平行板约束对激光诱导PMMA等离子体中CN分子光谱的影响
下载: 617次
1 引言
激光诱导击穿光谱(LIBS),又称为激光诱导等离子体光谱(LIPS)或激光火花光谱(LSS),作为原子发射光谱(AES),因具有操作简便、成本低廉、检测范围广、多组分元素能同时检测等优点,在物质组分分析方面有着独特的优势[1-3]。LIBS的基本原理相对简单,即一束具有高能量密度的激光脉冲照射样品,在激光照射样品区的上方形成高温、高密度等离子体,产生等离子体辐射的光经透镜会聚到光谱探测器中,再通过探测器得到光谱波长和强度信息。LIBS技术已经被应用到环境监测、空间探测、生物医学及一些**应用等领域[4-9]。当前,提高LIBS技术的探测灵敏度是人们关注的热点之一,很多实验技术已经被用来提高LIBS信号的强度,代表性的方法有双脉冲LIBS[10-11]、火花放电辅助LIBS[12-13]、预加热LIBS[14-15]、磁约束LIBS[16-19]及空间约束LIBS[20-25]等。
在上述增强光谱技术中,空间约束LIBS凭借操作简单、效率高、成本低等优点被广泛研究。空间约束LIBS加强效应的主要机理是反射冲击波与等离子体的相互作用[26]。