基于光反射式耦合探针的高灵敏曲率与振动双参量传感器
Due to inherent merits of anti-electromagnetic disturbance, compact size, high sensitivity and low fabrication cost, fiber curvature sensor (FCS) and fiber vibration sensor (FVS) play important roles in optical fiber sensing and optical fiber communication, which can achieve effective structural safety monitoring and be widely used in structural health monitoring basic fields such as machinery manufacturing, bridge transportation, oil and gas pipelines. Moreover, the researches on multi-parameter fiber optic sensors have been driven by miniaturized and multifunctional sensor solutions, as well as the need to meet the measurement of multiple physical quantities in narrow operating environments. While meeting the application requirements of curvature and vibration sensing, it is necessary to further improve sensitivity and response range. In practical sensing applications, solving the cross-sensitivity problem of multiple parameters and applying it in confined spaces and harsh environments also puts forward higher requirements for the compactness, flexibility, and adaptability of sensors. In this paper, a highly sensitive curvature and vibration dual-parameter sensor based on optical reflective coupler probe (ORCP) is proposed and demonstrated. With the advantages of high sensitivity, wide response range, good linearity, high stability, high fidelity, and the probe size is in mm level with compact structure, the dual-parameter sensor based on ORCP would further be widely used in limited space and harsh environment fields, providing good application prospects in oil, coal mine and other structural safety monitoring fields.
The beam propagation method (BPM) was used to simulate the modal field intensity distribution of different ORCPs. In order to fabricate the ORCP, it is necessary to obtain the single mode microfiber coupler (SMC) firstly. Two single mode fibers (SMFs, core/cladding diameter is 8.2/125 μm, NA is 0.14) are aligned with each other before they are fused together using the flame modification method. During the fabrication process, the hydrogen gas flow, stretching speed and length, which determine the performance of the SMC are controlled and optimized. Based on the brittle fracture characteristics of quartz optical fibers, applying axial tension to the fabricated SMC and snapping the waist region with a gem knife to form the Fresnel reflection end face with high quality. The waist diameter and coupling region length of the ORCP are characterized by optical microscope. For curvature sensing, the bending signals applied to the coupling region of the ORCP cause changes in the wavelength and intensity of reflection spectra. A broadband source (BBS, 1250 nm to 1650 nm) is connected to the port 1 and the reflection spectra of the ORCP are recorded by an optical spectrum analyzer (OSA, AQ6370D, resolution of 0.02 nm, 900 nm-1700 nm) real time through port 2 of the ORCP. For vibration sensing, a piezoelectric transducer is connected to the coupling region of the ORCP to apply vibration signals. If the wavelength of narrow linewidth laser was tuned to the reflection spectral wave-nodes of the ORCP, the output intensity will be modulated. A tunable laser source (TLS, line width < 5 kHz) is connected to the port 1 and output signals (port 2) are recorded by potodetector and oscilloscope to realize the detection of continuous single-frequency, damped vibration signal and sound recognition.
In the measurement of curvature sensing based on the absolute symmetric ORCP, as curvature is increased from 0 m-1 to 9.58 m-1, the wavelength red shifts and is stable gradually while the intensity changes weakly (Fig. 6). When the curvature increases from 0 m-1 to 1.92 m-1, the wavelength red shifts with sensitivities of 0.63 nm/m-1 (-0.29 dB/m-1) @ 1510 nm and 0.58 nm/m-1 (0.29 dB/m-1) @ 1470 nm, respectively. The linearity (R2) is ~0.99. As curvature is increased from 1.92 m-1 to 3.75 m-1, the sensitivities are 2.75 nm/m-1 (2.16 dB/m-1) @ 1470 nm and 2.84 nm/m-1 (-2.01 dB/m-1) @ 1510 nm, respectively. The curvature continues to increase from 3.75 m-1 to 9.58 m-1, the wavelength and intensity are stable. In the measurement of curvature sensing based on the single ORCP, the envelope of the ORCP's reflection spectrum red shifts with increased curvatures (Fig. 7). As curvature is increased from 0.57 m-1 to 10.49 m-1, the shift of the spectral envelope is ~56.6 nm. The measured curvature sensitivity is 11.97 nm/m-1 (-1.88 dB/m-1) @ 1470 nm ranging from 0.57 m-1 to 3.72 m-1 and the R2 is 0.98. When curvature increases from 4 m-1 to 10.49 m-1, the sensitivity is 2.63 nm/m-1@1470 nm with R2 of 0.94. The experimental results indicate that the proposed ORCP is suitable for monitoring small curvature deformations. For the vibration sensing, the proposed single ORCP can achieve frequency response range from 185 Hz to 20 MHz without data filtering process. The R2 of vibration detection is ~1 and the resolution of real-time vibration signal monitoring can reach 1 Hz with good fidelity (Fig. 9). The ORCP achieves a sensitivity of 0.72 mV/V@80 kHz (Fig. 11) and the highest signal-to-noise ratio is ~53.56 dB @ 2 MHz (Fig. 10). The vibration amplitude of the ORCP at different frequencies is tested for many times and the amplitude fluctuation is <0.1 dB. In addition, the sensor can realize the detection of damped vibration signal and sound recognition with high stability.
