基于非均匀采样的五棱镜阵列扫描测量大口径准直波前
下载: 681次
Collimated wavefront is an important parameter to estimate the quality of optical systems such as collimator, interferometer, and camera lens. Pentaprism scanning method is a conventional method to detect large aperture collimated wavefront by continuously collecting the coordinates of the focus of sub-aperture beams along the scanning direction and retrieving the wavefront distribution from the slope of the wavefront. The traditional pentaprism scanning method can only measure the wavefront distribution on one line of the collimated wavefront, and cannot obtain the full aperture aberration distribution of the wavefront. In order to obtain the full aperture aberration distribution of the wavefront, it is necessary to conduct multiple scans to obtain the slopes of multiple lines of the wavefront. This scanning method is often used for the surface shape detection of large aperture flat optical elements, through rotating the optical flat and measuring the slope of the surface in polar coordinates. However, the method is not suitable for measuring the collimated wavefront of the optical system. To measure the full aperture collimated wavefront, two pentaprism scanning systems in the X and Y directions, respectively, are generally required. The scanning mechanisms are complex, time-consuming, and vulnerable to environmental disturbances. In this paper, pentaprism array is proposed to simplify the scanning mechanism, shorten the scanning detection time of the full aperture collimated wavefront, and realize the rapid detection of the collimated wavefront.
Based on the traditional one-dimensional pentaprism scanning method, we propose to add multiple pentaprisms to form a group of parallel pentaprisms and a group of series pentaprisms, and realize a pentaprism array scanning based on nonuniform sampling to measure the three-dimensional distribution of large aperture collimated wavefront. Three parallel pentaprisms parallel to the scanning direction are used to measure the slope of the collimated wavefront in the X direction, and simultaneously three series pentaprisms perpendicular to the scanning direction are used to measure the slope of the collimated wavefront in the Y direction. All the pentaprisms move along the scanning direction simultaneously. Thus, this method only needs one scanning measurement process to obtain the slopes of the three lines of the wavefront simultaneously. It avoids the process of multiple scanning in the X and Y directions when measuring the full aperture collimated wavefront by the traditional method. The measured slope is fitted with the derivative function of the 4th11th terms in the Zernike polynomials, and then the three-dimensional distribution of the collimated wavefront is represented by the Zernike polynomials. Only low order aberrations of the wavefront can be fitted since the low sampling rate in the Y direction.
The feasibility and accuracy of using the slopes of the three lines of the wavefront to reconstruct the wavefront are verified by simulations, in which the reconstruction errors of the collimated wavefront with the pentaprism array are peak-to-valley (PV) value of 0.058λ and root-mean-square (RMS) value of 0.009λ (Fig. 3). The analysis for robustness of the proposed method is also given (Fig. 4). The proposed method is used to detect the collimated wavefront with the aperture of 1 m (Fig. 5). After correcting the mechanical scanning errors, the PV and RMS values of the measured wavefront are 2.495λ and 0.448λ, respectively. As a comparison, detection of the wavefront is also carried out with interferometry, with which the PV and RMS values of the measured wavefront are 2.752λ and 0.496λ, respectively. The retrieved collimated wavefront results obtained by the two methods are basically consistent, with nearly the same values and distribution form, which proves the feasibility of this method (Fig. 9). The uncertainty analysis of the pentaprism array scanning method is carried out, including uncertainty of spot centroid detection, uncertainty of the rail straightness and uncertainty of environmental disturbance. The combined uncertainty of the proposed method is 0.035λ.
Based on the traditional pentaprism scanning method, in this paper we propose a pentaprism array scanning method based on nonuniform sampling to measure the large aperture collimated wavefront. It solves the problem of complex and time-consuming scanning mechanism for the traditional pentaprism scanning method to detect the full aperture three-dimensional aberration distribution of large aperture collimated wavefront. This method measures the slope of the collimated wavefront in the X direction through three pentaprisms in parallel and the slope in the Y direction through three pentaprisms in series, and fits the low order aberration of the wavefront through the derivative of Zernike polynomials. The proposed method is simulated and analyzed, and the collimated wavefront with the aperture of 1 m is detected. The detection results are compared with the collimated wavefront measured by interferometry. The low order aberration distributions of the collimated wavefront measured by the two methods are basically consistent with the numerical value, which proves the feasibility and accuracy of the proposed method. The uncertainty analysis of the pentaprism array scanning method is also carried out, which demonstrates the measurement accuracy of the proposed method.