在采用空间约束LIBS的实验中,当激光脉冲会聚到样品表面时,在样品表面产生等离子体,同时伴随冲击波的产生,冲击波的传播速度高于等离子体羽膨胀的速度;当冲击波在其膨胀过程中遇到约束腔的内壁时,冲击波会被反弹并开始反向传播,随后将和膨胀的等离子体羽相遇,能将等离子体羽压缩至较小尺寸[27],使得粒子间的碰撞几率增加,从而产生更多的高激发态粒子,这些粒子跃迁至低的能级时能使辐射更强。Gao等[20]研究了空间约束下纳秒激光诱导Cu等离子体,在无空间约束情况下,冲击波以半球形不断向外膨胀,而在空间约束的情况下,冲击波被反弹并将等离子体羽压缩,使等离子体辐射出更强的光。Wang等[22]讨论了不同形状约束腔对LIBS的影响,结果表明,空间约束可以提高等离子体的光谱强度和电子温度,且在圆柱形约束腔下,等离子体的光谱强度和电子温度最高。Shen等[23]研究了圆柱形约束腔下激光诱导Al等离子体光谱,在空间约束下,波长为394.40 nm和396.15 nm处的Al(I)的谱线强度显著增强,增强因子约为7。Wang等[28]研究了空间约束下激光诱导Cu等离子体中Cu(I)光谱的持续时间,结果发现,空间约束可以在一定延迟时间范围内增强光谱强度,且这个延迟时间的范围取决于激光的能量和约束腔的直径。
尽管许多国内外学者在这个领域都进行了大量的工作,但这些工作主要针对LIBS中的原子和离子光谱,而关于空间约束下激光诱导等离子体中的分子光谱的研究相对较少。在LIBS中,分子光谱能提供样品中非常有价值的化学信息,可以用来分析爆炸物、细菌、生物材料、卤素元素、同位素及各种有机化合物等[29-32],所以有必要研究空间约束对激光诱导等离子体中分子光谱的影响。