空间整形飞秒激光图案化加工氧化石墨烯
下载: 1743次特邀研究论文
Objective Graphene oxide (GO) is a graphene derivative with oxygen-containing functional group in its graphite structure. It can be reduced by heat, chemical reaction, and laser-induced reduction methods. Laser irradiation can induce reduction of GO in the irradiation area with good flexibility and area selectivity, and no special environment is required. In this paper, we propose a patterning method to process GO using a spatially-shaped femtosecond laser. We use the spatially-shaped laser irradiating method and a Gaussian laser scanning method to fabricate patterns on GO, and we analyze the effects of irradiation time, laser fluence, etc. The characteristic results demonstrate that the spatially-shaped femtosecond laser irradiating method realizes reductive patterning on GO, and the efficiency of patterning is improved. The proposed method also demonstrates good repeatability and flexibility in patterning. It has application potential in fabricating GO-based microcircuits and microdevices.
Methods GO films were prepared by filtering GO dispersion with a cellulose filter membrane and drying. The femtosecond laser has an 800-nm central wavelength, 35-fs pulse duration, and 1-kHz repetition rate. Different holograms were loaded on a spatial light modulator (SLM) to obtain the corresponding optical field at the focus. The Gerchberg-Saxon algorithm was used to calculate the phase distribution (i.e., hologram) according to the targeted complex amplitude distribution. When the specific optical field shape at the back focal plane of lens was obtained, the 4f optical system was used to guarantee that laser propagates without distortion and focuses on the surface of GO by the 5× objective lens. To realize unshaped laser scanning and compare fabrication results of shaped laser irradiating, a plane phase hologram was loaded on the SLM. Under this condition, an unshaped Gaussian optical field was formed at the focus. After reductive patterning, a scanning electron microscope (SEM) was used to characterize the micromorphology of the patterned areas to confirm the patterning effect of the two fabrication procedures. The height distribution and uniformity of the patterns were observed using a white light interferometer (WLI). Raman spectroscopy was used to obtain the Raman spectra of the patterned areas, and the characteristic peaks in the Raman spectra demonstrated whether the patterned area was reduced by the femtosecond laser.
Results and Discussions The two methods were used to fabricate the same four 40mm×40mm square patterns on GO. Sketch maps of the two methods and micromorphologies characterized by SEM and WLI of the squares are showed (Fig. 2). For the proposed irradiating method, the intensity of the light field in the entire irradiating area was not uniform, and we regarded it as uniform when calculating fluence. The results demonstrate that the patterns are divided into many out-of-shape areas by gully-like structures. Areas surrounded by gullies showed hump-like structures (~5μm scale). Some lamellar structures were observed on these humps. These results were generated by the nonuniform intensity of the optical field, gullies were fabricated by a light field with higher intensity, and humps were fabricated by a weaker light field. For the micromorphology of the laser scanning method, humps and gullies were also observed in the areas. However, the generated humps were smaller (~1μm scale). Floccule structures were also observed in the entire area. These floccule structures were generated because gas was released from GO in a short period during laser scanning, which made the GO porous. Regarding fabrication efficiency, the irradiating procedure was performed in 500 ms; however, the scanning procedure required 640 s. We studied the influences of laser fluence and irradiating time on shaped laser irradiating (Fig. 3). Patterns irradiated with the same irradiating time and different fluence are distinguished on the depth and width of gullies. The D-band intensity of the Raman spectra indicates distortion of the graphic structure of GO, and 2D-band of the Raman spectra represents production of the graphene-like sp 2 structure. For unshaped laser scanning, we also adjusted some parameters to obtain diverse micromorphologies and reduction results (Fig. 4). Based on the Raman spectrum results, the maximum reduction in the two series of experiments was similar; however, the time required to perform each method differed significantly. Processing efficiency was improved significantly using shaped laser irradiating when we fabricated the same pattern and obtained the nearly reduction effect. Finally, we applied the proposed spatially-shaped laser irradiating method to fabricate a “THU” shaped pattern (Fig. 5). For this relatively complicated pattern, it is difficult to fabricate it once via irradiation using the shaped laser because the calculation of the hologram would differ. The tailoring and splicing method can simplify this process. In this example, the entire pattern “THU” was difficult to fabricate directly; thus, we divided it into three parts, i.e., “T,” “H,” and “U,” and we calculated their individual holograms. We then loaded the three holograms on the SLM and irradiated GO one after another.