1 引言
对于平行光管、干涉仪、相机镜头等光学系统而言,准直波前是衡量光学系统质量的重要指标。常用的准直波前检测方法有干涉检测法[1-3]、哈特曼法[4]、夏克-哈特曼法[5-6]等。这些检测方法往往需要与待测准直波前口径相当的附加元件,检测成本较高。五棱镜扫描法通过测量准直波前斜率的变化,实现对大口径准直波前的扫描检测[7-9]。五棱镜扫描法光学结构简单,成本低,对于准直波前中低阶像差的检测具有重要价值。
传统的五棱镜扫描法通过单个五棱镜扫描待测波前一条线上的斜率从而复原准直波前在该条线上的波前分布。刘兆栋等[10]采用该方法对大口径近红外干涉仪的准直波前进行了检测,并对检测误差进行了详细分析。为了提高五棱镜扫描法的稳定性,减小五棱镜运动误差的影响,研究人员提出了差分五棱镜扫描法[11]、双五棱镜扫描法[12-13]等改进方法。这些方法极大地提高了五棱镜扫描法的检测精度,但是都只能测量准直波前一条线上的波前分布,无法得到待测波前全口径的像差分布。为了获得全口径的三维波面分布,需要进行多次扫描,获得待测波前多条线上的斜率。这种多条线的扫描方式常被用于大口径平面光学元件的面形检测[14-17],通过旋转待测光学元件,在极坐标下扫描测量面形多条线上的斜率,再通过Zernike多项式系数拟合的方式,实现对大口径平面光学元件全口径的三维面形检测。但旋转待测件的方式不适用于测量光学系统的准直波前。
若要测量全口径准直波前,一般需要X、Y两个方向的五棱镜扫描系统,扫描机构复杂,并且耗时长,易受环境扰动的影响。若能够只用一个X方向的扫描机构完成准直波前X、Y两个方向的斜率测量,则能简化扫描机构,缩短扫描时间。考虑Y方向斜率依靠多个五棱镜组合完成采样,X方向仍依靠扫描完成采样,即可形成X方向和Y方向的非均匀采样。本文提出在扫描机构上用三个平行于扫描方向的并联五棱镜测量X方向的斜率,三个垂直于扫描方向的串联五棱镜测量Y方向的斜率,所有五棱镜随机械扫描机构同时运动,只需要进行一次X方向的扫描测量就可以复原全口径的准直波前三维分布。该方法极大地减少了五棱镜扫描法检测全口径准直波前需要的扫描时间。
2 基本原理
2.1 五棱镜扫描法检测原理
五棱镜扫描法的原理如
五棱镜由位置1移动到位置2,光斑在探测器上移动的距离为Δc,会聚透镜的焦距为f,则待测波前在位置1和位置2处X方向的斜率差Δkx由下式可得:
若五棱镜以位置1作为起始点沿X方向对待测波前上每个点进行扫描,则待测波前上每个位置处的斜率与位置1处的斜率之差都可以计算得到。
2.2 非均匀采样的五棱镜阵列原理
由2.1可知,单个五棱镜沿一个方向扫描只能获得待测波前一条线上的斜率变化,为了得到待测波前全口径的斜率分布,需要分别测量待测波前多条线上的X方向和Y方向斜率。本文提出一种非均匀采样的五棱镜阵列,Y方向仅采样三个位置的斜率,X方向通过扫描采样多个位置的斜率,一次扫描就可以同时获得待测波前三条线上的X方向和Y方向斜率。其原理如
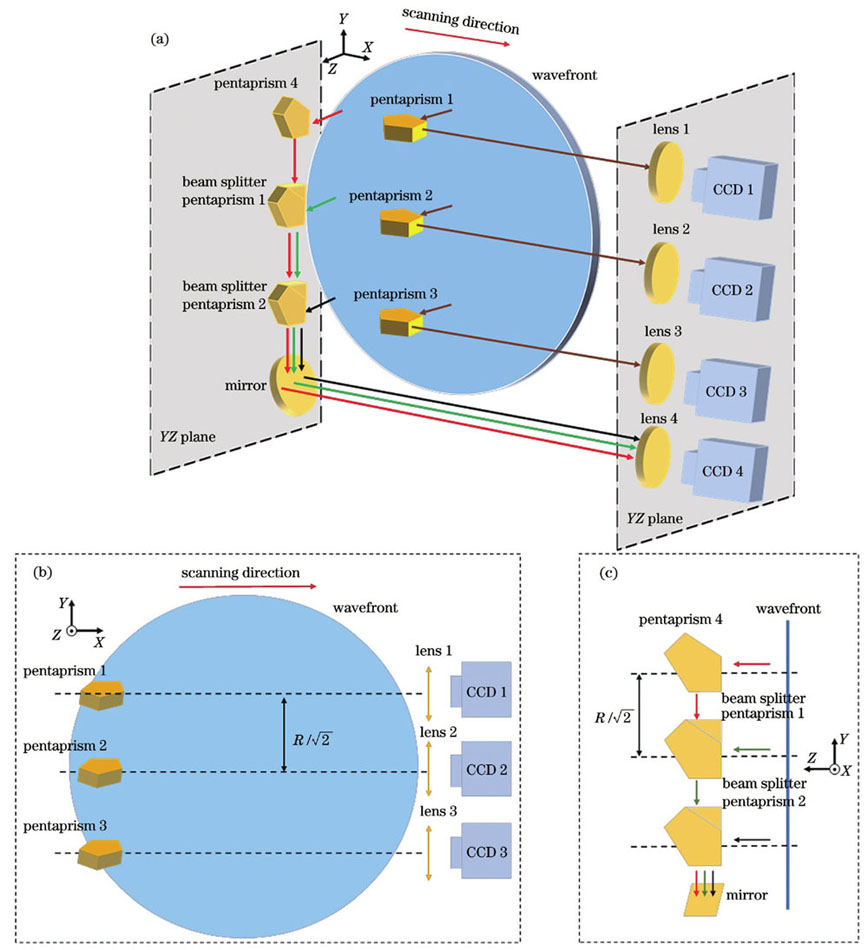
图 2. 非均匀采样的五棱镜阵列原理图。(a)非均匀采样的五棱镜阵列原理三维示意图;(b)X方向斜率测量原理;(c)Y方向斜率测量原理
Fig. 2. Principle of nonuniform sampling pentaprism array. (a) Three-dimensional diagram of principle of nonuniform sampling pentaprism array; (b) principle of slope measurement in X direction; (c) principle of slope measurement in Y direction
三个并联的五棱镜测量X方向斜率的原理如
三个串联的五棱镜测量Y方向斜率的原理如
2.3 复原准直波前三维分布的原理
准直波前W(x,y)可以用Zernike多项式表征:
对于五棱镜扫描系统而言,其扫描方向可以表示为
因此,待测准直波前沿扫描方向的斜率g(x,y,θ)可以表征为
五棱镜扫描法获得的数据为离散扫描点与起始位置点波前斜率的差值,因此该离散点的数据可以表征为
可由最小二乘法计算获得式(5)中Zernike多项式的系数:
待测准直波前可由Zernike多项式系数利用式(2)计算得到。准直波前一般只需要考虑其低阶像差,因此采用Zernike多项中的低阶像差项即4~11项即可。由于45°像散的Zernike多项式为xy,待测波前上任意点的一阶偏导数与起始点的一阶偏导数作差之后等于0,因此该五棱镜阵列法在复原待测波前时无法求解其45°像散大小。考虑到大口径准直系统中,像散一般是由于大口径的光学元件支撑或者光源偏心造成的,多表现为0°像散与45°像散的叠加,因此检测到0°像散后可以判断出系统的问题并指导装调。