另外,聚甲基丙烯酸甲酯(PMMA),又称亚克力,是最为常见的聚合物材料。PMMA价格低、韧性强、易于机械加工,具有良好的光学和绝缘特性。同时,PMMA表面光滑平整,在聚焦激光脉冲实验的过程中,平整的表面有利于光谱信号的稳定。因此,在本实验中采用PMMA作为样品,研究了空间约束对激光诱导等离子体中分子光谱的影响,测量了CN分子的时间分辨光谱,并利用CN分子光谱计算了CN分子的振动温度。
2 实验装置
空间约束下激光诱导PMMA等离子体的实验装置如
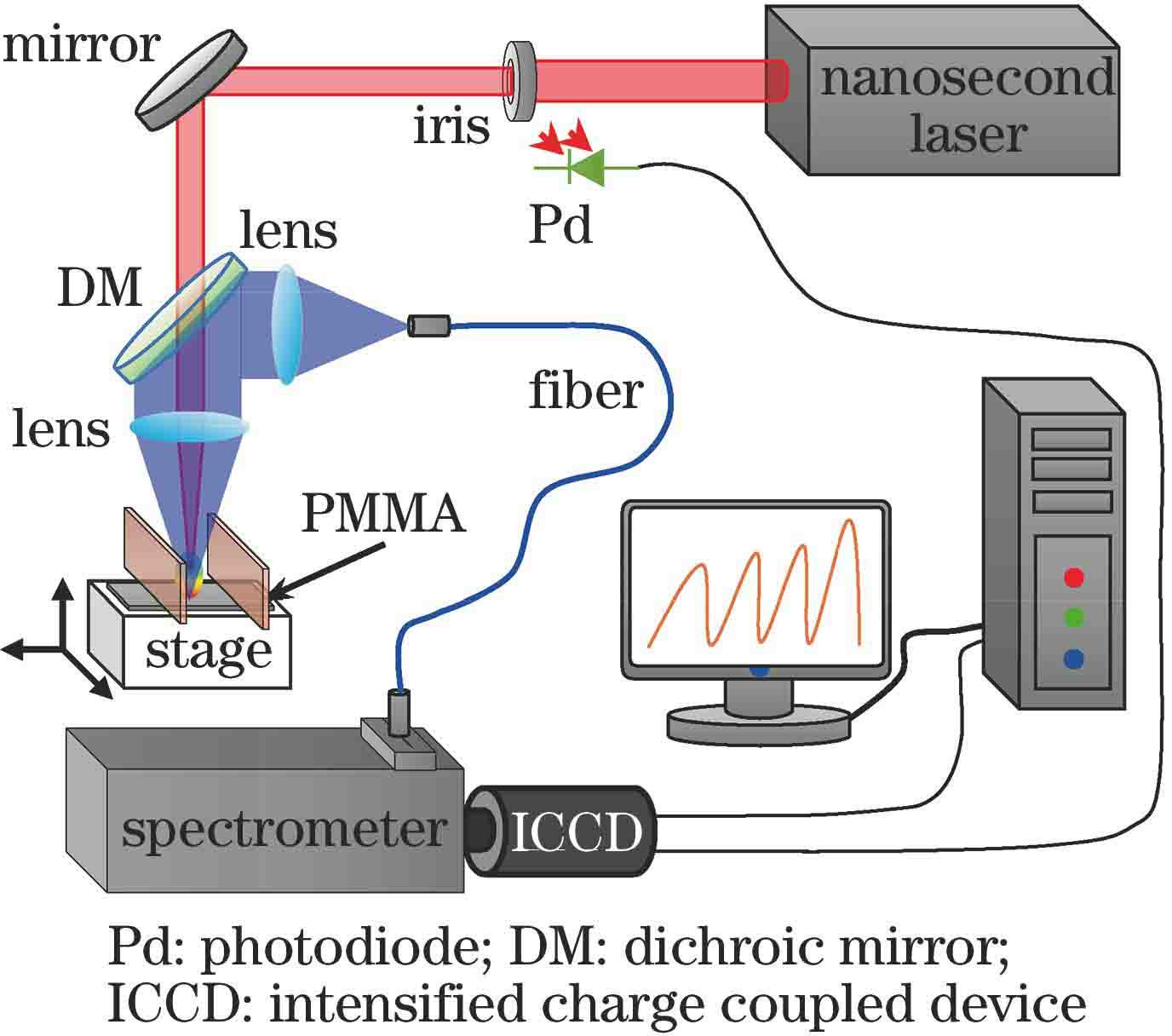
图 1. 纳秒激光诱导PMMA等离子体光谱实验装置示意图
Fig. 1. Schematic of experimental setup for nanosecond laser-induced PMMA plasma spectroscopy
3 结果与讨论
LIBS的变化过程主要分为三个阶段:待测样品被脉冲激光激发;产生激光诱导的等离子体;等离子体的快速膨胀和冷却[33-34]。其中,最有趣的过程就是等离子体的动态衰减过程,这个过程中的等离子体光谱随着延迟时间不断变化[35]。为了了解等离子体光谱的时间演化,测量了在有、无空间约束下的时间分辨光谱。无、有平行板约束下CN分子的时间分辨光谱如
