锥形光纤SERS探针的批量制备和定量检测
Surface-enhanced Raman scattering (SERS) spectroscopy has significant applications in various fields such as food safety, environmental monitoring, and life sciences. In recent years, there has been growing interest in the quantitative detection of substance concentrations using SERS spectroscopy. SERS fiber probes, which offer outstanding practical value, enable in situ and on-site detection of substances in complex environments, making them highly suitable for practical quantitative measurements. However, because the reuse of fiber probes is challenging owing to contamination by the substances being measured, it is essential to prepare a large number of fiber probes that exhibit good interchangeability in batches. This allows the establishment of a statistical quantitative relationship between the spectral amplitude and substance concentration through large-sample spectral detection. This statistical quantitative relationship can thereafter be used to determine the concentrations of unknown samples. However, the interchangeability differences of fiber probes prepared from similar and different batches and the elimination or compensation for interchangeability degradation during batch preparation for quantitative detection have not yet been studied. In this study, we employ an electrostatic adsorption self-assembly method to prepare tapered SERS fiber probes. By assimilating, statistically averaging, and fitting large-sample spectral data measured from different batches of fiber probes, we obtain high-precision quantitative curves and achieve quantitative detection of thiram samples.
A batch of bare tapered fibers is fixed onto a specially designed disc. Subsequently, a monolayer of uniformly distributed gold nanospheres is grown on the surface of these fibers at the same density under the optimized electrostatic adsorption self-assembly conditions. Subsequently, a batch of tapered SERS fiber probes with excellent interchangeability is obtained. Tapered SERS fiber probes are prepared by repeating the same preparation process under identical conditions. During the testing stage, fiber probes from the same batch, which exhibit good interchangeability, are initially used to individually test a series of thiram standard solutions with varying concentrations. The spectral data obtained from these single tests are thereafter fitted to establish a quantitative relationship between the spectral amplitude and the sample concentration for that particular batch. Subsequently, spectral data obtained from single tests using different batch probes are fitted to obtain a quantitative relationship for each batch. Based on these relationships, spectral calibration factors are calculated to account for variations across different batches. Ultimately, the spectral data measured by the probes from different batches are assimilated into a single batch using calibration factors such that large-sample spectral data can be collected. Spectral data are statistically averaged and fitted to obtain a high-precision quantitative curve. The quantitative detection capability of this curve is assessed using recovery testing experiments.
The results reveal that fiber probes from the same batch exhibit good interchangeability, with the relative standard deviation (RSD) of the spectral amplitude measured for the same sample concentration being less than 8%. Fiber probes from different batches exhibit greater variability owing to inherent factors in the chemical growth process, with an RSD of 15% for the spectral amplitude measured for the same sample concentration (Fig.3). The quantitative relationship between the spectral amplitude measured by probes from the same batch and concentration is investigated, and the results indicate that for all batches of fiber probes measured, the spectral amplitudes measured by probes from a single batch follow a Langmuir function relationship with thiram concentration, but the quantitative relationships obtained are different for each batch (Fig.4). Using the spectral calibration factors obtained from the quantitative curves of single tests from each batch, ten batches of spectral data are successfully assimilated to the same batch level, and a high-precision quantitative relationship curve is obtained through statistical averaging and data fitting of the assimilated large-sample spectral data with a fitting degree of up to 0.999 (Fig.5). The quantitative curves obtained after assimilating the spectral data to the 1st, 4th, and 8th batches individually exhibit excellent quantitative detection capabilities, and the recovery rates for thiram-spiked samples at concentrations of 8×10-7 mol/L and 8×10-8 mol/L fall within the range of 90%?110% (Table 2).