3 仿真分析
实际检测系统的准直波前以低阶像差为主。为了验证非均匀采样的五棱镜阵列法复原准直波前三维分布的可行性与准确性,通过Zernike多项式的4~11项仿真一个待测的准直波前,并按照
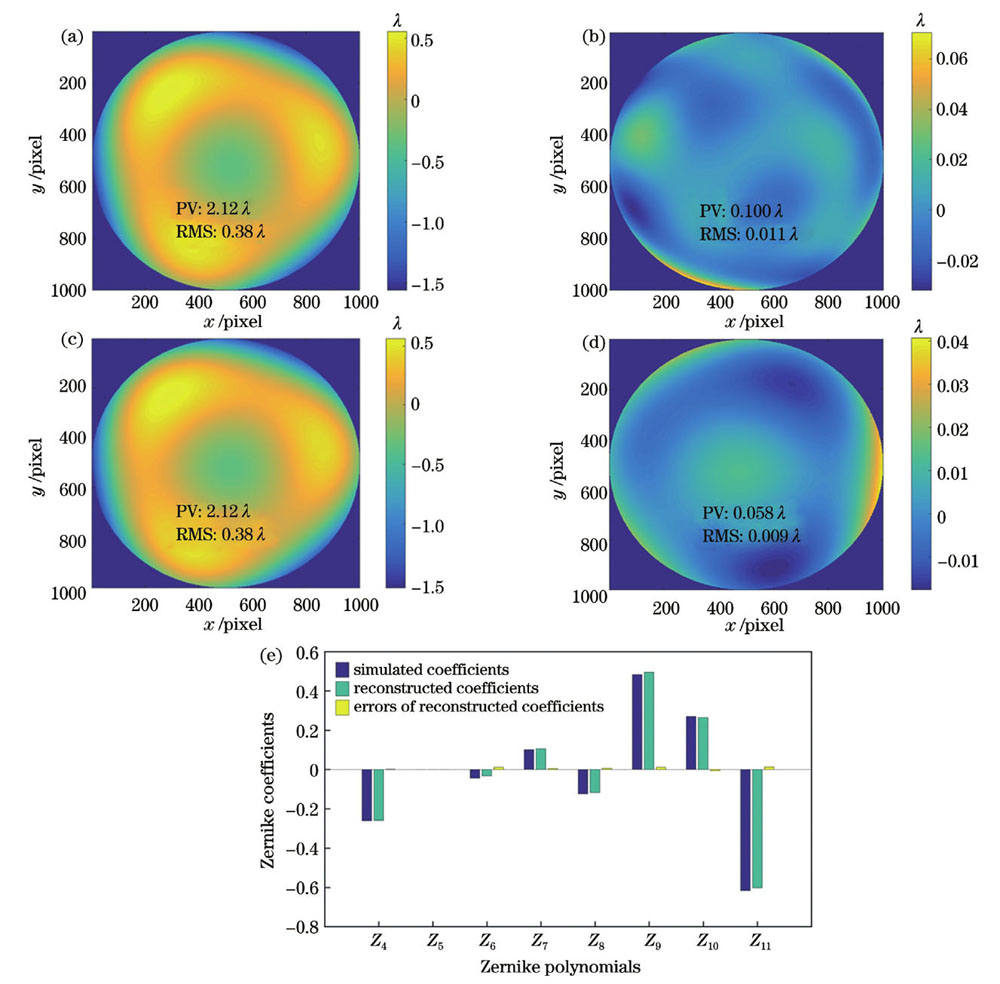
图 3. 五棱镜阵列复原待测波前。(a)仿真的准直波前;(b)仿真的准直波前中的随机误差;(c)复原的准直波前;(d)准直波前复原误差;(e)仿真的Zernike多项式系数与复原的Zernike多项式系数
Fig. 3. Reconstruction of wavefront with pentaprism array. (a) Simulated collimated wavefront; (b) random error in simulated collimated wavefront; (c) reconstructed collimated wavefront; (d) errors of reconstructed collimated wavefront; (e) simulated and reconstructed Zernike polynomial coefficients
可以看出用待测波前三条线上斜率复原的准直波前与仿真的准直波前像差分布一致,Zernike多项式系数也基本一致。仿真的准直波前PV值为2.12λ,均方根(RMS)值为0.38λ,复原的准直波前PV值为2.10λ,RMS值为0.37λ。将复原的准直波前与仿真的准直波前点对点相减后得到的复原误差PV值为0.058λ,RMS值为0.009λ,该方法复原的准直波前结果准确。为了证明该方法对任意准直波前都具有较高的复原精度,对其鲁棒性进行了仿真,每次仿真随机给定待测波前的Zernike多项式4~11项的系数,并且每次添加随机误差,比较复原出的准直波前与仿真的准直波前误差的PV值与RMS值。仿真了500次准直波前的复原误差,结果如
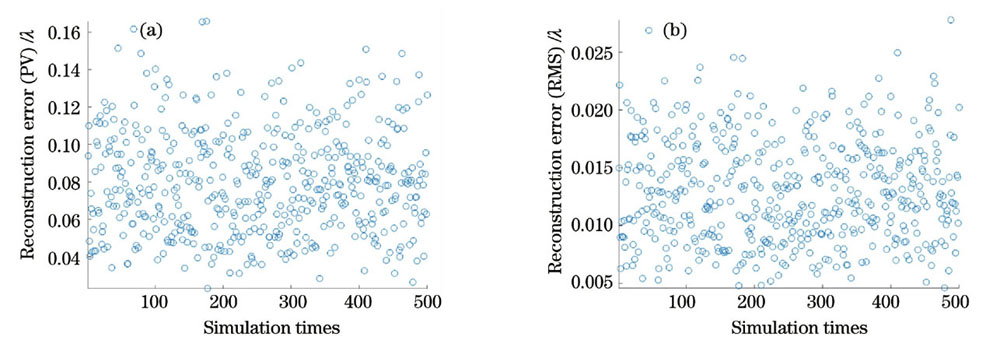
图 4. 复原准直波前的鲁棒性。(a)误差PV值;(b)误差RMS值