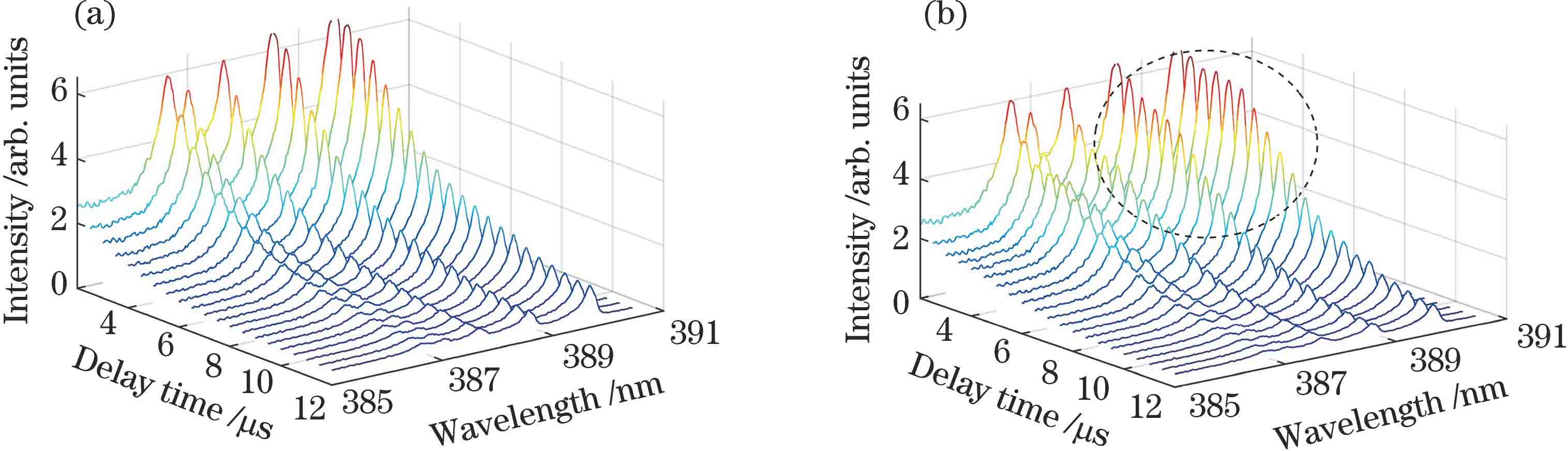
图 2. CN分子(Δν=0)的时间分辨光谱。(a)无空间约束腔;(b)有空间约束腔
Fig. 2. Time-resolved spectra of CN molecule (Δν=0). (a) Without spatial confinement cavity; (b) with spatial confinement cavity
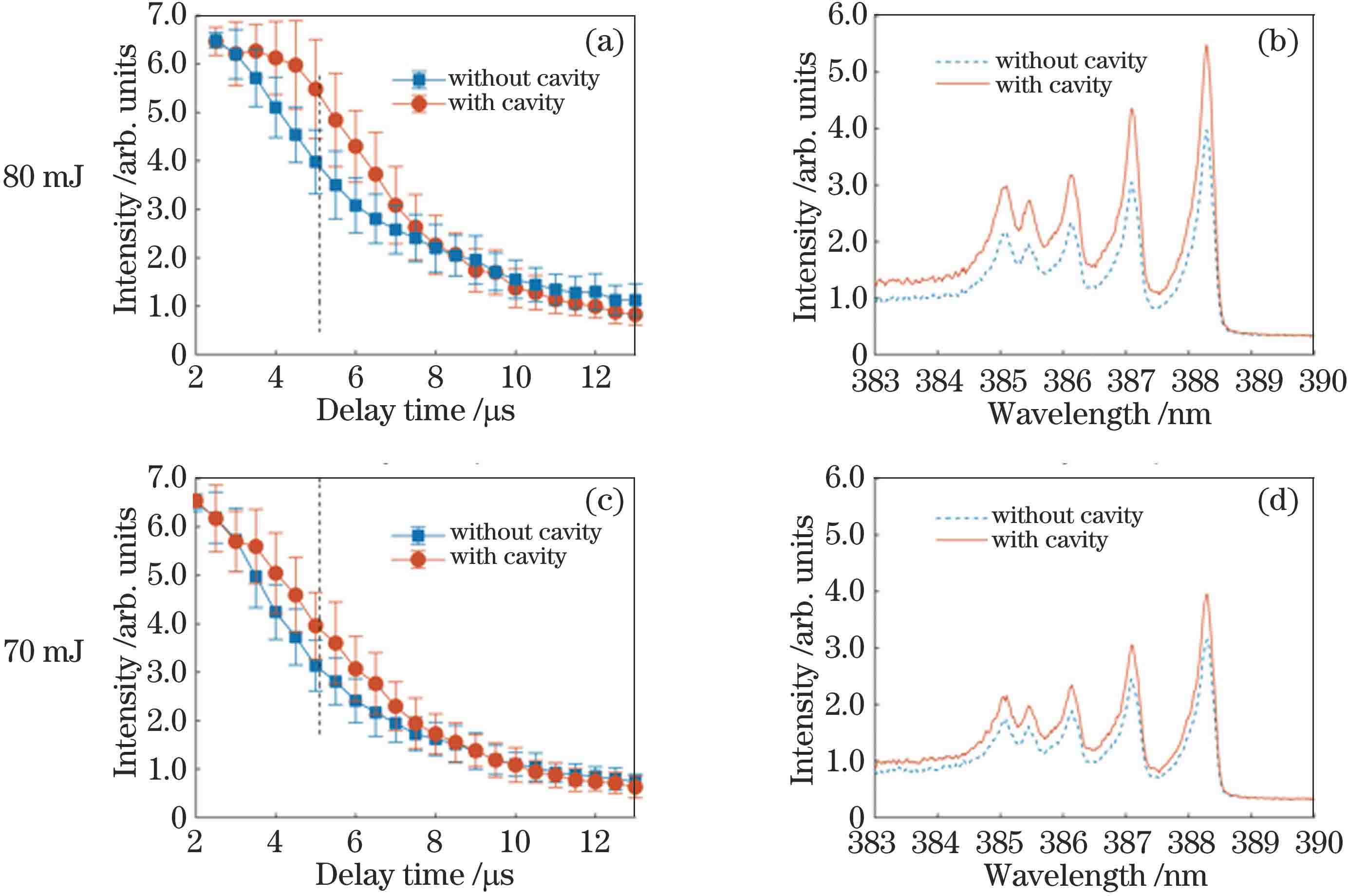
图 3. CN(0-0)光谱峰强度随延迟时间和波长的变化。(a)延迟时间;(b)波长
Fig. 3. Evolution of CN (0-0) spectral peak intensity with delay time and wavelength. (a) Delay time; (b) wavelength
光谱的信号强度是一个重要的指标,增加激光能量能直接地增强光谱的强度。
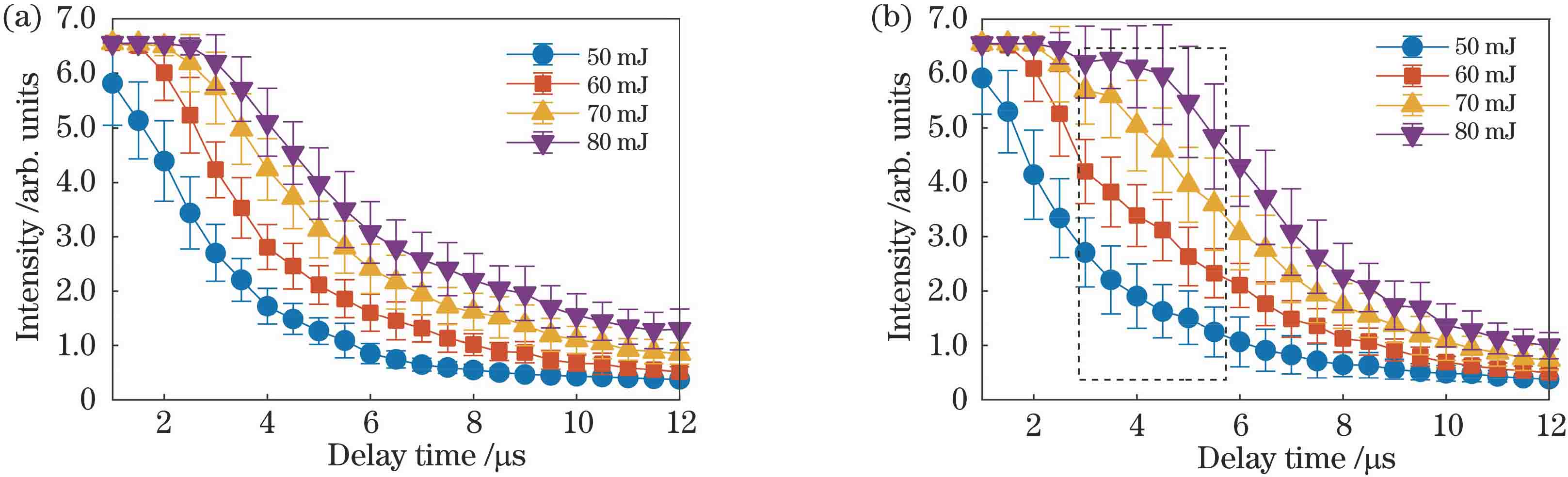
图 4. CN(0-0)光谱峰强度随延迟时间的变化。(a)无空间约束腔;(b)有空间约束腔
