InGaN基蓝光激光器p型波导层和有源区优化研究
1 引言
基于GaN的蓝色激光二极管(LD)在显示器、照明、量子技术、光学时钟、医疗仪器、材料加工、水下通信和检测等方面具有应用潜力,得到了研究人员的广泛关注[1-6]。相比具有5~10对量子阱的发光二极管(LED)[7],激光二极管通常采用1~3对量子阱[8],这是因为激光发射需要粒子数反转,而过多的量子阱就需要很高的载流子注入来激活每个量子阱,造成较高的阈值电流。但由于热化不足或捕获效率低,较少的量子阱会导致LD的载流子泄漏更加严重[9-10]。此外,在InGaN材料中存在极化效应,导致能带倾斜,这不仅在空间上分离了注入的载流子,造成量子限制斯塔克效应[11-12],而且降低了电子有效势垒高度,从而减少了对载流子的限制[13]。为了增强GaN基LD的载流子约束,不同课题组纷纷开展研究,例如:Le等[14]在电子阻挡层(EBL)之前插入一层薄薄的无掺杂InGaN层来抑制电子泄漏;Liang等[15]利用非对称多量子阱(MQW)和InxGa1-xN低波导层来提高GaN基蓝色LD的斜率效率;Hou等[16]研究了阶梯掺杂低波导层对蓝紫外LD性能的影响;Liang等[17]使用不带第一层量子垒层的多量子阱提高了电子注入比并降低了光损耗;Zhou等[18]使用阶梯式薄InGaN量子势垒显著降低了效率下降;Liu等[19]通过调整第一势垒掺杂以增强载流子约束和辐射复合。在蓝光LD中,p型波导层位于有源区和EBL之间[20],因而相比EBL,波导层或有源区的结构设计对蓝光LD的性能提升效果会更加明显。
本文在Zhong等[21]制备的蓝光LD样品的基础上,对p型波导层和有源区进行结构优化,使用模拟计算软件PICS3D分析其光电特性、能带结构、载流子电流密度分布、激射复合率等,结果表明,In组分线性递增p型波导层、前两个量子垒层In组分渐变、最后一个量子垒层使用AlGaN材料的结构可以抑制电子泄漏,增加空穴注入,提高蓝光LD的发光效率。
2 实验仿真
