014, 22(15): 17723-17734.
[10] 刘永欣, 陈子阳, 蒲继雄. 随机电磁高阶Bessel-Gaussian光束在海洋湍流中的传输特性[J]. 物理学报, 2017, 66(12): 124205.
Liu Y X, Chen Z Y, Pu J X. Propagation of stochastic electromagnetic high-order Bessel-Gaussian beams in the oceanic turbulence[J]. Acta Physica Sinica, 2017, 66(12): 124205.
[11] Liu Z L, Chen J L, Zhao D M. Experimental study of propagation properties of vortex beams in oceanic turbulence[J]. Applied Optics, 2017, 56(12): 3577-3582.
[12] Xu Y, Shi H F, Zhang Y X. Effects of anisotropic oceanic turbulence on the power of the bandwidth-limited OAM mode of partially coherent modified Bessel correlated vortex beams[J]. Journal of the Optical Society of America A, 2018, 35(11): 1839-1845.
[13] Ye F, Zhang J B, Xie J T, et al. Propagation properties of the rotating elliptical chirped Gaussian vortex beam in the oceanic turbulence[J]. Optics Communications, 2018, 426: 456-462.
[14] Liu D J, Wang G Q, Yin H M, et al. Propagation properties of a partially coherent anomalous hollow vortex beam in underwater oceanic turbulence[J]. Optics Communications, 2019, 437: 346-354.
[15] Wang W, Wang P, Pang W N, et al. Evolution properties and spatial-mode UWOC performances of the perfect vortex beam subject to oceanic turbulence[J]. IEEE Transactions on Communications, 2021, 69(11): 7647-7658.
[16] 郭寅龙, 聂芯雨, 李晋红, 等. 扭曲椭圆高斯谢尔模光束的自修复特性[J]. 激光与光电子学进展, 2023, 60(17): 1703001.
[17] MandelL, WolfE. Optical coherence and quantum optics[M]. Cambridge: Cambridge University Press, 1995.
[18] Tang B H, Luo Y M, Zhang Y, et al. Analytical vectorial structure of Gaussian beams carrying mixed screw-edge dislocations in the far field[J]. Optics Communications, 2014, 324: 182-187.
[19] 田燕男, 段美玲, 吴云光, 等. 高斯谢尔模型光束在生物组织中的光谱变化[J]. 中国激光, 2019, 46(12): 1207001.
[20] Gbur G. Partially coherent vortex beams[J]. Proceedings of SPIE, 2018, 10549: 1054903.
[21] Freund I, Shvartsman N. Wave-field phase singularities: the sign principle[J]. Physical Review A, 1994, 50(6): 5164-5172.
[22] Gbur G, Wolf E. Spreading of partially coherent beams in random media[J]. Journal of the Optical Society of America A, 2002, 19(8): 1592-1598.
[23] Korotkova O, Farwell N, Shchepakina E. Light scintillation in oceanic turbulence[J]. Waves in Random and Complex Media, 2012, 22(2): 260-266.
[24] GradysteynI, RyzhikI. Table of integrals[M]∥Series and products. 7th ed. New York: Academic Press, 2007.
[25] 陈斐楠, 陈延如, 赵琦, 等. 部分相干厄米高斯光束在海洋湍流中光束传输质量的变化[J]. 中国激光, 2013, 40(4): 0413002.
[26] 任庭瑞, 于笑楠, 佟首峰, 等. 基于数字信号处理的高灵敏度水下光通信发收机设计与评估[J]. 中国激光, 2022, 49(4): 0406005.
Article Outline
陈海涛, 高曾辉. 相干涡旋和刃型位错在海洋湍流中的相互作用[J]. 中国激光, 2024, 51(8): 0805002. Haitao Chen, Zenghui Gao. Interaction Between Coherence Vortex and Edge Dislocation in Oceanic Turbulence[J]. Chinese Journal of Lasers, 2024, 51(8): 0805002.