Fig. 4. Evolution of CN(0-0) spectral peak intensity with delay time. (a) Without spatial confinement cavity; (b) with spatial confinement cavity
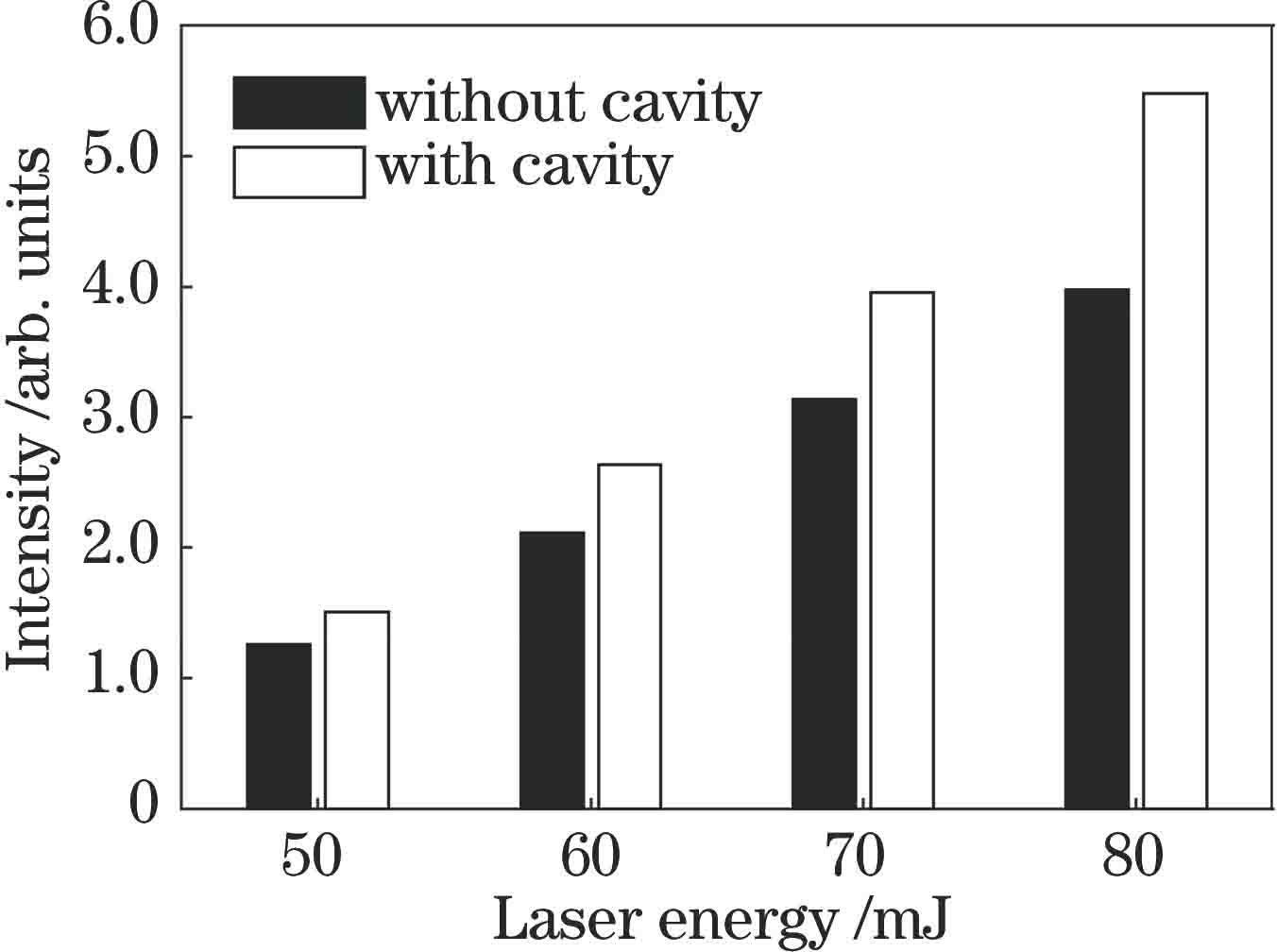
图 5. 延迟时间为5 μs时CN(0-0)光谱峰强度随激光能量的变化
Fig. 5. Evolution of CN(0-0) spectral peak intensity with laser energy at delay time of 5 μs
温度信息是等离子体重要的参数之一,通过温度信息,能更有助于理解空间约束下CN分子光谱的增强效应。
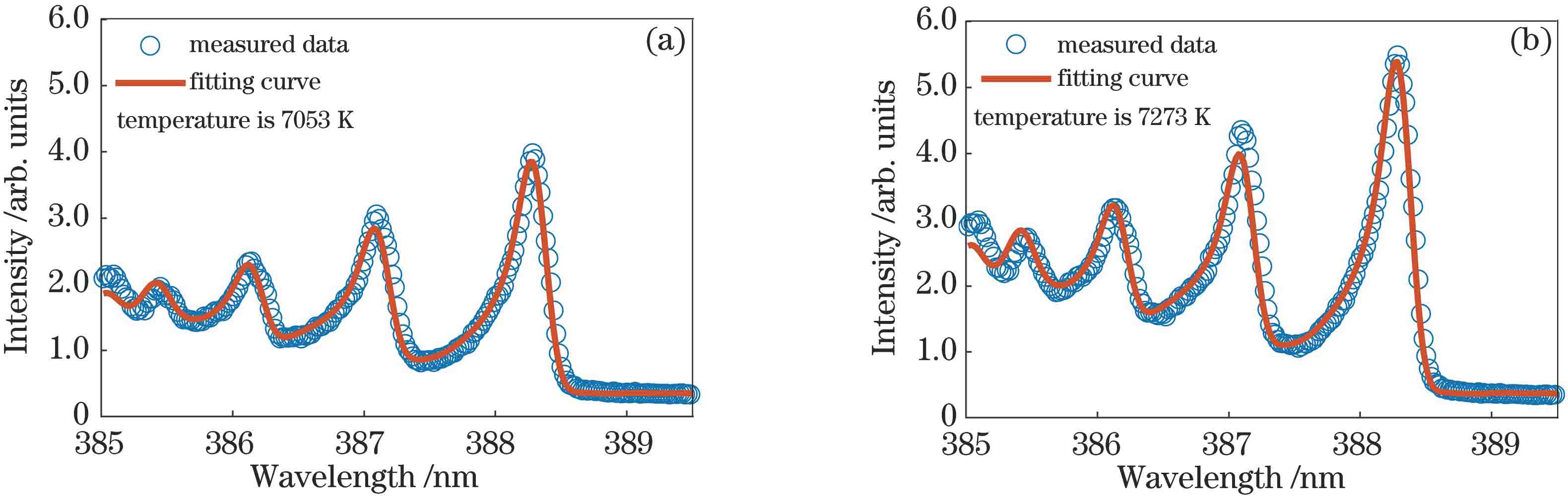
图 6. CN(Δν=0)光谱对比。(a)无空间约束腔;(b)有空间约束腔
Fig. 6. Comparison of CN(Δν=0) spectra. (a) Without spatial confinement cavity; (b) with spatial confinement cavity
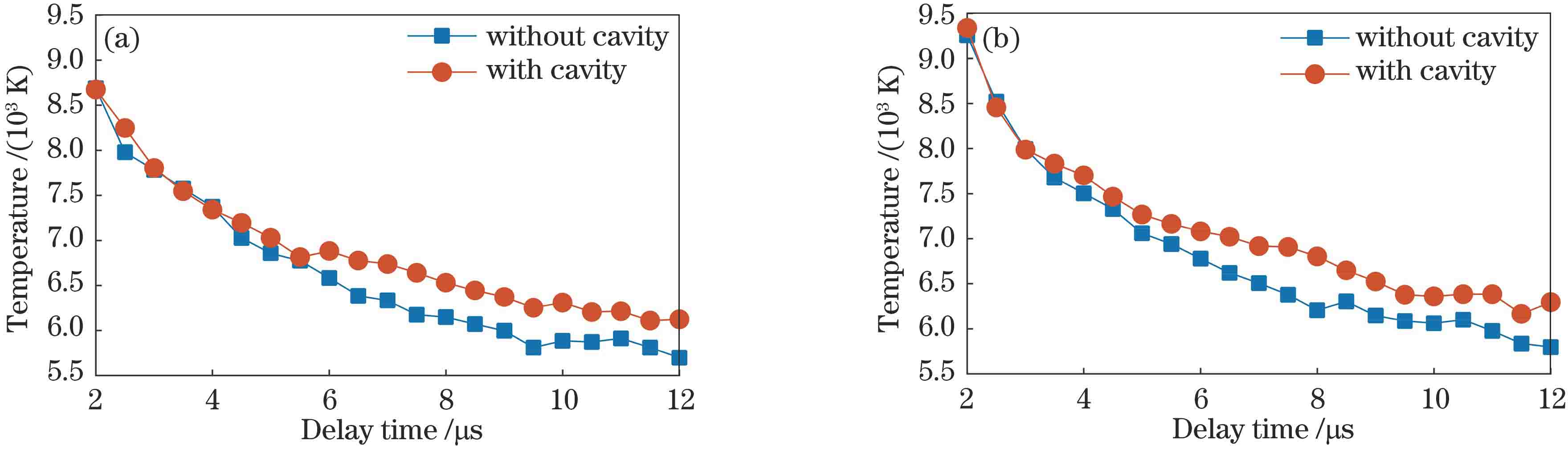
图 7. 不同激光能量下,CN分子的振动温度随延迟时间的变化。(a) 70 mJ;(b) 80 mJ
Fig. 7. Evolution of vibration temperatureof CN molecule with delay time under different laser energies. (a) 70 mJ; (b) 80 mJ
4 结论
研究平行板约束下的激光诱导PMMA等离子体,测量PMMA等离子体中CN(Δν=0)的时间分辨光谱。当平行板被用于约束PMMA等离子体时,CN(Δν=0)光谱峰强度得到明显增加,并且,激光能量越强,CN(Δν=0)光谱峰强度增加得越明显。另外,通过拟合CN(Δν=0)光谱,获得了CN分子的振动温度,发现采用空间约束的振动温度高于无空间约束下振动温度的情况。PMMA等离子体中CN光谱的增强是基于冲击波与等离子体羽的相互作用的,平行板反射冲击波,等离子体羽得到压缩,等离子体羽中的各种粒子数密度增加,进而增加了粒子之间的碰撞几率和等离子体温度,导致PMMA等离子体中CN分子辐射出幅值更大的光谱。
[1] Wang Z, Dong F Z, Zhou W D. A rising force for the world-wide development of laser-induced breakdown spectroscopy[J]. Plasma Science and Technology, 2015, 17(8): 617-620.