本文运用PICS3D软件模拟计算时所采用的蓝光LD结构示意图如
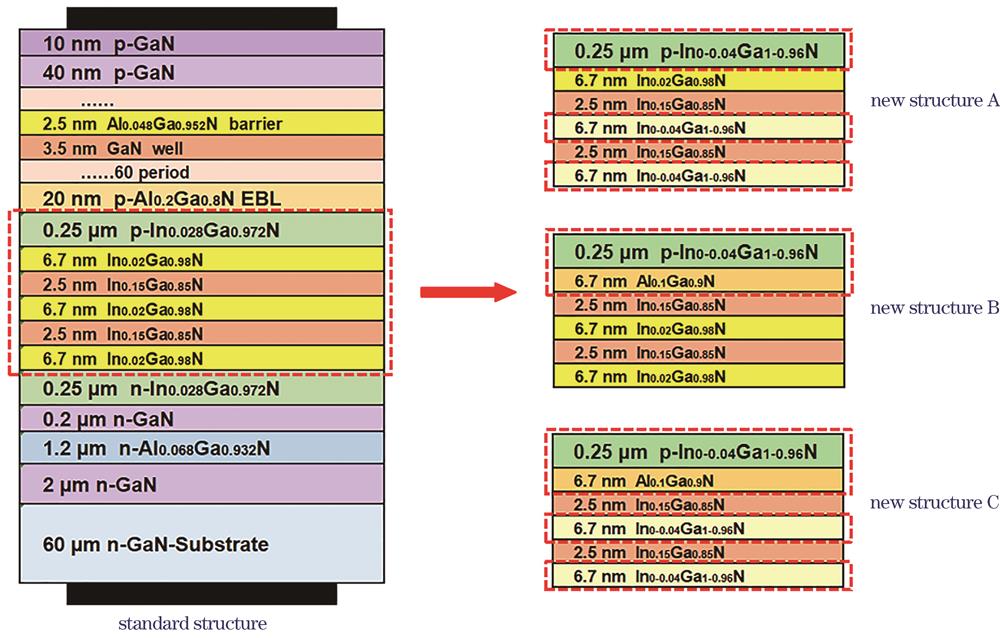
图 1. 蓝光LD标准结构与新结构示意图
Fig. 1. Schematic diagram of blue LD standard structure and new structure
模拟计算中,本文将Shockley-Read-Hall(SRH)复合寿命、俄歇复合系数分别设为1.5 ns[22]和1×10-31 cm6/s[23]。由于缺陷和自由载流子的存在,表面电荷可能被屏蔽,通常从实验测量得到的表面电荷量小于理论值[24],因而将屏蔽因子设为30%[25]。利用自洽薛定谔-泊松方程和偏振工程理论,运用包含偏移扩散和偏振模型的一维薛定谔-泊松求解器[26],可以计算LD的能带分布和载流子分布,带偏率设为0.65[27]。
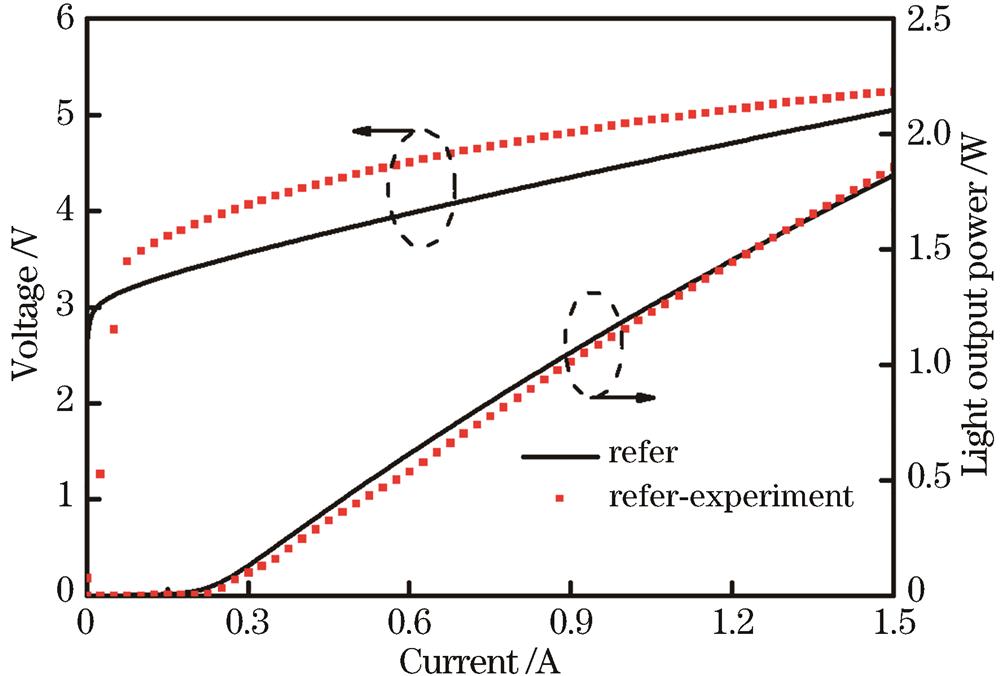
图 2. 标准结构的模拟和实验L-I-V特性曲线对比
Fig. 2. Simulated and experimental L-I-V curves of standard structures
在此基础上,提出了三种新结构,如
3 分析与讨论
由PICS3D模拟计算所得到的标准结构与三种新结构的L-I-V特性曲线图如
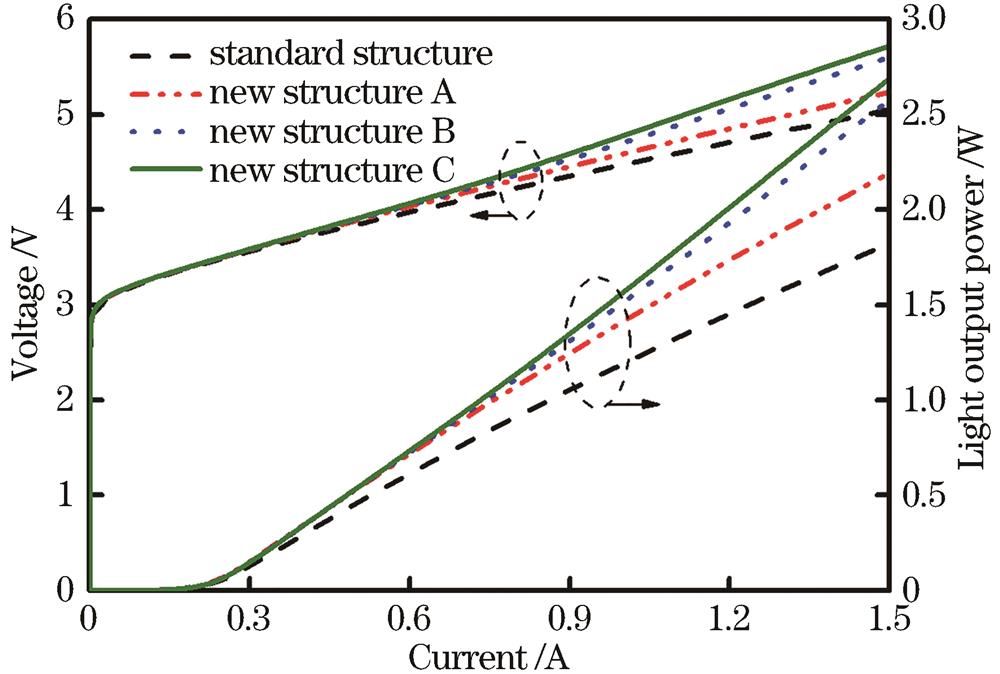
图 3. 标准结构和三种新结构的L-I-V特性曲线
Fig. 3. L-I-V characteristic curves of standard structure and three new structures
表 1. 四种结构的光电特性
Table 1. Photoelectric characteristics of four structures
|
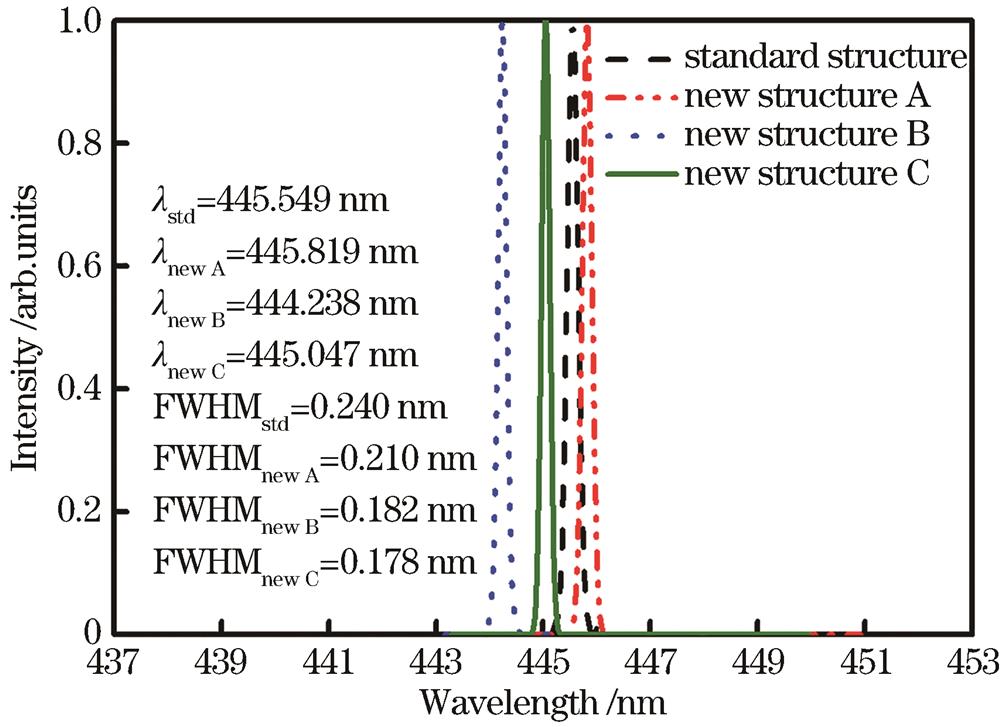
图 4. 1.5 A电流注入下标准结构和三种新结构的激射光谱图