Fig. 4. Robustness of reconstructing collimated wavefront. (a) PV values of errors; (b) RMS values of errors
4 实验
4.1 五棱镜阵列扫描实验
为了验证本文方法的可行性,对1 m口径的准直波前进行了实验测量,准直波前波长为632.8 nm。实验装置如
实验中待测波前传播方向为Z轴正方向,波前圆心为坐标原点,沿待测波前X轴正方向扫描。待测波前半径为500 mm,则扫描待测波前三条线的Y坐标位置分别为y1=354 mm,y0=0,y-1=-354 mm。由于y1与y-1位置处的两条线上点的X方向坐标范围为-354~354 mm,因此实验中机械扫描范围为X方向坐标-354~354 mm,每隔25 mm测量一次,每次采集5幅图做平均之后用于计算光斑质心,经过二值化与插值处理后再根据质心计算公式获得每个采样点的质心在CCD上的像素位置。将每个扫描点的质心位置与起始位置的质心位置作差后得到质心的变化量。待测波前三条线上X方向测得的光斑质心移动量如
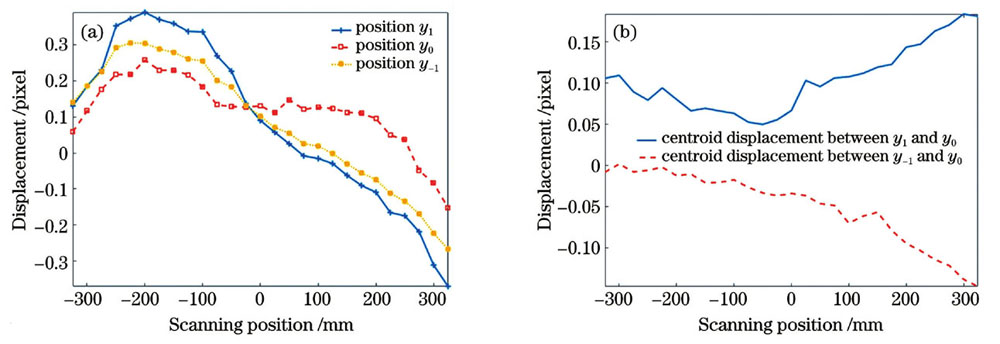
图 6. 五棱镜阵列扫描测量的光斑质心移动量。(a)X方向移动量;(b)Y方向移动量
Fig. 6. Spot centroid displacement measured with pentaprism array scanning. (a) Spot centroid displacement in X direction; (b) spot centroid displacement in Y direction
该实验数据需要考虑扫描过程中机械导轨的俯仰倾斜以及偏摆角对光斑质心位置的影响。根据五棱镜扫描法的机械扫描误差分析可知[18],在X方向斜率的扫描过程中,五棱镜测量精度只受到机械导轨的滚转角影响。当机械导轨存在一个滚转角β时,五棱镜测量的斜率误差为tan βsin β,因此实验中需要将机械导轨在测量点处的滚转角测量出来。测量点处的导轨滚转角如
对于Y方向斜率测量的数据,由于计算的是每个测量点处上下两个五棱镜与中间五棱镜的光斑质心的偏差,导轨的俯仰、偏摆和滚转角作差之后抵消掉了,因此Y方向的测量数据不需要考虑导轨的影响。综上所述,在测量数据中,X方向的斜率数据需要扣除导轨的滚转角误差。消除机械误差后的数据如
用校正后的数据复原待测波前,结果如