[2] Wang Z Z, Deguchi Y, Zhang Z Z, et al. Laser-induced breakdown spectroscopy in Asia[J]. Frontiers of Physics, 2016, 11(6): 114213.
[3] 杨雪, 张丹, 陈安民, 等. 聚焦透镜到样品表面的距离对激光诱导硅等离子体原子谱线强度和离子谱线强度的影响[J]. 中国激光, 2019, 46(11): 1111001.
[4] Knight A K, Scherbarth N L, Cremers D A, et al. Characterization of laser-induced breakdown spectroscopy (LIBS) for application to space exploration[J]. Applied Spectroscopy, 2000, 54(3): 331-340.
[5] 陈安民, 何喜明, 费德厚, 等. 飞秒激光加热双层金属薄膜的理论研究[J]. 激光与光电子学进展, 2017, 54(5): 015402.
Chen A M, He X M, Fei D H, et al. Theoretical study on femtosecond laser heating of two-layer metal films[J]. Laser & Optoelectronics Progress, 2017, 54(5): 015402.
[6] Harilal S S, Brumfield B E. LaHaye N L, et al. Optical spectroscopy of laser-produced plasmas for standoff isotopic analysis[J]. Applied Physics Reviews, 2018, 5(2): 021301.
[7] 杨文斌, 李斌成, 韩艳玲, 等. 激光诱导击穿光谱技术定量分析氩气和氮气中的痕量氧含量[J]. 中国激光, 2017, 44(10): 1011001.
[8] 蔡志龙, 杨秋松, 王阳. 铜铝合金溅射薄膜的飞秒激光诱导击穿光谱分析[J]. 中国激光, 2015, 42(6): 0615001.
[9] 王金梅, 颜海英, 郑培超, 等. 基于激光诱导击穿光谱定量检测土壤中营养元素的研究[J]. 中国激光, 2017, 44(11): 1111002.
[10] 高勋, 邵妍, 杜闯, 等. 预烧蚀激光参数对双脉冲激光诱导击穿光谱增强的影响[J]. 中国激光, 2013, 40(8): 0815003.
[11] Wang Y, Chen A M, Wang Q Y, et al. Enhancement of optical emission generated from femtosecond double-pulse laser-induced glass plasma at different sample temperatures in air[J]. Plasma Science and Technology, 2019, 21(3): 034013.
[12] Wang Q Y, Chen A M, Xu W P, et al. Time-resolved spectroscopy of femtosecond laser-induced Cu plasma with spark discharge[J]. Plasma Science and Technology, 2019, 21(6): 065504.
[13] Wang Y R, Jiang Y H, He X Y, et al. Triggered parallel discharge in laser-ablation spark-induced breakdown spectroscopy and studies on its analytical performance for aluminum and brass samples[J]. Spectrochimica Acta Part B: Atomic Spectroscopy, 2018, 150: 9-17.
[14] Tavassoli S H, Gragossian A. Effect of sample temperature on laser-induced breakdown spectroscopy[J]. Optics & Laser Technology, 2009, 41(4): 481-485.
[15] 齐洪霞, 赵亮, 金川琳, 等. 样品温度对纳秒激光诱导铝等离子体光谱强度的影响[J]. 中国激光, 2019, 46(2): 0211002.
[16] Harilal S S. O'Shay B, Tillack M S, et al. Fast photography of a laser generated plasma expanding across a transverse magnetic field[J]. IEEE Transactions on Plasma Science, 2005, 33(2): 474-475.
[17] Lu Y, Zhou Y S, Qiu W, et al. Magnetic field enhancement for femtosecond-laser-ablation mass spectrometry in ambient environments[J]. Journal of Analytical Atomic Spectrometry, 2015, 30(11): 2303-2306.
[18] Pandey P K, Thareja R K. Rotating copper plasmoid in external magnetic field[J]. Physics of Plasmas, 2013, 20(2): 022117.
[19] Singh K S, Sharma A K. Spatially resolved behavior of laser-produced copper plasma along expansion direction in the presence of static uniform magnetic field[J]. Physics of Plasmas, 2016, 23(12): 122104.
