Super-Resolution Fluorescence Microscopy for Cilia Investigation and Ciliopathy Diagnosis (Invited)特邀综述
1 Introduction
Fluorescence microscopy has been extensively used in biological research due to its molecular specificity, live-cell compatibility, and multicolor observation[1-2]. However, due to diffraction of light, the resolution of fluorescence microscopy is limited to ~250 nm, impeding researchers from observing the mechanism below the diffraction limit. Super-resolution microscopy(SRM)has emerged as a collection of techniques that break the diffraction limit and push the optical resolution to several nanometers, enabling the visualization of subdiffraction cellular structures.
Based on different principles, as shown in

图 1.
Fig. 1. Summary of different super-resolution imaging techniques. (a) Conventional imaging; (b) SIM; (c) SOFI; (d) STED; (e) expansion microscopy; (f) SMLM
Two other techniques—super-resolution optical fluctuation imaging(SOFI)and expansion microscopy—also achieve super-resolution imaging. 4)SOFI uses the intrinsic fluctuation properties of fluorophores and is a computational technique that relies on the post-data analysis of the fluorescence signal correlation to achieve enhanced resolution[15]. The simplest second-order SOFI offers a
Recent developments in super-resolution microscopy involve the combination of different techniques to achieve sub-10-nm resolution or molecular resolution. MINFLUX[19] is such an example that uses a donut-shaped excitation laser rather than a depletion laser to scan a fluorescent molecule to determine its center position, which requires significantly fewer photons yet provides nanometer accuracy. Similar to SMLM, by introducing photoswitchable fluorescent molecules, MINFLUX can sequentially localize molecules and realize super-resolution imaging with several nanometer resolutions.
The range of tools available for super-resolution imaging is expanding, with each offering distinct characteristics to meet various needs in biological research, such as higher spatial and/or temporal resolution, multiplexed labeling, reduced phototoxicity, and extended sample depth. In summary, these techniques have dramatically altered fluorescence microscopy and pushed it to the nanoscopy era, revolutionizing the field of biology.
2 Cilia and ciliopathy
Cilia are hair-like slender cellular organelles that arise from membrane-docked centrioles and protrude from the surface of the cell. Cilia can be motile or sensory depending on whether they beat or not.
Generally, sensory cilia are solitary and critical for signal perception and transduction. One example is the photoreceptor outer segment, which is a specialized cilium for photon reception. Sensory cilia appear in nearly all human cells and function in signaling pathways, such as Hedgehog(Hh), GPCR, RTK, and WNT signaling[20]. Recent studies reported that sensory cilia have diverse functions, such as controlling appetite[21], regulatig circadian clock[22], and forming axon-cilium synapses[23].
Motile cilia also possess sensory functions but are designed specifically for fluid propelling or cell motility. Motile cilia are present along the airway epithelia, brain ventricular wall, and female fallopian tube to remove mucus, circulate cerebrospinal fluids, and deliver zygotes[24-26]. Mucociliary clearance is the primary innate immunity mechanism in the airways[27]:airway cilia beat continuously in a coordinated metachronous pattern to eliminate mucus-trapped pathgogens[28]. In the central nervous system, the synchronized beating of ependymal cilia plays an indispensable role in the directional flow of cerebrospinal fluid[29]. Sperm cells move along the female reproductive system and are locomoted by a specialized cilium(flagellum), and motile cilia cover the epithelia of the fallopian tube[26].
Cilia defects lead to ciliopathies, a collection of rare diseases that affect nearly all human organs, including symptoms such as blindness, obesity, kidney abnormalities, and/or mental retardation[30]. While certain ciliopathies mainly affect one organ, others affect nearly all organs, examples being patients with Bardet-Biedl syndrome who suffer from vision loss, obesity, polydactyly, intellectual problems, and infertility. Despite their rarity, because of the variety of ciliopathies, the cumulative prevalence reaches ~1 in 2000 populations[31].