Fig. 4. Lasing spectra of standard structure and three new structures at current of 1.5 A
为了探究新结构C性能提升的原因,本文绘制了1.5 A电流注入下标准结构和新结构C的能带结构图,如
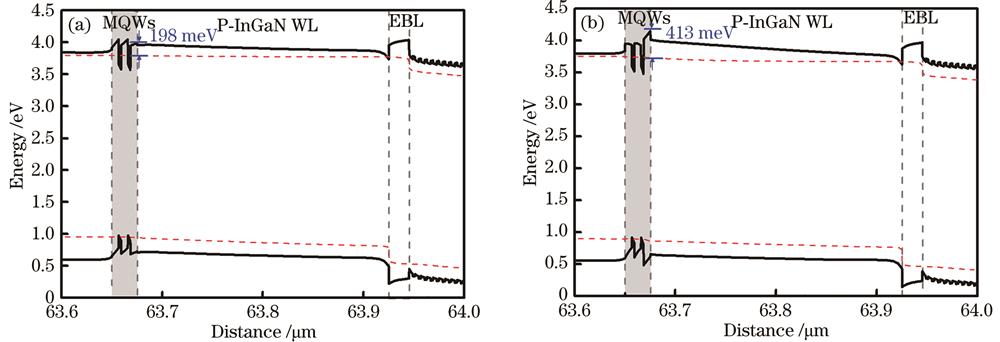
图 5. 1.5 A电流注入下的能带图。(a)标准结构;(b)新结构C
Fig. 5. Energy band diagrams at current of 1.5 A. (a) Standard structure; (b) new structure C
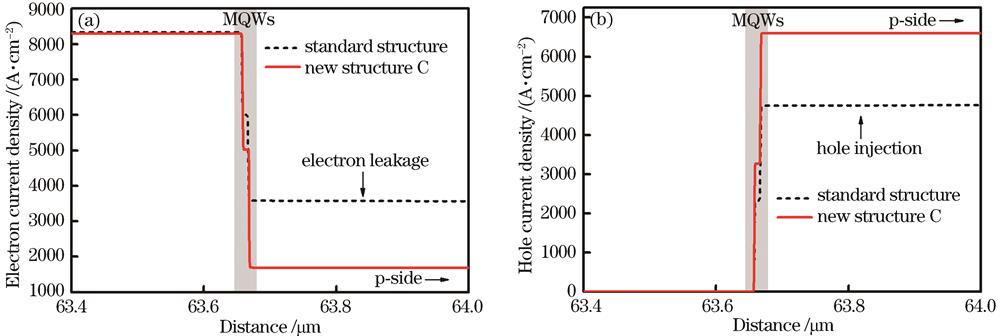
图 6. 1.5 A电流注入下标准结构和新结构C的载流子电流密度。(a)电子电流密度;(b)空穴电流密度
Fig. 6. Carrier current density of standard structure and new structure C at current of 1.5 A. (a) Electron current density; (b) hole current density
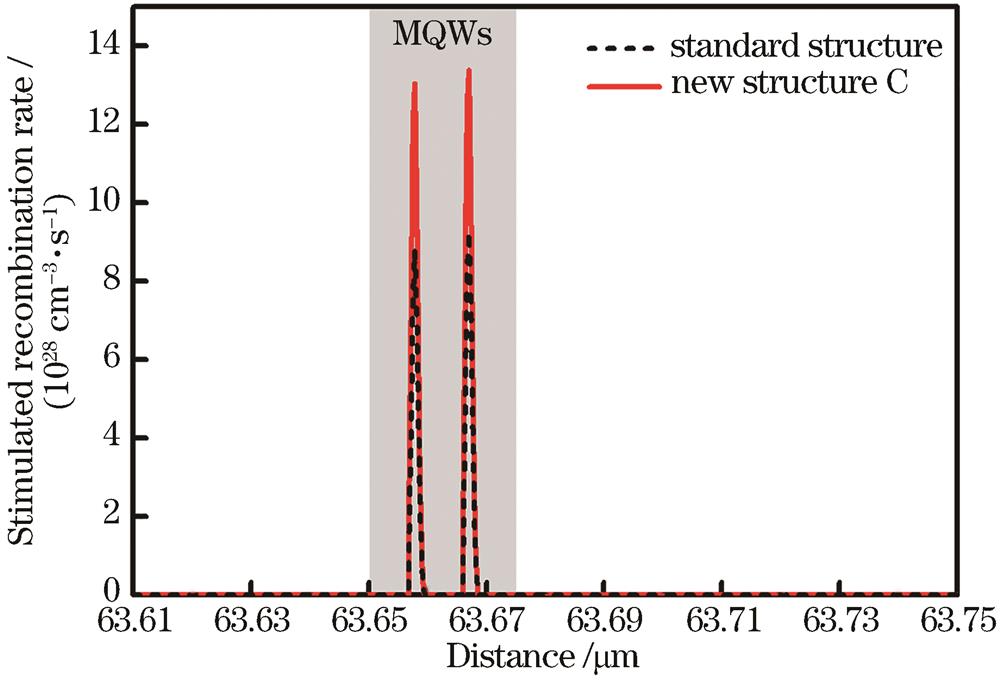
图 7. 1.5 A电流注入下标准结构和新结构C的受激辐射复合率
Fig. 7. Stimulated recombination rate of standard structure and new structure C at current of 1.5 A
4 结论
本文在实验样品结构基础上,提出了In组分线性递增p型波导层以及前两个量子垒层In组分渐变、最后一个量子垒层使用AlGaN材料的综合设计。模拟结果表明,新结构可以很好地抑制电子泄漏,增加空穴注入,提高量子阱内的受激辐射率,从而提高蓝光激光器的发光效率,在1.5 A注入电流下,新结构的光输出功率可达2.69 W,相较标准结构提升了47.8 %。本文的模拟结果对后续的实验具有一定的指导意义。