In this study, we investigate the quantitative SERS detection performance of tapered fiber probes prepared in batches using an electrostatic adsorption self-assembly method. Under consistent preparation and detection conditions, different fiber probes from the same batch exhibit excellent interchangeability, with an RSD of the SERS spectral amplitude of less than 8% for thiram samples at the same concentration. To address the issue of the reduced interchangeability of fiber probes from different batches, which does not meet the demands for the number of probes needed in practical quantitative detection applications, we propose and demonstrate a method to assimilate the spectral data measured by probes from different batches to those of probes from a single batch. By statistically averaging and fitting the assimilated large-sample spectral data, we obtain a calibration curve for the SERS quantitative detection of thiram samples in the concentration range of 2×10-8?10-6 mol/L. Using this calibration curve, the recovery rates for tests on spiked thiram samples at concentrations of 8×10-7 mol/L and 8×10-8 mol/L reach 90%?110%. The proposed method for the batch preparation of tapered SERS fiber probes, the assimilation method of spectral data from probes prepared in different batches, and the scheme for obtaining high-precision quantitative detection curves through statistical averaging of large-sample spectral data are expected to provide references for practical SERS quantitative detection applications.
1 引言
表面增强拉曼散射(SERS)光谱检测技术在食品安全、环境监测及生命科学等领域中有着重要应用[1-5]。随着检测灵敏度的提高,利用SERS光谱技术实现物质浓度的定量检测逐渐成为研究热点。在SERS检测中,待测物质分子须吸附在SERS基底表面,这会污染SERS基底,使得其难以重复使用。因此,SERS定量检测需要大量可互换的SERS基底。但是,对于“热点”随机分布的高灵敏SERS基底,不同基底测得的光谱强度存在差异,即SERS基底的互换性差,导致基于这类SERS基底的定量检测的准确性难以保证[6]。尽管借助内标法可在一定程度上提高定量检测的准确性,但内标物须具有化学性质稳定及SERS光谱特征峰与待测物不重叠等特性,故内标法难以推广应用[7]。为此,人们改用刻蚀法[8]、纳米压印[9]和模板法[10-11]等微纳加工方法来制备结构规整有序的SERS基底,以提高SERS基底的互换性。目前,基于微纳加工制备的SERS基底,在同一样品浓度下测得SERS光谱强度的相对标准偏差(RSD)已达2%以下[12],SERS定量检测的准确度高。但是,微纳加工方法难以高效经济地制备大量可互换的SERS基底,不利于SERS定量检测的实际应用。