Dysfunctions of motile cilia lead to motile ciliopathies[26], as shown in
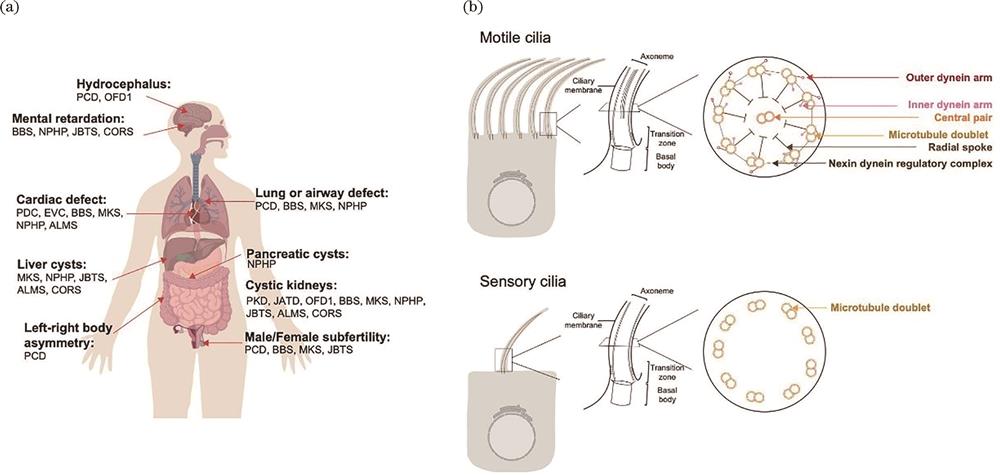
图 2.
Fig. 2. Cilia and ciliopathy. (a) Cilia and affected organs; (b) structure of sensory and motile cilia
3 Size and architecture of cilia
For long, cilia have been regarded as futile, partly because of their small size. Despite their small size, cilia possess a delicate architecture. The centriole first docks on the plasma membrane through its distal appendage and forms the basal body of cilia, which are approximately 200-nm wide and 300‒500-nm long. Cilia then build the transition zone, a diffusion barrier that gates the ciliary contents, and the membrane-encased nine-microtubule-based axoneme grows from the transition zone. Intraflagellar anterograde and retrograde transport shuttles ciliary components into and out of cilia, building cilia and maintaining their functions. The cilia diameter is approximately 80‒200 nm, and their lengths vary from 2 to 10 μm. For motile cilia, most have two microtubule central singlets and molecular motors, such as outer dynein arm(ODA)and inner dynein arm(IDA)complexes, surrounding the microtubule doublet and power the cilia beat, as shown in
4 Super-resolution microscopy boosts research
Sensory cilia have long been ignored until the discovery of their role in Hh signaling[36]. Although transmission electron microscopy(TEM)can distinguish different subciliary structures such as the axoneme and transition zone, it lacks molecular specificity and fails to reveal the spatial organization of the ciliary proteome, which is estimated to possess >200 proteins[37]. Motile cilia are a hot spot for cryo-electron microscopy/tomography(cryo-EM[38]/ET)[39], which have revealed the molecular architecture of the axoneme by averaging the periodic pattern along the axoneme[40]. However, this method uses purified cilia components and sacrifices the heterogeneity among microtubule doublets. Notably, the transition zone and ciliary membrane components have a highly organized architecture. Although conventional fluorescence microscopy can locate proteins in cilia, it fails to reveal protein organization within cilia due to resolution limit. To our delight, super-resolution imaging provides a powerful tool for interrogating these questions in situ. To date, SMLM, SIM, and STED have been successfully used to investigate the subciliary architecture, such as the diffusion barrier, basal foot, ciliary membrane, and axoneme. More recently, expansion microscopy and the combination of different super-resolution imaging techniques have emerged to further boost cilia research and aid the diagnosis of ciliopathies, such as Joubert syndrome(JS)and PCD.
4.1 Diffusion barrier
To maintain the cilia’s unique composition, a diffusion barrier gates the transport of ciliary components. The diffusion barrier sits at the base of cilia and comprises the transition zone, transition fiber, and the region in between. The transition zone is characterized by a Y link that connects the axoneme and ciliary membrane. Transition zone defects lead to ciliopathies, such as JS, Meckel-Gruber syndrome(MKS), and nephronophthisis(NPHP).