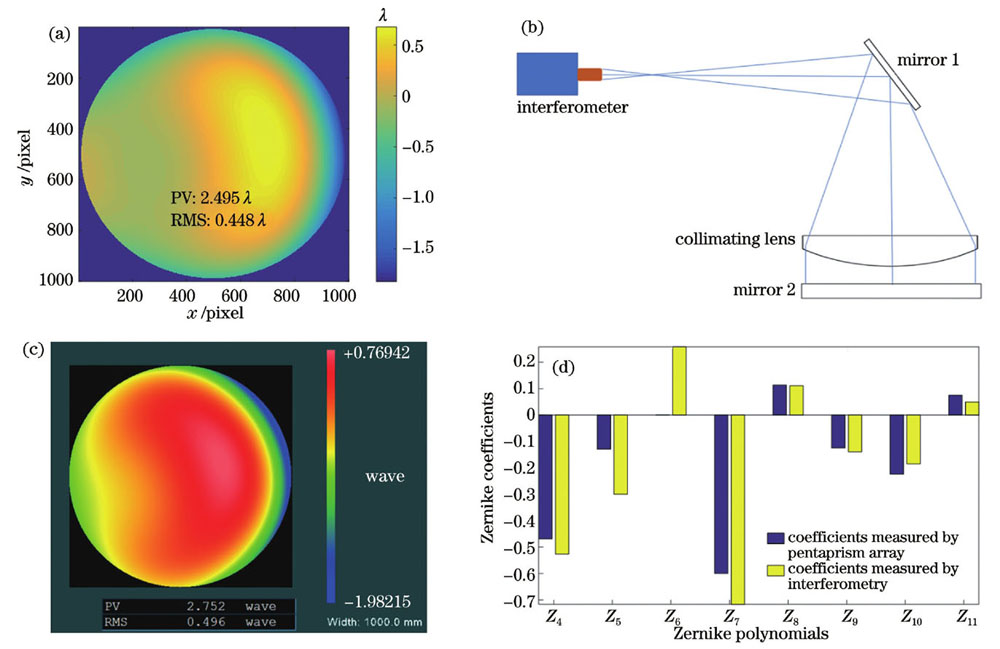
图 9. 五棱镜阵列扫描测量结果与干涉法测量结果比较。(a)五棱镜阵列扫描测量结果;(b)干涉法检测光路;(c)干涉法测量结果;(d)两种方法测量结果的Zernike多项式系数
Fig. 9. Comparison between pentaprism array scanning measurement results and interferometric measurement results. (a) Pentaprism array scanning measurement results; (b) optical path of interferometric test; (c) interferometric measurement results; (d) Zernike polynomial coefficients of measurement results
4.2 五棱镜阵列扫描法不确定度分析
首先分析光斑质心探测不确定度。本实验采用二值化与插值处理后再通过加权质心计算方法获得每个采样点的质心在CCD上的像素位置,该方法质心的探测不确定度为0.15 pixel[12]。实验采用的CCD像素大小为6.45 μm,会聚透镜焦距300 mm,因此可以得到由光斑质心不确定度造成的波前斜率测量不确定度为
其次分析导轨直线度不确定度。实验中针对导轨的直线度进行了测量,并在实验数据中扣除了导轨的直线度影响,因此,导轨的直线度不确定度取决于测量方法的不确定度。本文采用的导轨直线度测量方法不确定度为[19-20]
接下来讨论环境扰动不确定度。实验中为了减小环境扰动,使五棱镜扫描装置与被测系统尽可能贴近,且将它们放置在同一个隔振平台上,环境温度控制为(20±0.2)℃。在10 min内,通过单个五棱镜在固定位置监测光斑质心在CCD上的漂移,质心位置漂移量最大0.1 pixel。因此,环境扰动的不确定度为
最后仿真分析五棱镜阵列的不确定度。综合以上不确度分析可以得到五棱镜阵列上单个五棱镜的合成不确定度。由于Y方向的三个五棱镜不含有导轨直线度的不确定度,因此,X方向的五棱镜和Y方向的五棱镜合成不确定度分别为