A highly sensitive curvature and vibration dual-parameter sensor based on ORCP is proposed and demonstrated. The ORCP is fabricated by melting coupling method and vertical cutting technology. The sensing performance is stable and not influenced by packaging methods, achieving high sensitivity for detecting weak curvature and vibration signals. Applying bending or vibration deformation signals to the ORCP cantilever-beam coupling region changes the refractive index and mode field distribution of the interference supermodes, causing a shift in the wavelength or reflection of the spectrum, realizing sensing of curvature and vibration with high sensitivity. For curvature sensing, the sensitivity is up to 11.97 nm/m-1 ranging from 0 m-1 to 10.49 m-1, and the linearity is >0.98. For vibration sensing, the ORCP has a sensitivity of 0.72 mV/V@80 kHz and achieves an ultra-wideband frequency response range from 185 Hz to 20 MHz with high fidelity and linearity, and the signal-to-noise ratio is ~53.56 dB. In addition, the sensor can realize the detection of damped vibration signal and sound recognition with high stability. The proposed curvature and vibration sensor based on ORCP has the advantages of high sensitivity, wide response range, good linearity, high stability, high fidelity, and the probe size is in mm level with compact structure, supporting its potential application prospects in limited space and harsh environment fields such as oil field, coal mine and other structural safety monitoring fields, which is expected to achieve the prediction of potential threats of infrastructure emergencies.
1 引 言