The transition zone contains at least 26 proteins[41-42], which can be grouped into three modules:MKS, NPHP, and CEP290. In 2015, Yang et al.[43] first investigated the organization of the transition zone components by STED, as shown in
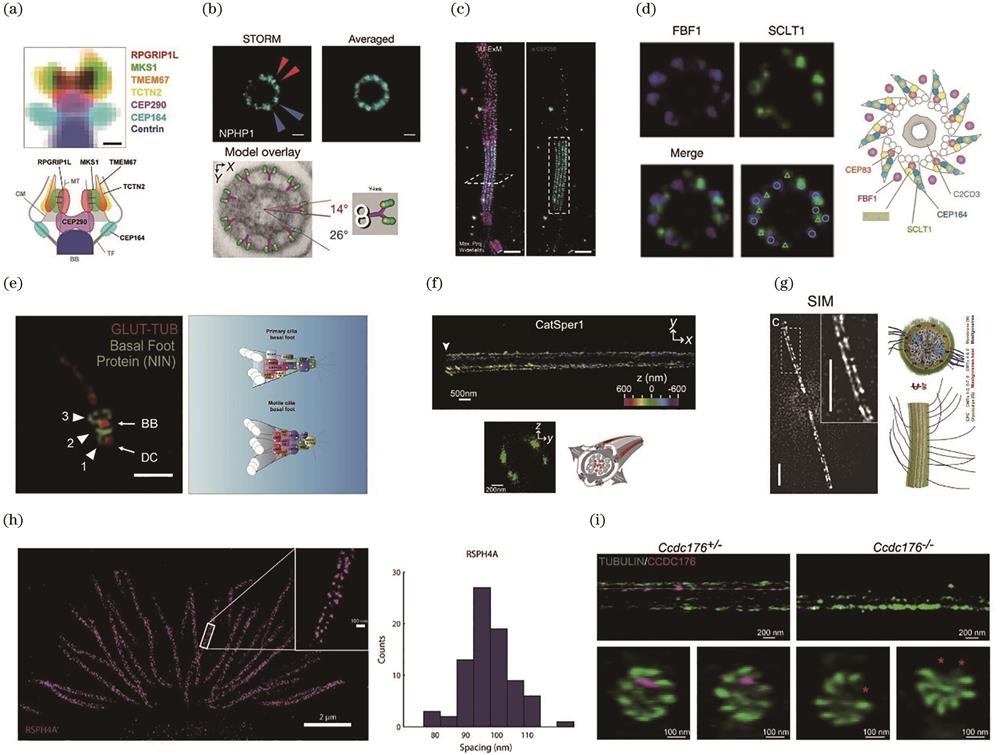
图 3.
Fig. 3. Super-resolution imaging resolves ciliary architecture. (a)‒(c) Transition zone architecture revealed by STED[43], STORM[44], and expansion microscopy[48]; (d) STORM reveals an unexpected matrix zone for the transition fiber[43]; (e) organization of basal foot proteins in both sensory and motile cilia revealed by three-dimensional (3D) SIM[50]; (f) STORM shows that CatSper1 forms four linear domains along the sperm flagella[55]; (g) SIM reveals that Chlamydomonas PKD2 forms two linear domains and associates with doublets 4 and 8[57]; (h) STORM reveals the 96-nm periodicity of the RS component RSPH4A[40]; (i) ROSE-Z shows that CCDC176 binds and stabilizes doublets 1 and 9[58]
Yang et al. also resolved the organization of the transition fiber, another diffusion barrier of cilia. Under TEM micrographs, the transition fiber appears as nine-fold blades emanating from the basal body. By examining 16 transition fiber components, they found that these proteins form a cone-shaped structure with proteins, such as FBF1, located in the space between the blades, a new structure not previously recognized by TEM, as shown in
4.2 Basal foot
The basal foot is a macromolecular structure of the basal body that originates from the subdistal appendage of the centriole. Unlike the transition fiber, which exhibits a 9-fold symmetry, the number of basal feet per cilium varies among different cell types and depends on different factors[50-51]. The sensory cilia basal foot participates in TGFbeta-signaling, and its components keep cilia merged in the ciliary pocket[52-53]. The basal feet of the motile cilia are important for coordinating the motile cilia beat. TEM reveals that the basal foot has a conical structure;however, how basal foot proteins are organized remains unclear. Nguyen et al.[50] reported the structure of the basal foot by examining its known components. Using 3D SIM, they found that the basal foot forms three modular regions:the basal foot anchoring domain, scaffolding domain, and MT anchoring domain[
4.3 Ciliary membrane protein