光纤SERS探针优点突出,通过与便携式拉曼光谱仪联用,可实现复杂环境中物质的原位现场检测[13]。随着光纤SERS探针检测灵敏度的不断提升[14-15],人们开始关注光纤SERS探针定量检测的可能性。但与SERS基底一样,表面“热点”随机分布的光纤SERS探针也存在互换性差的问题,影响SERS定量检测的准确性。因此,研究人员选用结构相对简单的平端面光纤探针,采用多种自组装方法,在其平端面上制备出相对均匀的纳米结构,以提高光纤SERS探针的互换性[16-18]。近年来,人们采用静电吸附等自组装方法,在弯曲光纤表面制备出具有不同形貌和尺度的纳米颗粒,如锥形光纤SERS探针。通过优化锥角,实现了光纤锥表面金属纳米颗粒的表面波激发,既避免了金属纳米颗粒的光热损伤,又增大了SERS相互作用的面积,锥形光纤SERS探针展现出大幅提升检测灵敏度的潜力[19-21]。因此人们开始考察锥形光纤SERS探针的定量检测问题[22]。目前,基于静电吸附自组装方法制备的不同光纤探针,测得SERS光谱强度的RSD可达10%以下[23],表明这种锥形光纤SERS探针具有较好的互换性。但是,在实际SERS定量检测应用中,必须制备出大量的具有良好互换性的光纤探针:一是因为光纤探针在检测过程中存在污染而不能重复使用;二是需要通过大样本光谱检测来获取光谱幅度与待测物质浓度之间的统计定量关系。但是,批量制备的锥形光纤探针的互换性、不同批次光纤探针之间的互换性差异,以及定量检测时消除或补偿这类批量制备带来的互换性退化等问题,尚需进一步深入研究。
本文研究了静电吸附自组装法批量制备的锥形光纤探针的SERS定量检测性能。在相同制备条件下,同一批次制备的锥形光纤SERS探针具有良好的互换性,而对于不同批次制备的光纤探针,由于化学制备过程中的固有影响因素,互换性降低。提出了一种光谱数据同化方法,将10批次光纤探针测得的福美双样品的SERS光谱幅度同化至同批次光纤探针测得的光谱幅度。对同化后所得到的大样本光谱数据进行统计平均和Langmuir函数拟合,获得了福美双样品的光谱幅度与浓度之间的定量关系曲线,实现了对未知浓度福美双样品的定量检测。
2 光纤探针的制备与测试
用于制备锥形光纤SERS探针的光纤为普通多模石英光纤,纤芯直径为200 µm,数值孔径(
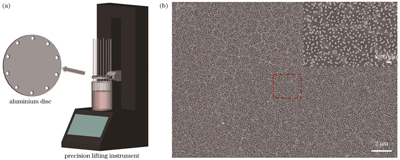
图 1. 锥形光纤SERS探针的批量制备装置和效果图。(a)带圆通孔的圆盘示意图,其中圆盘直径和厚度分别为10.0 mm和3.0 mm,圆通孔直径和间距分别为400.0 μm和2.5 mm;(b)2 h静电吸附后制备出的光纤SERS探针锥表面的金球颗粒分布情况,插图为虚线框区域的局部放大图
Fig. 1. Batch preparation device and effect diagram of tapered fiber SERS probes. (a) Schematic of disc with circular through holes, in which diameter and thickness of disk are 10.0 mm and 3.0 mm, and diameter and spacing of circular through holes are 400.0 μm and 2.5 mm respectively; (b) distribution of golden sphere particles on tapered surface of prepared fiber SERS probe after 2 h electrostatic adsorption with local enlarged picture of dotted box area shown in inset
然后,采用静电吸附自组装法,在固定在圆盘上的裸光纤锥表面上,均匀地单层生长球形金纳米颗粒。为充分利用颗粒的局域表面等离子体激元共振(LSPR)效应且便于在光纤锥表面上实现颗粒的均匀单层生长,我们选择直径为70 nm的球形金纳米颗粒制备光纤探针[19]。采用食人鱼溶液(质量分数为30%的过氧化氢与质量分数为98%的浓硫酸的体积比为3∶7)浸泡光纤锥1 h,使每根光纤表面的SiO2分子羟基化;将其置于羧基乙基硅烷三醇钠盐(CEOS)溶液(体积分数为20 µL/mL)中浸泡2 h,通过羟基之间的缩合反应,在光纤锥表面上实现了带负电的羧基基团的均匀分布;将表面带有均匀羧基基团的光纤锥置于由十六烷基三甲基氯化铵(CTAC)包覆且带正电的金球溶胶中,由于静电吸附,金球颗粒自组装至光纤锥表面,构成单层均匀分布的锥形光纤SERS探针。制备每批次光纤探针时金球溶胶的配置方法相同,且光纤探针浸入CEOS溶液和金球溶胶的深度均由升降平台精密控制为3 mm。通过改变静电吸附的时长,可批量制备出具有不同金球分布密度的锥形光纤探针,但在光纤锥表面金球颗粒的分布密度一致[19]。2 h静电吸附后制备出的光纤SERS探针锥表面的金球颗粒分布情况如
锥形光纤SERS探针的定量检测性能采用便携式拉曼光谱仪测试,实验装置如
3 结果与讨论
在实验中,根据前期研究结果[19],选定2 h静电吸附时间下制备的13°锥形光纤探针来研究SERS定量检测效果。该13°锥形光纤SERS探针可避免过大的光传输损耗和外部分子散射损耗,同时也不易在锥底部产生光折射而造成光热损伤,有利于实现整个锥面上SERS效应的高效率激发,测得的光谱幅度高,光谱稳定性好。为避免较大激发光功率引起的光谱幅度波动对后续光谱定量检测的干扰,选定激发光功率为18 mW。在本文后续部分,若无特殊说明,所用光纤SERS探针的锥角为13°,纳米颗粒吸附时间为2 h,激发光功率为18 mW。
采用不同批次制备的光纤探针(每批次10根),分别实测了10-6 mol/L福美双标准溶液的SERS光谱及其RSD。