光纤传感器具有抗电磁干扰、体积小、灵敏度高、稳定性好、制造成本低等显著优点,其中,光纤曲率传感器(FCS)和光纤振动传感器(FVS),可以实现有效的结构安全监测,广泛应用于机械制造、桥梁运输、油气管道等结构健康监测基础领域,用于预测基础设施突发事件或事故的潜在威胁并有效避免。
基于不同光纤结构的FCS和FVS相继被提出,包括光纤光栅(OFG)[1-3]、马赫-曾德尔干涉仪(MZI)[4-6]、法布里-珀罗干涉仪(FPI)[7-8]和微光纤结构[9-10]等。通过光纤的结构与材料功能化作用[11-14],可实现高灵敏度曲率和振动检测。2015年,Sun等[11]基于错位偏芯熔接方法制备single mode fiber-fiber Bragg grating-multimode fiber-single mode fiber(SMF-FBG-MMF-SMF)结构,曲率灵敏度达到-0.164 nm/m-1。2016年,Dass等[12]利用火焰喷刷技术在SMF上产生两个级联薄锥的混合级联结构,曲率灵敏度达到-11.92 dB/m-1,范围为0 m-1~1 m-1。2018年,Ni等[13]提出一种基于石墨烯膜片的声学FVS,频率响应范围(FR)为5 Hz~0.8 MHz,信噪比(SNR)高达35 dB。2019年,Zhou等[14]报道出基于Morpho蝴蝶翅膀光子纳米结构的声FVS,SNR高达56.4 dB@1 kHz,响应范围为200 Hz~4500 Hz。2022年,本研究团队提出一种基于氧化石墨烯-FPI以及模式选择耦合器的高灵敏振动传感器,响应范围分别为4 Hz~20 kHz[15]以及3 Hz~22 MHz[16]。
为进一步增强FCS以及FVS的灵敏度并拓宽响应范围,基于新型光纤结构的光纤传感器被相继提出。2021年,Jiang等[17]基于保偏光纤长周期光栅提出一种FCS,最大灵敏度达到9.26 nm/m-1,曲率响应范围为0 m-1~4.137 m-1。2019年,Tan等[18]提出基于一多模双锥形超细光纤的FCS,曲率灵敏度最大为0.7 nm/m-1,范围为0 m-1~11.82 m-1。同年,Novais等[8]提出一种基于多模气泡腔结构的FCS,曲率灵敏度达到(0.561±0.014)dB/m-1。2019年,Yu等[19]基于空心光子晶体光纤提出全光纤温度不敏感型FVS,响应范围为1 Hz~20 kHz。2019年,Sui等[20]提出一种基于双包层光纤的声FVS,响应范围为1 Hz~400 kHz。在满足曲率和振动传感应用要求的同时,需要进一步提高灵敏度和响应范围。在实际传感应用中需要解决多个参数的交叉敏感性问题[21]以及应用在受限空间与恶劣环境时对传感器的紧凑型、灵活性和适应性也提出了更高的要求。
本文提出并研究一种基于光反射式耦合探针(ORCP)的高灵敏度曲率传感器(ORCP-FCS)和振动传感器(ORCP-FVS)。利用两根SMF通过加热熔融、拉锥、耦合方法结合垂直切割技术,在耦合区域构建菲涅耳反射端面而成。基于紧凑的悬臂梁探针式结构,实现对曲率和振动两种物理量的高灵敏度测试。施加在ORCP上的弯曲、振动形变可改变结构悬臂耦合区域的折射率分布,引起反射光谱波长或强度的偏移。对于曲率传感测试,灵敏度高达11.97 nm/m-1,线性度大于0.98。对于振动传感测试,ORCP实现了从185 Hz到20 MHz的超宽带响应范围,灵敏度为0.72 mV/V@80 kHz,具有高保真度以及高线性度,SNR高达~53.56 dB。此外,该传感器可实现阻尼振动信号的检测以及声音识别。由于该传感器具有灵敏度高、稳定性好、频率响应范围宽以及结构紧凑性高等特点,在结构健康监测和环境评估中具有潜在的应用价值。
2 理论模型与器件制备
2.1 ORCP的理论模型

图 1. 不同ORCP内的模场传输与功率分布。(a)ORCP结构示意图;(b)(e)未弯曲和弯曲ORCP的模场传输;(c)(f)未弯曲和弯曲ORCP纤芯和包层中的功率传播;(d)(g)未弯曲和弯曲ORCP耦合区内包层中功率传播细节放大图