[1] Tian A Q, Hu L, Zhang L Q, et al. Design and growth of GaN-based blue and green laser diodes[J]. Science China Materials, 2020, 63(8): 1348-1363.
[2] Murayama M, Nakayama Y, Yamazaki K, et al. Watt-class green (530 nm) and blue (465 nm) laser diodes[J]. Physica Status Solidi (a), 2018, 215(10): 1700513.
[3] Monavarian M, Rashidi A, Feezell D. A decade of nonpolar and semipolar III-nitrides: a review of successes and challenges[J]. Physica Status Solidi (a), 2018, 216(1): 1800628.
[4] 李晋闽, 刘志强, 魏同波, 等. 中国半导体照明发展综述[J]. 光学学报, 2021, 41(1): 0116002.
[5] Kothuru A, Goel S. Leveraging 3-D printer with 2.8-W blue laser diode to form laser-induced graphene for microfluidic fuel cell and electrochemical sensor[J]. IEEE Transactions on Electron Devices, 2022, 69(3): 1333-1340.
[6] 孙天宇, 夏明俊, 乔雷. 半导体激光器失效机理与检测分析研究进展[J]. 激光与光电子学进展, 2021, 58(19): 1900003.
[7] Jia C Y, Yu T J, Lu H M, et al. Performance improvement of GaN-based LEDs with step stage InGaN/GaN strain relief layers in GaN-based blue LEDs[J]. Optics Express, 2013, 21(7): 8444-8449.
[8] Liu J X, Wang J, Sun X J, et al. Performance improvement of InGaN-based laser grown on Si by suppressing point defects[J]. Optics Express, 2019, 27(18): 25943-25952.
[9] Grupen M, Hess K. Simulation of carrier transport and nonlinearities in quantum-well laser diodes[J]. IEEE Journal of Quantum Electronics, 1998, 34(1): 120-140.
[10] 宁永强, 陈泳屹, 张俊, 等. 大功率半导体激光器发展及相关技术概述[J]. 光学学报, 2021, 41(1): 0114001.
[11] Tsai Y C, Bayram C, Leburton J P. Effect of auger electron-hole asymmetry on the efficiency droop in InGaN quantum well light-emitting diodes[J]. IEEE Journal of Quantum Electronics, 2022, 58(1): 3300109.