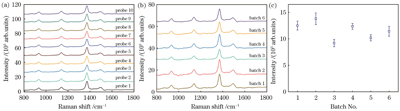
图 3. 锥形光纤SERS探针的检测效果。(a)同一批次10根锥形光纤探针测得的10-6 mol/L福美双标准溶液的SERS光谱;(b)6个不同批次探针测得的10-6 mol/L福美双标准溶液的平均SERS光谱;(c)6个不同批次探针测得的SERS平均光谱中的1386 cm-1拉曼峰光谱幅度及其标准差
Fig. 3. Detection results of tapered fiber SERS probes. (a) SERS spectra of 10-6 mol/L thiram standard solution measured by 10 tapered fiber probes in same batch; (b) average SERS spectra of 10-6 mol/L thiram standard solutions measured by 6 different batches of probes; (c) spectral amplitudes of Raman peaks at 1386 cm-1 and corresponding SDs in SERS average spectra measured by 6 different batches of probes
表 1. 光谱数据同化前后加标样品的检出浓度
Table 1. Detected concentrations of spiked samples before and after spectral data assimilation
|
表 2. 光谱数据同化前后的测试回收率
Table 2. Detected recovery rates before and after spectral data assimilation
|
当福美双溶液浓度分别为10-7 mol/L和5×10-8 mol/L时,任一批次的10根光纤探针测得的光谱与
由于同一批次制备的光纤探针所测的光谱幅度RSD小,且同一批次的光纤探针有10根,故可考察同一批次光纤探针所测的光谱幅度与浓度之间的定量关系。
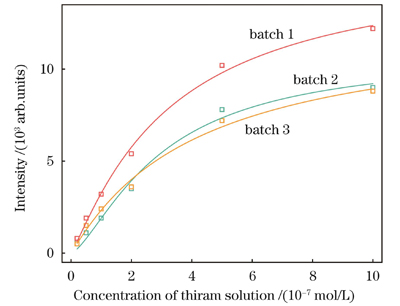
图 4. 福美双溶液的1386 cm-1拉曼特征峰光谱幅度的拟合曲线(方块为数据,线为拟合线)
Fig. 4. Fitted curves of spectral amplitude of Raman characteristic peak at 1386 cm-1 for thiram solutions (square is data and line is fitted line)
因此,应设法消除不同批次光纤探针的光谱检测定量关系之间的差异并增加光谱样本数目。首先同化不同批次光纤探针的定量检测光谱数据,以提高不同批次光谱检测结果之间的互换性。大量研究结果已表明,SERS定量检测所得的光谱幅度与样品浓度基本满足Langmuir函数关系。本文
为验证上述方法,我们制备了10批次光纤探针,利用每一批次的6根光纤探针,分别对6个福美双标准浓度点(10-6、5×10-7、2×10-7、10-7、5×10-8、2×10-8 mol/L)进行单次检测,获得了1386 cm-1拉曼特征峰的光谱数据,通过Langmuir函数
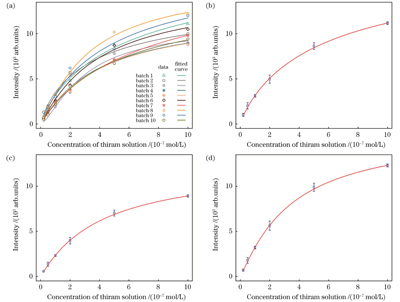
图 5. 光谱数据同化前后的定量曲线。(a)光谱数据同化前的定量曲线;以第(b)1、(c)4和(d)8批次光纤探针的定量关系曲线作为基准进行光谱数据同化后的定量曲线(方块为数据,线为拟合线)
Fig. 5. Quantitative curves before and after spectral data assimilation. (a) Quantitative curves before spectral data assimilation; quantitative curves after spectral data assimilation based on quantitative relationship curves of batch (b) 1, (c) 4 and (d) 8 fiber probes (square is data and line is fitted line)
进一步地,选定浓度为8×10-7 mol/L和8×10-8 mol/L的福美双标准溶液作为加标样品进行回收测试实验,以考察基于大样本光谱数据的定量曲线的实际定量检测能力。测试步骤如下:依次从前述10批次光纤探针中各取1根探针对同一浓度加标样品进行光谱测试,将每根探针(第j批)测得的1386 cm-1拉曼峰幅度与该批次的Langmuir曲线进行对照,获得单批次检出浓度值
以上是基于批量制备的光纤探针(每批次10根光纤探针)所得的定量检测结果,10根光纤探针数目依然有限,不利于实际定量检测。但是,通过调整装配光纤的圆盘规格,可以实现每批次20根及以上的互换性良好的锥形光纤SERS探针的批量制备。此时,若采用上述同化校准方法来获取大样本光谱数据和定量曲线,则可富余更多光纤探针供实际定量检测使用。因此,对于简单经济的静电吸附自组装法批量制备的锥形光纤SERS探针,在对不同批次光纤探针所测的光谱数据进行同化处理后,光纤探针具有良好的互换性,可提供大样本光谱数据以获取高精度定量检测定标曲线,这有望推动SERS定量检测技术的实用化进程。