Fig. 1. The mode field transmission and power distribution within different ORCPs. (a) Schematic diagram of ORCP; (b)(e) mode field transmission in ORCP without bent and with bent; (c)(f) power propagation in the core and cladding of ORCP without bent and with bent; (d)(g) enlarged view of power propagation details in the cladding within the coupling zone of the ORCP without bent and with bent
由于整个耦合区域中偶超模和奇超模之间的干涉,ORCP的输出端功率产生耦合与交换。根据耦合模理论,该ORCP为弱耦合器件,在x和y偏振态下的耦合系数[22]为
式中:n1和n0分别为光纤包层以及空气中的折射率系数;a为耦合区单根微纳光纤的直径(a=D/2);U∞=2.405;V=[(2πa)/λ](n12-n02)1/2;λ为入射光波长。当光由port 1进入并由端面反射,由port 2输出,该端口的输出功率P2为
式中:L是ORCP的耦合长度;
当相位差满足
在ORCP上施加扰动信号,改变耦合区域干涉模场分布,导致ERID以及干涉长度L改变,引起相位差变化,改变输出光谱,体现在光谱波长漂移量的变化上,干涉光谱波长的漂移量为
式中,Δc为外界扰动物理量的变化。根据
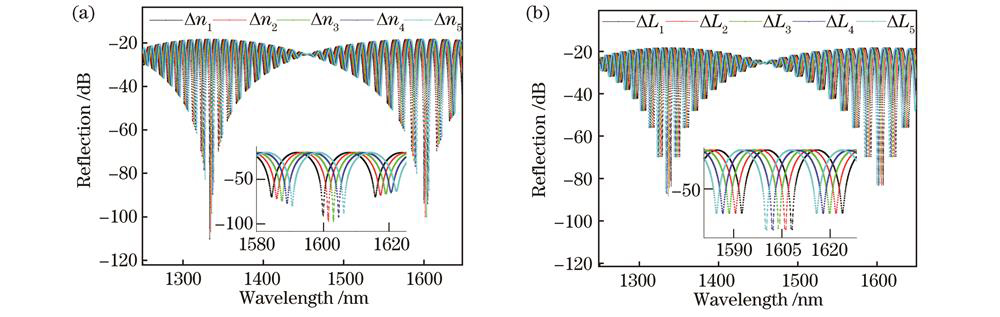
图 2. 不同结构参数下ORCP的仿真反射光谱。(a)L固定,不同ERID;(b)ERID固定,不同L
Fig. 2. Simulated reflection spectra of ORCP with different structural parameters. (a) Different ERIDs with a fixed L; (b) different L with a fixed ERID
2.2 器件制备与性能
将两个平行SMF(纤芯/包层直径为8/125 µm,纤芯/包层RI为1.455/1.445)使用火焰改性方法熔融拉锥、耦合,形成普通SMF耦合器(绝对对称型ORCP)。制备过程中,控制并优化决定光纤结构性能的氢气流量、拉伸速度和长度。基于石英光纤的易脆性断裂特性,对结构施加轴向张力的同时,垂直切割单模光纤耦合器耦合区域的中心位置,以产生高质量菲涅耳反射端面。ORCP的port 1与port 2分别与宽带光源(BBS,1250 nm~1650 nm)以及光谱分析仪(OSA,AQ6370D,带宽分辨率为0.02 nm)相连,实时记录输出反射光谱。

图 3. ORCP的显微镜实物图和反射光谱。(a)耦合区纵向显微镜图像;(b)耦合区横向光纤截面显微镜图像;(c)反射光谱
Fig. 3. Microscopic images and the measured spectral results of the ORCP. (a) At the coupling region; (b) at the fiber cross-section; (c) the output spectrum
3 曲率传感实验
3.1 实验装置
式中:X为毛细玻璃管与ORCP接触点至ORCP固定点处的距离,约1.87 cm;d为毛细玻璃管垂直上下的移动距离。

图 4. 基于ORCP的曲率传感实验。(a)实验装置;(b)原理分析
Fig. 4. Curvature sensing experiment of the ORCP. (a) Experimental setup; (b) principle analysis