Membrane proteins have peculiar organizations, such as protein islands and functional domains. Recent research explored if ciliary membrane proteins have similar distributions. Yoon et al.[54] first examined the nanoscale morphology of primary cilia by imaging the ciliary membrane protein Smoothened(SMO). Through surface reconstruction, they found significant heterogeneity, such as deformations not visible through conventional fluorescence microscopy. Interestingly, some specific ciliary membrane proteins distributions were discovered along the axis of the sperm flagellum. CatSper is a sperm-specific calcium channel whose activation is critical for fertilization. Using STORM, Chung et al.[55] revealed that CatSper forms four strip-like domains along the flagellum, as shown in
4.4 Ciliary axoneme
The distribution of axoneme accessory proteins, such as ODAs and IDAs forms periodic structures. For instance, ODAs form 32-nm repeats, whereas IDAs, NDRC, and RS proteins form 96-nm repeats[24]. This rhythmic distribution is highlighted by cryo-EM/ET but not by fluorescence microscopy due to resolution limit. Liu et al.[40] first observed the 96-nm periodic pattern of the RS component RSPH4A[
5 Ciliopathy investigation and diagnosis using super-resolution microscopy
5.1 Joubert syndrome study using SIM
JS is a rare ciliopathy with symptoms of brain abnormalities characterized by the molar tooth sign under nuclear magnetic resonance imaging. JS is genetically heterogeneous, and a large portion of the disease-causative genes encode transition zone components. The molecular mechanism of JS is unclear. Using STORM, Shi et al. examined the fibroblast cilia transition zone from patients with JS bearing either TCTN2 or RPGRIP1L mutations. Interestingly, they found that the MKS and NPHP complexes are absent from the patients’ cells, suggesting that transition zone absence might be a common feature[
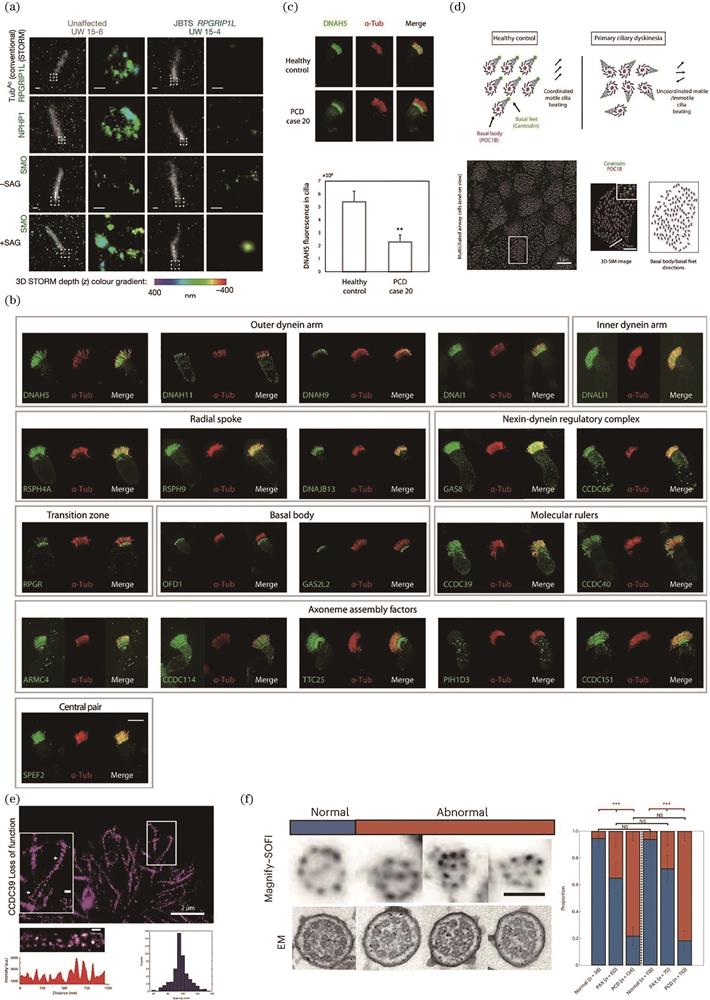
图 4.
Fig. 4. Super-resolution imaging aids ciliopathy investigation and diagnostics. (a) 3D SIM reveals the disruption of the transition zone and impairment of Hh signaling in patients with JS[44]; (b) 3D SIM-based PCD molecular fingerprint for PCD diagnosis[40]; (c) PCD diagnosis based on quantitative 3D SIM[40]; (d) PCD diagnosis based on rotational polarity[40]; (e) STORM reveals the preservation of 96-nm periodicity in CCDC39 loss of function cells[40]; (f) PCD diagnosis based on MAGNIFY-SOFI[65]
5.2 PCD investigation and diagnosis using 3D SIM and STORM
Though rare, direct application of super-resolution imaging in disease diagnosis is possible, and PCD is one of the few examples that demonstrate the potential of super-resolution imaging. In the remaining sections, we focus on motile ciliopathy PCD. We first introduce the traditional methods of PCD diagnosis and then illustrate how super-resolution imaging improves the diagnosis. Finally, we discuss how the diagnosis can be further optimized by integrating multiplexed labeling and high-speed video microscopy(HSVM).