按照第3节中的仿真方法,在采样的待测面形斜率数据上引入X方向和Y方向测量斜率的不确定度。综合上述不确定度进行仿真,得到复原的波前误差RMS值作为五棱镜阵列的测量不确定度[16]。仿真100次的最大波前误差结果值为0.035λ,因此五棱镜阵列扫描法的不确定度为0.035λ。
5 结论
本文在传统的五棱镜扫描法基础上,提出了基于非均匀采样的五棱镜阵列扫描测量大口径准直波前的方法,解决了传统五棱镜扫描法检测大口径准直波前全口径三维像差分布时扫描机构复杂且时间冗长的问题。该方法通过三个并联的五棱镜测量准直波前X方向斜率,通过三个串联的五棱镜测量Y方向斜率,并通过Zernike多项式的一阶导数拟合出待测波前的低阶像差。对该方法进行了仿真分析,并检测了1 m口径的准直波前,检测结果与采用干涉法测量的结果进行了比较,两种方法测量的准直波前低阶像差分布与数值基本一致,证明了该方法的可行性与准确性。
[1] Prakash S, Rana S, Prakash S, et al. Automated collimation testing using a temporal phase shifting technique in Talbot interferometry[J]. Applied Optics, 2008, 47(31): 5938-5943.
[2] Wang Y, Zhai H C, Jutamulia S, et al. Collimation test of a corrected laser diode beam using lateral shearing interferometer[J]. Optics Communications, 2007, 274(2): 412-416.
[3] 冯鹏, 唐锋, 王向朝, 等. 双孔点衍射干涉成像系统波像差检测技术研究[J]. 中国激光, 2021, 48(9): 0904002.
[4] Cordero-Davila A, Cornejo-Rodriguez A, Cardona-Nunez O. Null Hartmann and Ronchi-Hartmann tests[J]. Applied Optics, 1990, 29(31): 4618-4621.
[5] 吴伟, 罗自荣, 于乃辉, 等. 基于夏克-哈特曼传感器的计算机辅助装调方法[J]. 光学学报, 2021, 41(20): 2022001.