3.2 实验结果与分析
在基于ORCP的曲率传感实验中,首先测试了基于绝对对称型ORCP的曲率特性,并与单个ORCP的曲率传感特性进行对比。绝对对称型ORCP是垂直切割前完整的光纤耦合器,属于透射型光纤器件,具体结构如

图 5. 基于绝对对称型ORCP的曲率传感实验。(a)绝对对称型ORCP结构示意图;(b)实验装置
Fig. 5. Curvature sensing experiment of the absolute symmetric ORCP. (a) Schematic diagram of the absolute symmetric ORCP; (b) experimental setup
基于绝对对称型ORCP的曲率测试结果如

图 6. 基于绝对对称型ORCP的曲率传感实验结果。(a)不同曲率下的透射光谱;(b)波长、强度与曲率拟合关系曲线
Fig. 6. Experimental results of curvature sensing based on absolute symmetric ORCP. (a) The transmission spectra with different curvatures; (b) fitting relationship between wavelength, transmission and curvature
在单个ORCP的曲率实验中,毛细玻璃管单调升高以实现ORCP的弯曲,测试单个ORCP的曲率传感特性。
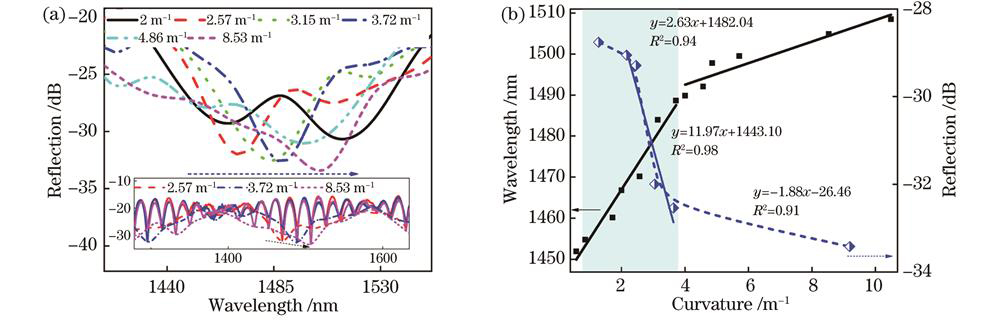
图 7. 基于ORCP的弯曲传感实验结果。(a)不同曲率下的ORCP反射光谱及其包络;(b)波长、反射强度与曲率之间的拟合关系
Fig. 7. Experimental results of curvature sensing based on ORCP structure. (a) The reflection spectra and the envelopes with different curvatures; (b) fitting relationship between wavelength, reflection and curvature
将所提ORCP-FCS性能与之前报道过的基于不同类型光纤结构的FCS进行比较,包括曲率灵敏度以及曲率响应范围,结果如
表 1. 不同类型光纤曲率传感器的性能比较
Table 1. Performance comparisons of different FCSs
|
4 振动传感实验
4.1 实验装置
4.2 连续单频振动传感
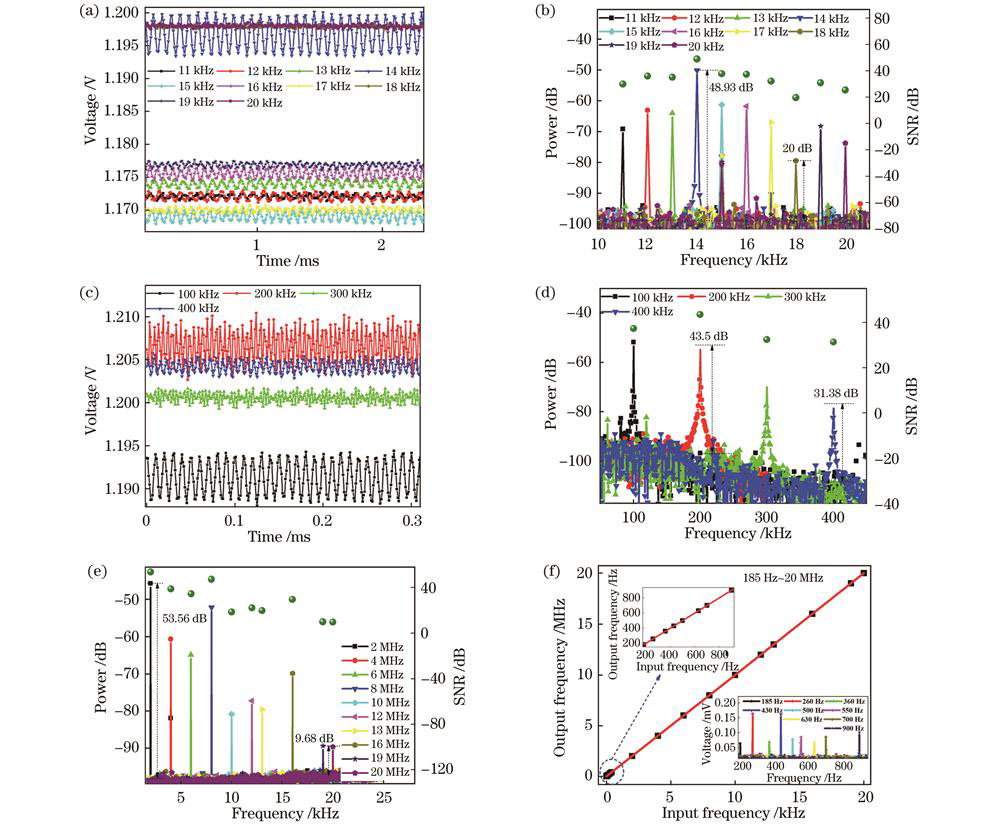
图 9. 基于ORCP-FVS对不同连续单频振动信号的响应测试。(a)(c)11 kHz~400 kHz振动信号对应的时域谱;(b)(d)(e)11 kHz~20 MHz振动信号的频域谱和对应的SNR;(f)185 Hz~20 MHz的基本输出振动信号频率与实际施加的振动信号频率关系,插图为185 Hz~900 Hz振动信号的频域谱
Fig. 9. The measured response of the ORCP-FVS with different single-frequency vibration signals. (a)(c) The measured frequency-domain spectra from 11 kHz to 400 kHz; (b) (d) (e) the corresponding time-domain spectra of vibration signals and SNRs from 11 kHz to 20 MHz; (f) the linearity of the ORCP-FVS for vibration detection of the n-randomly applied signal frequencies from 185 Hz to 20 MHz, the inset is the frequency-domain spectra from 185 Hz to 900 Hz