Conclusions In this study, we have proposed a method based on spatial beam shaping technology and the G-S algorithm to realize reductive patterning on GO. The proposed method is compared with the Gaussian laser scanning method. The micromorphologies of patterns fabricated by the two methods are different. Gullies and big humps (~5μm) were observed in areas irradiated by the shaped laser, and lamellar structures were observed on the humps. In areas scanned by the unshaped laser, the humps were smaller (~1μm), and there were many floccule structures in these areas. Morphology observations demonstrate that the spatially-shaped laser irradiating method has good repeatability, and the final pattern does not change if the holograms loaded on the SLM are the same. Raman spectra demonstrate the reduction effect of the two methods. Longer irradiation time and larger fluence lead to better reduction effect in spatially-shaped laser irradiating procedure. Similarly, shorter scanning speed and larger fluence lead to reduction in laser scanning method. By adjusting related parameters, we obtained similar reduction extent in both methods. Here, the efficiency of the shaped laser irradiating was approximately 400 times that of the unshaped laser scanning. Finally, we applied the spatially-shaped laser irradiating method to fabricating more complicated patterns by tailoring and splicing the entire pattern, and the results confirmed the good flexibility of the proposed method. With improved efficiency and good repeatability and flexibility, the proposed method is expected to be applicable to the fabrication of GO-based microcircuits, microdevices, and many other related devices.
1 引言
石墨烯是一类重要的二维材料,自被发现以来引起了大量关注,具有优良的机械性能、高导电性和导热性等,已被广泛应用于多个领域[1]。制备高质量的石墨烯具有很大挑战性,目前常用的制备方法包括机械剥离石墨[2]、化学气相沉积[3]、外延生长[4]等。氧化石墨烯(GO)是一大类带有羟基、环氧和羧基等含氧官能团的石墨烯衍生材料[5],其碳层结构中大量的sp2碳碳键转化成为sp3键,其性质不同于石墨烯[6]。诱导GO还原是制备类石墨烯材料的重要途径[7],还原方法有化学还原[8]、热还原[9]和激光诱导还原[10]等,其中激光诱导还原法具有较好的灵活性和区域选择性。飞秒激光、纳秒激光和连续激光均可以诱导GO的还原,飞秒激光的效率更高[11]。飞秒激光还原GO包括两个过程,分别是碳碳键从sp3键向sp2键的转化和氧原子的去除[12]。飞秒激光逐点扫描可以在GO上加工微电路以实现线路电阻可调[13],也可以在GO上制备不同形状的超级电容,从而使GO在能量存储方面具有一定的应用潜力[14],GO在生物、传感、催化等领域也有所应用[15-16]。
在诱导GO还原时,激光逐点扫描法的加工效率低,加工大量重复图案耗时长。激光空间整形技术可以改变焦点处光场的分布,从而提升加工效率、优化加工结果,利用经过空间整形的飞秒激光可在电介质、金属等材料上进行图案化加工[17-19]。衍射光学元件(DOE)可以将飞秒激光整形为多光束光场,用于在材料上快速制备相似图案[20]。数字微镜装置(DMD)通过加载特定相位图对激光进行光场整形,可以获得具有特殊功能的光场[21]。空间光调制器(SLM)具有使用便利、可重复使用、对光场利用率高等优点,在飞秒激光微纳加工领域有着广泛的应用[22],其可以产生类型丰富的特殊光场,例如贝塞尔光束、艾里光束等,也可以产生任意图案化的光场,因此SLM在图案化加工方面具有应用潜力[23-24]。