[20] Gao X, Liu L, Song C, et al. The role of spatial confinement on nanosecond YAG laser-induced Cu plasma[J]. Journal of Physics D: Applied Physics, 2015, 48(17): 175205.
[21] Shen X K, Sun J, Ling H, et al. Spatial confinement effects in laser-induced breakdown spectroscopy[J]. Applied Physics Letters, 2007, 91(8): 081501.
[22] Wang Q Y, Chen A M, Zhang D, et al. The role of cavity shape on spatially confined laser-induced breakdown spectroscopy[J]. Physics of Plasmas, 2018, 25(7): 073301.
[23] Shen X K, Sun J, Ling H, et al. Spectroscopic study of laser-induced Al plasmas with cylindrical confinement[J]. Journal of Applied Physics, 2007, 102(9): 093301.
[24] Guo L B, Hu W, Zhang B Y, et al. Enhancement of optical emission from laser-induced plasmas by combined spatial and magnetic confinement[J]. Optics Express, 2011, 19(15): 14067-14075.
[25] Wang Y, Chen A M, Sui L Z, et al. Two sequential enhancements of laser-induced Cu plasma with cylindrical cavity confinement[J]. Journal of Analytical Atomic Spectrometry, 2016, 31(10): 1974-1977.
[26] Li X W, Yang Z F, Wu J, et al. Spatial confinement in laser-induced breakdown spectroscopy[J]. Journal of Physics D: Applied Physics, 2017, 50(1): 015203.
[27] Fu Y T, Hou Z Y, Wang Z. Physical insights of cavity confinement enhancing effect in laser-induced breakdown spectroscopy[J]. Optics Express, 2016, 24(3): 3055-3066.
[28] Wang Y, Chen A M, Sui L Z, et al. Persistence of atomic spectral line on laser-induced Cu plasma with spatial confinement[J]. Physics of Plasmas, 2016, 23(11): 113105.
[29] Trautner S, Jasik J, Parigger C G, et al. Laser-induced optical breakdown spectroscopy of polymer materials based on evaluation of molecular emission bands[J]. Spectrochimica Acta Part A: Molecular and Biomolecular Spectroscopy, 2017, 174: 331-338.
[30] Hou H M, Mao X L, Zorba V, et al. Laser ablation molecular isotopic spectrometry for molecules formation chemistry in femtosecond-laser ablated plasmas[J]. Analytical Chemistry, 2017, 89(14): 7750-7757.
[31] Mousavi S J. HematiFarsani M, Darbani S M R, et al. CN and C2 vibrational spectra analysis in molecular LIBS of organic materials[J]. Applied Physics B, 2016, 122(5): 106.
[32] Rai S, Rai A K. Characterization of organic materials by LIBS for exploration of correlation between molecular and elemental LIBS signals[J]. AIP Advances, 2011, 1(4): 042103.
[33] LaHaye N L, Harilal S S, Diwakar P K, et al. Persistence of uranium emission in laser-produced plasmas[J]. Journal of Applied Physics, 2014, 115(16): 163301-163308.
[34] Hussein A E, Diwakar P K, Harilal S S, et al. The role of laser wavelength on plasma generation and expansion of ablation plumes in air[J]. Journal of Applied Physics, 2013, 113(14): 143305.
[35] Guo K M, Chen A M, Xu W P, et al. Effect of sample temperature on time-resolved laser-induced breakdown spectroscopy[J]. AIP Advances, 2019, 9(6): 065214.
[36] Guo L B, Li C M, Hu W, et al. Plasma confinement by hemispherical cavity in laser-induced breakdown spectroscopy[J]. Applied Physics Letters, 2011, 98(13): 131501.
[37] Wang J M, Zheng P C, Liu H D, et al. Spectral characteristics of laser-induced graphite plasma in ambient air[J]. Plasma Science and Technology, 2016, 18(11): 1123-1129.
[38] Wang Q Y, Chen A M, Qi H X, et al. Influence of distance between sample surface and geometrical focal point on CN emission intensity from femtosecond laser-induced PMMA plasmas[J]. Physics of Plasmas, 2019, 26(7): 073302.
杨雪, 陈安民, 李苏宇, 姜远飞, 金明星. 平行板约束对激光诱导PMMA等离子体中CN分子光谱的影响[J]. 中国激光, 2020, 47(8): 0811002. Yang Xue, Chen Anmin, Li Suyu, Jiang Yuanfei, Jin Mingxing. Effect of Parallel Plate Constraint on CN Molecular Spectra in Laser-Induced PMMA Plasma[J]. Chinese Journal of Lasers, 2020, 47(8): 0811002.