图 10. SNR与施加信号频率的关系曲线
Fig. 10. The measured relationship between frequency of applied vibration signal and SNR

图 11. 不同驱动电压下测试的输出振动信号。(a)时域谱;(b)输出振动信号幅值与驱动电压的关系
Fig. 11. Measured vibration signals with different amplitudes. (a) The time-domain spectra ; (b) measured vibration amplitudes versus driving voltage amplitudes
4.3 阻尼振动传感与声音识别
通过敲击信号,基于ORCP实现阻尼振动测试。

图 12. 基于ORCP-FVS的敲击阻尼振动测试。(a)时域谱;(b)对应的频域谱
Fig. 12. Measured damped vibration response of the ORCP-FVS. (a) Time-domain spectra; (b) frequency-domain spectra
实验中利用扬声器发出不同音乐,包括“chiled”“carton”“bounce”,加载至ORCP的悬空耦合区,记录其输出光谱的变化,实现对不同声信号的识别。
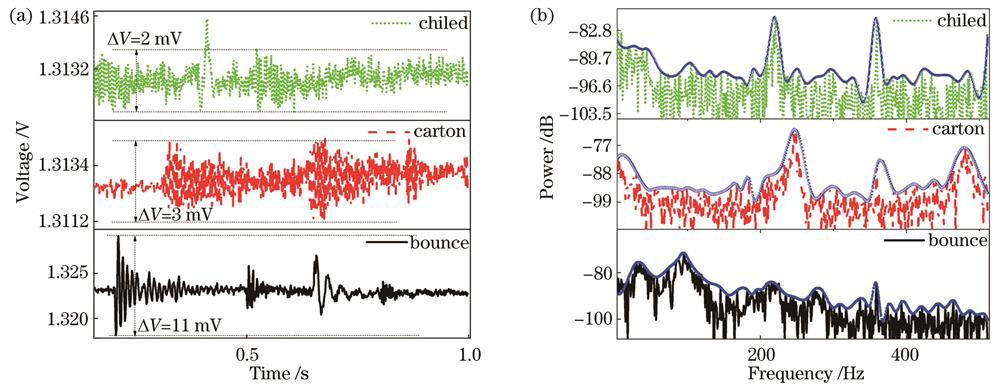
图 13. 基于ORCP-FVS的语音识别测试结果。(a)加载不同声音对应的输出时域谱;(b)对应的频域谱
Fig. 13. The measured speech recognition results of ORCP-FCS. (a) The measured time-domain spectra with different sounds; (b) the corresponding frequency-domain spectra
为对比明显,将所提ORCP-FVS性能与之前报道过的基于不同类型光纤结构的FVS进行比较,包括SNR、FR以及振动方式,结果如
表 2. 不同类型光纤振动传感器的性能比较
Table 2. Performance comparisons of different FVSs
|
所提传感器对曲率和振动交叉不敏感。与弯曲信号不同,振动信号是一个实时、连续的变化量,当振动信号加载于ORCP结构耦合区域,不会引起反射光谱的漂移。通过BBS以及OSA,基于ORCP反射光谱波长的漂移以及光谱强度的变化,实现曲率传感测试(
5 稳定性测试
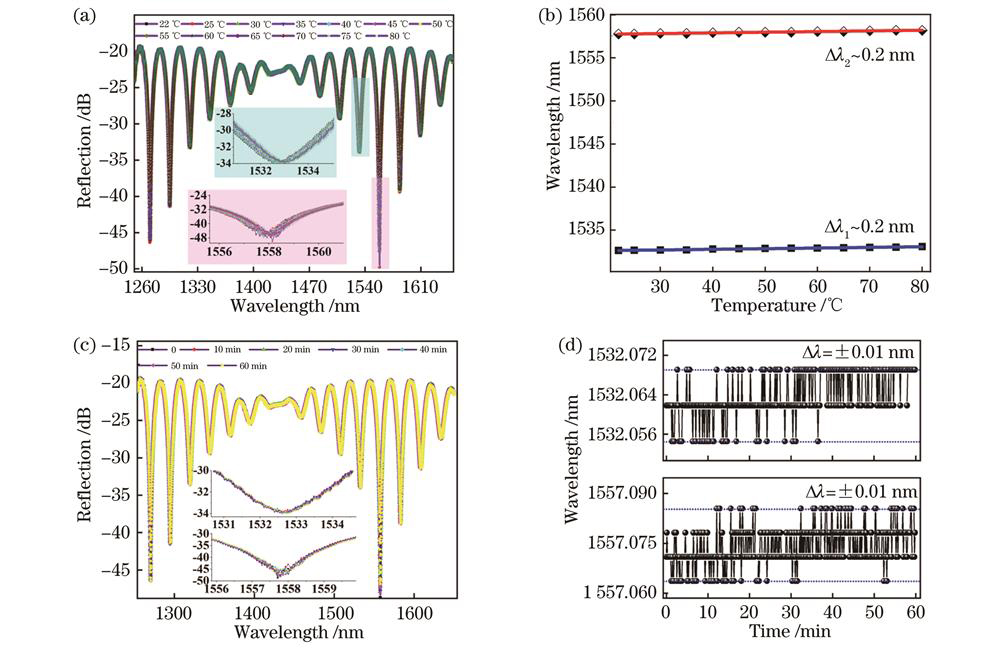
图 14. ORCP的稳定性测试。(a)不同温度下的反射光谱;(b)温度与波长对应关系;(c)1 h内的反射光谱;(d)1 h内光谱波长漂移的变化
Fig. 14. The measured stability of the ORCP. (a) The measured reflection spectra with different temperature; (b) the corresponding relationship between temperature and wavelength; (c) the measured reflection spectra within 1 h; (d) the wavelength fluctuation within 1 h