[12] Roy S, Ahsan S M T, Mondol N, et al. Comparative investigation into key optoelectronic characteristics of semipolar InGaN blue laser diodes: a strategy to mitigate quantum-confine stark effect[J]. Results in Physics, 2022, 34: 105246.
[13] Ikeda M, Zhang F, Zhou R L, et al. Thermionic emission of carriers in InGaN/(In)GaN multiple quantum wells[J]. Japanese Journal of Applied Physics, 2019, 58(SC): SCCB03.
[14] Le L C, Zhao D G, Jiang D S, et al. Suppression of electron leakage by inserting a thin undoped InGaN layer prior to electron blocking layer in InGaN-based blue-violet laser diodes[J]. Optics Express, 2014, 22(10): 11392-11398.
[15] Liang F, Zhao D G, Jiang D S, et al. Improvement of slope efficiency of GaN-Based blue laser diodes by using asymmetric MQW and InxGa1-xN lower waveguide[J]. Journal of Alloys and Compounds, 2018, 731: 243-247.
[16] Hou Y F, Zhao D G, Liang F, et al. Performance improvement of GaN-based blue and ultraviolet double quantum well laser diodes by using stepped-doped lower waveguide[J]. Materials Science in Semiconductor Processing, 2021, 121: 105355.
[17] Liang F, Zhao D G, Liu Z S, et al. Improved performance of GaN-based blue laser diodes using asymmetric multiple quantum wells without the first quantum barrier layer[J]. Optics Express, 2022, 30(6): 9913-9923.
[18] Zhou K, Ikeda M, Liu J P, et al. Remarkably reduced efficiency droop by using staircase thin InGaN quantum barriers in InGaN based blue light emitting diodes[J]. Applied Physics Letters, 2014, 105(17): 173510.
[19] Liu J X, Qie H R, Sun Q, et al. Enhanced carrier confinement and radiative recombination in GaN-based lasers by tailoring first-barrier doping[J]. Optics Express, 2020, 28(21): 32124-32131.
[20] Liang F, Zhao D G, Liu Z S, et al. GaN-based blue laser diode with 6.0 W of output power under continuous-wave operation at room temperature[J]. Journal of Semiconductors, 2021, 42(11): 70-72.
[21] Zhong Z B, Lu S Q, Li J C, et al. Design and fabrication of high power InGaN blue laser diode over 8 W[J]. Optics & Laser Technology, 2021, 139: 106985.
[22] Goudon T, Miljanović V, Schmeiser C. On the Shockley-read-hall model: generation-recombination in semiconductors[J]. SIAM Journal on Applied Mathematics, 2007, 67(4): 1183-1201.
[23] Brendel M, Kruse A, Jönen H, et al. Auger recombination in GaInN/GaN quantum well laser structures[J]. Applied Physics Letters, 2011, 99(3): 031106.
[24] Fiorentini V, Bernardini F, Ambacher O. Evidence for nonlinear macroscopic polarization in III-V nitride alloy heterostructures[J]. Applied Physics Letters, 2002, 80(7): 1204-1206.
[25] Li X, Zhao D G, Jiang D S, et al. The effectiveness of electron blocking layer in InGaN-based laser diodes with different indium content[J]. Physica Status Solidi (a), 2016, 213(8): 2223-2228.
[26] Li C K, Piccardo M, Lu L S, et al. Localization landscape theory of disorder in semiconductors. III. Application to carrier transport and recombination in light emitting diodes[J]. Physical Review B, 2017, 95(14): 144206.
[27] Vurgaftman I, Meyer J R, Ram-Mohan L R. Band parameters for III-V compound semiconductors and their alloys[J]. Journal of Applied Physics, 2001, 89(11): 5815-5875.
[28] Moustakas T D, Paiella R. Optoelectronic device physics and technology of nitride semiconductors from the UV to the terahertz[J]. Reports on Progress in Physics, 2017, 80(10): 106501.
石澜, 李书平. InGaN基蓝光激光器p型波导层和有源区优化研究[J]. 激光与光电子学进展, 2023, 60(17): 1714007. Lan Shi, Shuping Li. Research on Optimization of p-Type Waveguide Layer and Active Region of InGaN-Based Blue Laser Diodes[J]. Laser & Optoelectronics Progress, 2023, 60(17): 1714007.