[6] 董玉磊, 韦承甫, 刘现魁, 等. 狭缝式自由旋涡气动窗口光学质量测量方法研究[J]. 中国激光, 2021, 48(23): 2304003.
[7] 华志远, 徐晨. 五棱镜扫描法检测大口径平行光管光束平行性[J]. 光学技术, 2021, 47(5): 582-586.
[8] Yellowhair J, Burge J H. Analysis of a scanning pentaprism system for measurements of large flat mirrors[J]. Applied Optics, 2007, 46(35): 8466-8474.
[9] MohammadiK, LeeY S, JenneweinT. Characterization of optical aberrations with scanning pentaprism for large collimators[C]//Conference on Lasers and Electro-Optics, May 15-20, 2022, San Jose, CA, USA. Washington, D.C.: Optica Publishing Group, 2022: ATh1D.6.
[10] 刘兆栋, 于丽娜, 韩志刚, 等. 五棱镜扫描法检测大口径近红外干涉仪准直波前[J]. 中国激光, 2010, 37(4): 1082-1087.
[11] 汤兆鑫, 黄玮, 许伟才, 等. 差分五棱镜扫描法在波前检测中的应用[J]. 中国光学, 2014, 7(6): 1003-1011.
[12] Mallik P C V, Zhao C Y, Burge J H. Measurement of a 2-meter flat using a pentaprism scanning system[J]. Optical Engineering, 2007, 46(2): 023602.
[13] Zhu X L, Wang D S, Nie F M, et al. Large-aperture real-time compensated collimating wavefront error detection method[J]. Applied Sciences, 2019, 9(11): 2370.
[14] 戚二辉, 罗霄, 郑立功, 等. 大口径非圆形光学平面的五棱镜扫描检测技术研究[J]. 光电子·激光, 2014, 25(7): 1370-1375.
Qi E H, Luo X, Zheng L G, et al. Optical testing of large optical flat mirror with non-circular pupil based on scanning pentaprism technology[J]. Journal of Optoelectronics·Laser, 2014, 25(7): 1370-1375.
[15] 袁理, 张晓辉. 采用五棱镜扫描法检测大口径平面镜的面形[J]. 中国光学, 2019, 12(4): 921-932.
Yuan L, Zhang X H. Surface shape measurement of large flat mirrors using a scanning pentaprism method[J]. Chinese Optics, 2019, 12(4): 921-932.
[16] Qi E H, Hu H X, Luo X. Study on low-order aberration measurements of large-aperture flats based on scanning pentaprism technology[J]. Applied Optics, 2019, 58(4): 787-793.
[17] 王孝坤, 戚二辉, 胡海翔, 等. 超大口径平面反射镜的光学检测(特邀)[J]. 红外与激光工程, 2022, 51(1): 20210953.
[18] 常山, 曹益平, 陈永权. 五棱镜的运动误差对波前测量的影响[J]. 光学仪器, 2005, 27(3): 12-16.
Chang S, Cao Y P, Chen Y Q. Kinematic error effect of pentagonal prism on wavefront measurement[J]. Optical Instruments, 2005, 27(3): 12-16.
[19] Tenjimbayashi K. Straightness measurement of a moving table by using laser beams[J]. Proceedings of SPIE, 2000, 4093: 47-57.
[20] Hsieh T H, Chen P Y, Jywe W Y, et al. A geometric error measurement system for linear guideway assembly and calibration[J]. Applied Sciences, 2019, 9(3): 574.
Article Outline
刘威剑, 高志山, 马燚岑, 车啸宇, 雷李华, 傅云霞, 袁群. 基于非均匀采样的五棱镜阵列扫描测量大口径准直波前[J]. 中国激光, 2022, 49(24): 2404001. Weijian Liu, Zhishan Gao, Yicen Ma, Xiaoyu Che, Lihua Lei, Yunxia Fu, Qun Yuan. Scanning Measurement of Large Aperture Collimated Wavefront with Pentaprism Array Based on Nonuniform Sampling[J]. Chinese Journal of Lasers, 2022, 49(24): 2404001.