该传感器的探针结构尺寸为mm级别,结构紧凑,采用反射光谱解调传感物理量,光源和光谱测试仪器处于同一端,可实现曲率与振动双参量传感,可应用于包括结构机械运行、民用基础设施的健康监测以及在油田、煤矿等受限空间与恶劣环境领域中,甚至实现自然灾害的预测。与此同时,所提ORCP传感器具有独特的“探针式”结构,可以穿透某些痕量溶液并能够在微小空间内操作,在燃料储存、化学液位测量、生物分子结合领域具有广泛的应用前景。针对实际传感探头对洁净度和环境扰动的要求,还需要改善。
6 结 论
首次提出并研究一种基于ORCP的高灵敏曲率和振动双参量传感器。通过熔融拉锥、耦合方法结合垂直切割技术,在耦合区域构建菲涅耳反射端面,制成ORCP,实现对曲率和振动两种物理量的高灵敏度测试。对于曲率传感测试,灵敏度高达11.97 nm/m-1,曲率测量范围为0~10.49 m-1,线性度大于0.98。对于振动传感测试,ORCP实现了185 Hz~20 MHz的超宽带频率响应范围,具有高保真度以及高线性度,SNR高达~53.56 dB。此外,该传感器可实现阻尼振动信号的检测以及声音识别,具有较高的稳定性和较低的温度交叉敏感。由于该传感器具有灵敏度高、响应范围宽、线性度好、稳定性高、保真度高等优势,探针尺寸为mm级别,结构紧凑,在结构健康监测和环境评估中具有潜在的应用价值。
[1] Liu K J, Luo B B, Wu D C, et al. Broadband vibration sensor using reflected excessively tilted fiber grating with clamped beam[J]. IEEE Photonics Technology Letters, 2021, 33(8): 379-382.
[2] 姜宗福, 习锋杰, 许晓军, 等. 光栅型波前曲率传感器原理和应用[J]. 中国激光, 2010, 37(1): 205-210.
[3] 张乐, 吴波, 叶雯, 等. 基于光纤光栅法布里-珀罗腔锁频原理的高灵敏度光纤振动传感器[J]. 光学学报, 2011, 31(4): 0406006.
[4] Cheng H H, Wu S, Wang Q, et al. In-line hybrid fiber sensor for curvature and temperature measurement[J]. IEEE Photonics Journal, 2019, 11(6): 6803311.
[5] Dong S D, Dong B, Yu C Y, et al. High sensitivity optical fiber curvature sensor based on cascaded fiber interferometer[J]. Journal of Lightwave Technology, 2018, 36(4): 1125-1130.
[6] 付广伟, 李颀峰, 李昀璞, 等. 温度不敏感的错位熔接-粗锥型光子晶体光纤曲率传感器[J]. 光学学报, 2016, 36(11): 1106007.
[7] Zhu F X, Zhang Y D, Qu Y C, et al. Self-assembled vector curvature sensor based on vernier effect[J]. IEEE Sensors Journal, 2022, 22(7): 6586-6592.
[8] Novais S, Silva S O, Frazão O. A self-referencing intensity-based Fabry–Perot cavity for curvature measurement[J]. IEEE Sensors Letters, 2019, 3(10): 3501704.