4 结论
研究了基于静电吸附自组装法批量制备的锥形光纤探针的SERS定量检测性能。通过统一制备条件,静电吸附自组装法批量制备的同一批次锥形光纤探针展现出了良好的互换性,同一批次的不同光纤探针测得的同一浓度福美双样品的SERS光谱幅度的RSD可达8%以下。但化学制备过程中的固有影响因素会造成不同批次光纤探针之间的互换性降低,为满足实际定量检测应用对光纤探针数目的要求,必须提高不同批次光纤探针之间的互换性。通过光谱数据同化方法,可将不同批次光纤探针测得的光谱数据同化至某一批次光纤探针测得的光谱数据附近。利用这种光谱数据同化方法,对10批次光纤探针测得的福美双样品SERS光谱数据进行同化处理,得到大样本光谱数据,通过统计平均和Langmuir函数拟合,获得了浓度为2×10-8~10-6 mol/L的福美双样品的SERS定量检测的定标曲线。基于该定标曲线,浓度为8×10-7 mol/L和8×10-8 mol/L的两种福美双加标样品的测试回收率可达90%~110%。研究结果为实际SERS定量检测应用研究提供了参考。
[1] Zheng J K, He L L. Surface-enhanced Raman spectroscopy for the chemical analysis of food[J]. Comprehensive Reviews in Food Science and Food Safety, 2014, 13(3): 317-328.
[2] Pang S, Yang T X, He L L. Review of surface enhanced Raman spectroscopic (SERS) detection of synthetic chemical pesticides[J]. TrAC Trends in Analytical Chemistry, 2016, 85: 73-82.
[3] Pahlow S, Meisel S, Cialla-May D, et al. Isolation and identification of bacteria by means of Raman spectroscopy[J]. Advanced Drug Delivery Reviews, 2015, 89: 105-120.
[4] 赖春红, 赖林, 张芝峻, 等. 基于金纳米颗粒-半胱胺SERS基底的水中硝酸根检测[J]. 中国激光, 2022, 49(11): 1111002.
[5] 刘真真, 刘晓娴, 孙岩松, 等. 基于新型纳米材料的SERS免疫层析技术研究进展[J]. 光学学报, 2023, 43(17): 1712003.
[6] Chan Y F, Zhang C X, Wu Z L, et al. Ag dendritic nanostructures as ultrastable substrates for surface-enhanced Raman scattering[J]. Applied Physics Letters, 2013, 102(18): 183118.
[7] Zhao F T, Wang W P, Zhong H D, et al. Robust quantitative SERS analysis with Relative Raman scattering intensities[J]. Talanta, 2021, 221: 121465.
[8] Cai J Y, Wang Z Z, Jia S Y, et al. Si/TiO2/Ag multistorey structures with interfacial charge transfer for a recyclable surface-enhanced Raman scattering substrate[J]. ACS Applied Materials & Interfaces, 2022, 14(11): 13703-13712.
[9] Cai J Y, Liu R H, Jia S Y, et al. SERS hotspots distribution of the highly ordered noble metal arrays on flexible substrates[J]. Optical Materials, 2021, 122: 111779.
[10] Liu C, Wu J, Wang S, et al. Directional controllable electrodeposition growth of homogeneous Au nano-rampart arrays and its reliable SERS applications[J]. Journal of Electroanalytical Chemistry, 2022, 909: 116120.
[11] 孙伟, 洪瑞金, 陶春先, 等. 大面积图案化电场增强薄膜的设计及制备研究[J]. 中国激光, 2023, 50(23): 2303101.