5.3 Different diagnostic approaches for PCD
Early diagnosis is essential for initiating therapies to slow PCD progression because a delay in diagnosis may lead to lung collapse or transplantation, threatening patients’ lives. However, for a long time, PCD has been underdiagnosed because of its genetic heterogeneity, technical limitations, and lack of awareness. It may take years for patients with PCD to receive a confirmed diagnosis. Currently, two different methods are used to conclude the diagnosis:TEM and/or DNA sequencing. Even when the two techniques are combined, ~30% of patients with PCD clinical symptoms do not receive a confirmed diagnosis[59-60]. Unfortunately, a standalone approach for PCD diagnosis does not exist.
TEM was once regarded as the gold standard for PCD confirmation, specifically for ciliary ultrastructure defects. However, TEM mainly examines the absence of ODAs and/or IDAs, microtubule disorganization, and central pair defects and may introduce false-negative results. Generally, next-generation sequencing platforms enable the simultaneous querying of multiple exons, and compared with other methods, molecular genetic testing is an easy and quick option. However, unexpected mutations may occur in new PCD genes and untranslated regions, which can only be detected using the more expensive and time-consuming whole genome sequencing. Additionally, for known PCD causative genes, the mutations could be variants of unknown significance(VUS), which are common and increase the complexity of the diagnosis.
Two other techniques have recently emerged to aid PCD diagnosis:HSVM and fluorescence microscopy. Ciliary beat information can be extracted from the HSVM of multiciliated cells;however, it is insufficient to confirm PCD. Most PCD diagnostics only examine the cilia beat frequency but do not adequately differentiate PCD because the cilia beat amplitude, waveform, and coordination are vital to evaluating the ciliary beat. Additionally, chronic infections can disable ciliary motility, leading to false-positive diagnosis.
5.4 PCD diagnosis using fluorescence microscopy and 3D SIM
For most motile ciliopathies, molecular defects impair the assembly of the sophisticated axoneme of cilia. Immunofluorescence can help illustrate the absence of these components and contribute to the diagnosis. Shoemark etal.[61] reported that fluorescence microscopy cannot be applied as a single standard for PCD diagnosis after examining 35 patients using 6 antibodies. However, their study was limited by the number of PCD antibodies, microscope resolution, and absence of image quantification.
To further validate the fluorescence microscopy-based diagnosis and increase the resolution, Liu et al. first generated a molecular fingerprint of ~25 PCD proteins by staining control multiciliated cells and using 3D SIM, as shown in
To test whether 3D SIM and antibody panel staining combination can be developed as an independent diagnostic tool, the authors further tested two patients with different antibody panels. The first patient with DNAH5 and DNAH11 mutations was diagnosed with 15 PCD protein antibodies, and the result suggested the loss of all ODA components:DNAH5, DNAH11, and DNAI1. The second patient, with mutations in DNAH11 and TEM inconclusive, was diagnosed with 10 PCD protein antibody panels, and the result suggested the absence of DNAH11.
When cilia beat coordinately, the basal feet point toward the direction of the cilia beat, a phenomenon called rotational polarity[62]. For patients with PCD, cilia lose beat or beat coordination;consequently, rotational polarity is disrupted. Rotational polarity can be measured by staining the basal body and foot markers. However, because of the close distance between the basal body and foot, conventional microscopy fails to distinguish the basal foot from the basal body. Liu et al.[40] showed clear basal foot signal separation from the basal body using 3D SIM[
Antibody panel and quantitative 3D SIM-based diagnosis is straightforward and is likely to be implemented in clinical settings. Restricted by the availability of patients’ cells, this study only examined 31 patients with the most common PCD mutations. The sensitivity and robustness of this method require more testing of more patients and PCD mutations in the future. Note that the more antibodies in the panel, the more patient cells and labor required. To address this issue, an antibody multiplexed labeling strategy is required to optimize diagnostics in the future.