[9] Guo Y, Zhang Y D. A new bamboo-shaped sensor for curvature measurement with microstructured fiber[J]. IEEE Photonics Technology Letters, 2021, 33(12): 619-622.
[10] Wei Y, Liu C B, Liu C L, et al. Multichannel directional recognition SPR curvature sensor based on D-type double-clad multimode fiber[J]. IEEE Sensors Journal, 2022, 22(22): 21719-21726.
[11] Sun H, Yang S, Zhang X L, et al. Simultaneous measurement of temperature and strain or temperature and curvature based on an optical fiber Mach-Zehnder interferometer[J]. Optics Communications, 2015, 340: 39-43.
[12] Dass S, Jha R. Micrometer wire assisted inline Mach-Zehnder interferometric curvature sensor[J]. IEEE Photonics Technology Letters, 2016, 28(1): 31-34.
[13] Ni W J, Lu P, Fu X, et al. Ultrathin graphene diaphragm-based extrinsic Fabry-Perot interferometer for ultra-wideband fiber optic acoustic sensing[J]. Optics Express, 2018, 26(16): 20758-20767.
[14] Zhou L Y, He J Q, Li W Z, et al. Butterfly wing hears sound: acoustic detection using biophotonic nanostructure[J]. Nano Letters, 2019, 19(4): 2627-2633.
[15] Chen Y F, Wan H D, Lu Y, et al. An air-pressure and acoustic fiber sensor based on graphene-oxide Fabry-Perot interferometer[J]. Optical Fiber Technology, 2022, 68: 102754.
[16] Chen Y F, Wan H D, Lu Y, et al. Highly sensitive twist and vibration sensing based on polarization-sensitive mode selective coupler[J]. Optical Engineering, 2022, 61(8): 086109.
[17] Jiang C, Liu Y Q, Mou C B. Polarization-maintaining fiber long-period grating based vector curvature sensor[J]. IEEE Photonics Technology Letters, 2021, 33(7): 358-361.
[18] Tan S J, Li Y P, Liu T, et al. A large measurement range bending sensor based on microfiber probe[J]. IEEE Photonics Technology Letters, 2019, 31(24): 1964-1967.
[19] Yu H H, Luo Z Z, Zheng Y, et al. Temperature-insensitive vibration sensor with Kagomé hollow-core fiber based Fabry–Perot interferometer[J]. Journal of Lightwave Technology, 2019, 37(10): 2261-2269.
[21] Qin S P, Lu J Y, Yu Y, et al. Magnetic field and temperature two-parameter sensor based on optical microfiber coupler interference (OMCI) wrapped with magnetic fluid and PDMS[J]. Optics Express, 2021, 29(18): 29492-29504.
[22] Ding M, Wang P F, Brambilla G. A microfiber coupler tip thermometer[J]. Optics Express, 2012, 20(5): 5402-5408.
[23] Li K W, Zhang N, Zhang N M Y, et al. Birefringence induced Vernier effect in optical fiber modal interferometers for enhanced sensing[J]. Sensors and Actuators B: Chemical, 2018, 275: 16-24.
[24] Li H Y, Wang Z, Liu Y G, et al. FFT algorithm-assisted polarimetric twist sensor[J]. IEEE Photonics Technology Letters, 2017, 29(23): 2083-2086.
[25] Xu Y P, Lu P, Qin Z G, et al. Vibration sensing using a tapered bend-insensitive fiber based Mach-Zehnder interferometer[J]. Optics Express, 2013, 21(3): 3031-3042.
[26] Ran Y L, Xia L, Han Y, et al. Vibration fiber sensors based on SM-NC-SM fiber structure[J]. IEEE Photonics Journal, 2015, 7(2): 6800607.
Article Outline
陈彧芳, 万洪丹, 顾宇, 常洪强, 沈展羽, 汪静丽, 胡芳仁. 基于光反射式耦合探针的高灵敏曲率与振动双参量传感器[J]. 光学学报, 2023, 43(21): 2128001. Yufang Chen, Hongdan Wan, Yu Gu, Hongqiang Chang, Zhanyu Shen, Jingli Wang, Fangren Hu. Highly Sensitive Curvature and Vibration Dual-parameter Sensor Based on Optical Reflective Coupler Probe[J]. Acta Optica Sinica, 2023, 43(21): 2128001.