[12] Yang S S, Liu G Q, Meng L P, et al. Gap-dependent SERS effect of ordered composite plasmonic nanoparticle arrays and its application for detection of sodium saccharin[J]. Optical Materials, 2021, 112: 110788.
[13] Liu Y, Zhou F, Wang H C, et al. Micro-coffee-ring-patterned fiber SERS probes and their in situ detection application in complex liquid environments[J]. Sensors and Actuators B: Chemical, 2019, 299: 126990.
[14] Gao D H, Yang X H, Teng P P, et al. Optofluidic in-fiber integrated surface-enhanced Raman spectroscopy detection based on a hollow optical fiber with a suspended core[J]. Optics Letters, 2019, 44(21): 5173-5176.
[15] Li L, Deng S X, Wang H, et al. A SERS fiber probe fabricated by layer-by-layer assembly of silver sphere nanoparticles and nanorods with a greatly enhanced sensitivity for remote sensing[J]. Nanotechnology, 2019, 30(25): 255503.
[16] Wang B T, Liu Y, Ai C W, et al. Highly sensitive SERS detection in a non-volatile liquid-phase system with nanocluster-patterned optical fiber SERS probes[J]. Optics Express, 2022, 30(10): 15846-15857.
[17] Kwak J, Lee W, Kim J B, et al. Fiber-optic plasmonic probe with nanogap-rich Au nanoislands for on-site surface-enhanced Raman spectroscopy using repeated solid-state dewetting[J]. Journal of Biomedical Optics, 2019, 24(3): 1-6.
[18] He G Y, Han X Y, Cao S Y, et al. Long spiky Au-Ag nanostar based fiber probe for surface enhanced Raman spectroscopy[J]. Materials, 2022, 15(4): 1498.
[19] Qin Y Y, Huang R D, Lu F Y, et al. Effects of the cone angle on the SERS detection sensitivity of tapered fiber probes[J]. Optics Express, 2022, 30(21): 37507-37518.
[20] Li T, Yu Z N, Wang Z K, et al. Optimized tapered fiber decorated by Ag nanoparticles for Raman measurement with high sensitivity[J]. Sensors, 2021, 21(7): 2300.
[21] Hutter T, Elliott S R, Mahajan S. Optical fibre-tip probes for SERS: numerical study for design considerations[J]. Optics Express, 2018, 26(12): 15539-15550.
[22] Cao J, Zhao D, Mao Q H. A highly reproducible and sensitive fiber SERS probe fabricated by direct synthesis of closely packed AgNPs on the silanized fiber taper[J]. Analyst, 2017, 142(4): 596-602.
[23] Yu M, Tian Q H, He G Y, et al. Surface-enhanced Raman scattering fiber probe based on silver nanocubes[J]. Advanced Fiber Materials, 2021, 3(6): 349-358.
[24] Yu F F, Su M K, Tian L, et al. Organic solvent as internal standards for quantitative and high-throughput liquid interfacial SERS analysis in complex media[J]. Analytical Chemistry, 2018, 90(8): 5232-5238.
[25] Cao J R, Huang Y L, Shang Z Y, et al. Fabrication of core shell Au@Ag supraparticles with 3D hotspots via evaporation self-assembly for sensitive surface enhanced Raman scattering detection[J]. Sensors and Actuators B: Chemical, 2023, 382: 133529.
[26] Lu G, Shrestha B, Haes A J. Importance of tilt angles of adsorbed aromatic molecules on nanoparticle rattle SERS substrates[J]. The Journal of Physical Chemistry C, 2016, 120(37): 20759-20767.
Article Outline
秦琰琰, 黄瑞冬, 刘孝兵, 钱诚, 薛驷明, 毛庆和. 锥形光纤SERS探针的批量制备和定量检测[J]. 中国激光, 2024, 51(5): 0513002. Yanyan Qin, Ruidong Huang, Xiaobing Liu, Cheng Qian, Siming Xue, Qinghe Mao. Batch Preparation and Quantitative Detection of Tapered SERS Fiber Probes[J]. Chinese Journal of Lasers, 2024, 51(5): 0513002.