5.5 PCD investigation using 3D SIM and STORM
To further investigate whether super-resolution imaging can detect structural defects in patients with PCD, this study[40] focuses on patients with CCDC39 mutations. CCDC39[63] is the human homolog of Chlamydomonas fap59, a molecular ruler of motile cilia that determines the transport of IDA and NDRC into cilia and the arrangement of the 96-nm periodicity of RS components[64]. Whether CCDC39 has the same function in human multiciliated cells was a point raised. The authors assessed the motile cilia from three patients with CCDC39 loss of function using 3D SIM and found that the IDA component DNALI1 and NDRC component GAS8 are trapped at the transition fiber rather than the transition zone. They further employed STORM to check the periodicity of RSPH4A and found that, unlike Chlamydomonas, it is unaffected after loss of CCDC39 in these three patients, whereas motile cilia present disorganized microtubules, as shown in
5.6 PCD diagnosis using MAGNIFY-SOFI
3D SIM-based diagnosis relies on sophisticated microscopes, which may not be accessible in many hospitals/institutes. Contrarily, expansion microscopy and SOFI can be performed using a regular fluorescence/confocal microscope.
To explore the possibility of applying expansion microscopy and SOFI to PCD diagnosis, Klimas et al.[65] developed an optimized expansion technique named MAGNIFY that expands the specimens up to 11-fold. When combined with second-order SOFI, MAGNIFY can achieve a resolution down to ~15 nm. They first used this technique to check motile cilia, and using a pan staining strategy that labels all proteins within a cell, the axoneme 9+2 structure was observed, and found to be similar to TEM micrographs shown in
This study provides an economical and speedy method for assessing the motile cilia architecture using super-resolution microscopy instead of TEM. TEM requires cutting samples into thin cross-sections and examining different cross-sections to locate motile cilia. MAGNIFY-SOFI is fluorescence imaging-based and does not involve sample cutting. However, this study was limited to pan staining of the entire motile cilia proteome. As molecular specificity is one major advantage of fluorescence microscopy, it would be encouraging to observe different components of the cilia axoneme stained by different antibodies and to compare the differences between healthy controls and patients with PCD in the future.
6 Future perspectives on ciliopathy diagnosis
Despite the exciting progress made by the two groups, disease diagnosis using super-resolution microscopy is still at a preliminary stage.
One major challenge is the heterogeneity of the disease and the scarcity of patients. With the application of CRISPR KO in human airway cells, it would be helpful to test new and compare different diagnostics using CRISPR KO multiciliated cells[66]. The second challenge is the sensitivity of the antibody panel. New antibodies can be added to the panel but with increased requirements for patient samples and labor. Alternatively, multiplexed labeling approaches, such as DNA-PAINT[8], which can probe tens of targets easily and with single-cell sensitivity, can be adopted. The third challenge is data interpretation because antibody panel imaging produces large amounts of data. We anticipate that neural network-based image analysis can be applied to predict the results in the future.
Although the structure of cilia is well studied, their motility is only well characterized in a few model organisms and sperm cells. HSVM records ciliary motility with a high temporal resolution, and additional cilia beat parameters can be extracted to aid diagnosis. HSVM is also restricted by light microscopy resolution and sensitive cilia beat analysis which requires isolating a single cilium. Therefore, it is worthwhile to label motile cilia with fluorescent markers and apply high-speed super-resolution imaging techniques to distinguish single cilia for high-quality data analysis. Integrating cilia beat and antibody panel data will provide a more comprehensive characterization of cilia.
7 Summary
Super-resolution imaging has demonstrated its effectiveness in revealing the ciliary structure and diagnosing motile ciliopathy. The new generation of super-resolution imaging techniques that achieve nano- or angstrom spatial resolution and unprecedented time resolution are expected to revolutionize this field. High-speed super-resolution tracking techniques, such as MINFLUX[19] or MINSTED[67], can be applied to study cilia trafficking and cilia motor dynamics with single-molecule sensitivity. Combination of super-resolution imaging with other techniques, such as CryoET/CryoEM and CRISPR perturbation[68], to study the structural and functional changes in cilia in ciliopathies is also anticipated.
Regarding ciliopathy diagnosis, the sensitivity and accuracy of super-resolution imaging will be further evaluated by integrating patient data and checking CRISPR loss of function cells. The protocols can be further developed and optimized to facilitate diagnosis. Given that motile cilia are such sophisticated structures that they may reflect any cilia defects and multiciliated cells are easily accessible, other ciliopathies, such as sensory ciliopathies, can be possibly diagnosed by examining airway multiciliated cells.
Article Outline
, . Super-Resolution Fluorescence Microscopy for Cilia Investigation and Ciliopathy Diagnosis (Invited)[J]. 激光与光电子学进展, 2024, 61(6): 0618016. Zhen Liu, Yang Wu. Super-Resolution Fluorescence Microscopy for Cilia Investigation and Ciliopathy Diagnosis (Invited)[J]. Laser & Optoelectronics Progress, 2024, 61(6): 0618016.