本文采用空间整形的飞秒激光辐照GO表面,实现了对加工区域的还原图案化,这种加工方法称为空间整形激光辐照法。该方法根据Gerchberg-Saxton(G-S)算法计算整形所需的相位图[25],使用SLM加载相位图,对飞秒激光进行空间整形,使聚焦后的光场形状与待加工图案一致,通过定点辐照进行加工。作为对比的激光逐点扫描法可保持聚焦后光场的形状为点状,通过移动平移台使焦点扫过样品表面产生加工效果。文中分别采用空间整形激光辐照法和激光逐点扫描法加工GO,使用扫描电镜、白光干涉仪和拉曼光谱仪对加工区域的微观形貌、成分信息进行表征和分析。结果表明,空间整形的飞秒激光辐照法可以对GO进行图案化加工,并具有较好的灵活性和可重复性,其图案化加工效率有所提升,在制备GO基底的微电路和微器件方面有广泛的应用前景。
2 实验过程
2.1 空间整形飞秒激光加工系统
本文使用的材料是通过抽滤、干燥GO分散液的方法获得的GO薄膜[26],使用的飞秒激光波长为800nm,脉冲宽度为35fs,重复频率为1kHz。
在SLM上加载平面相位时,SLM上所有像素点均保持相同的灰度值,此时的SLM相当于一个平面反射镜,不会改变激光束的高斯分布,在这样的状态下,在物镜焦点处可得到点状光场,并可以进行激光逐点扫描。控制平移台按照设计的路线平移,可以在GO表面加工图案,通过调整激光功率和扫描速度可以改变加工效果。
2.2 基于SLM的激光空间整形原理
激光空间整形技术主要基于透镜的衍射规律,以复振幅分布来表示光场,在透镜的前焦面上,光场可以表示为
式中:(x0,y0)为透镜前焦面上一点的坐标;U0(x0,y0)表示透镜前焦面的光场分布;A0(x0,y0)表示光场的振幅分布;φ0(x0,y0)表示光场的相位分布。
根据透镜的菲涅耳衍射积分和相位变换规则,透镜后焦面的光场复振幅分布U(x,y)和U0(x0,y0)的关系为
式中:(x,y)为透镜后焦面上一点的坐标;U(x,y)表示透镜后焦面的光场分布;C为常数;ξ和η为分别与x和y轴方向垂直的坐标。
由(2)式推导可知,U(x,y)是U0(x0,y0)的傅里叶变换(FT)结果,改变U(x,y)的分布可以通过改变U0(x0,y0)来实现。根据(1)式,通过调整φ0(x0,y0)可以改变U0(x0,y0),将SLM安装在透镜的前焦面,可以实现对前焦面光场相位分布的调制。
激光空间整形需要根据目标光场的复振幅分布U(x,y)获得对应的相位分布φ0(x0,y0),实现该过程的方法是Gerchberg-Saxon算法[25],其示意图如
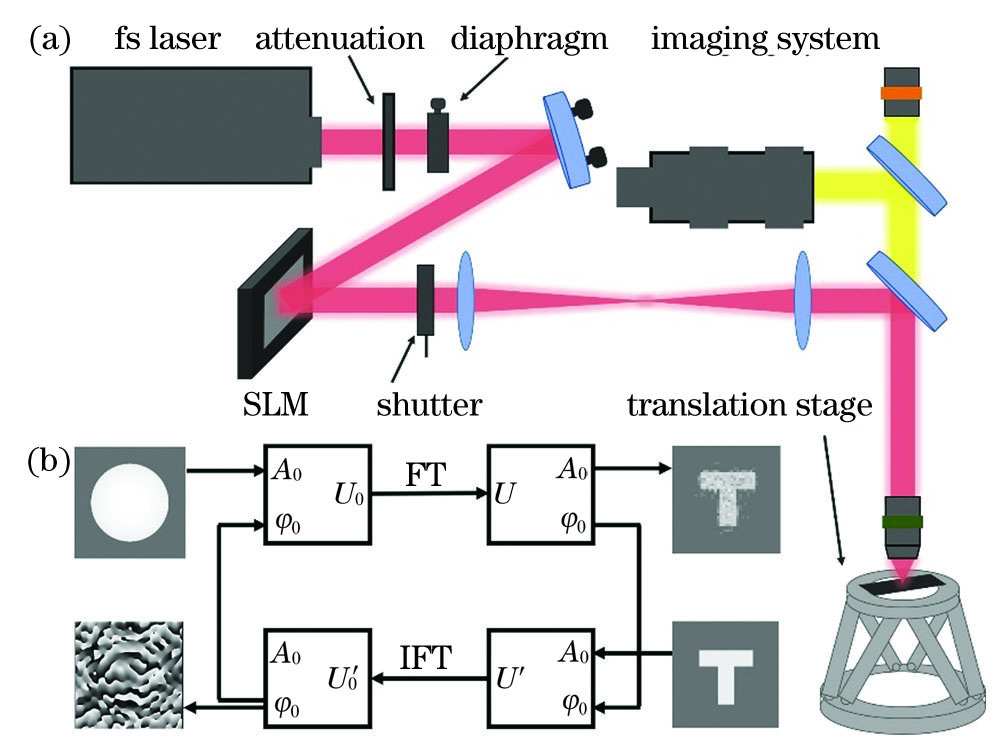
图 1. 基于SLM的飞秒激光空间整形、加工光学系统及计算相位图的算法。(a)飞秒激光空间整形、加工光学系统; (b)G-S迭代算法的示意图
Fig. 1. SLM-based optical system for space shaping and processing of femtosecond laser, and phase calculation algorithm. (a) Optical system for space shaping and processing of femtosecond laser; (b) schematic illustration of G-S iteration algorithm
2.3 加工区域表征实验
对样品进行还原图案化加工之后,使用扫描电镜对图案化区域进行表征,观察两种加工方法的图案化加工效果和微观形貌;使用白光干涉仪对加工区域的深度和均匀性进行表征;使用拉曼光谱仪获取图案化加工区域的拉曼光谱,根据拉曼光谱的特征峰的位置判断GO被飞秒激光还原的程度[11-12]。
3 分析与讨论

图 2. 两种方法加工结果的对比。(a)在SLM上加载的不同相位及获得的不同光场分布(加载计算得到的相位获得整形激光,加载平面相位获得高斯激光); (b)空间整形激光辐照区域的微观形貌; (c)激光逐点扫描区域的微观形貌; (d)空间整形激光辐照区域的深度分布; (e)激光逐点扫描区域的深度分布
Fig. 2. Comparison of processing results of two methods. (a) Two phases loaded on SLM and corresponding optical field distributions (load calculated phase to obtain spatially shaped laser and load plane phase to obtain Gaussian laser); (b) micromorphology of region irradiated by spatially shaped laser; (c) micromorphology of region for laser point-by-point scanning; (d) height distribution of spatially shaped laser irradiated pattern; (e) height distribution of region for laser point-by-point scanning
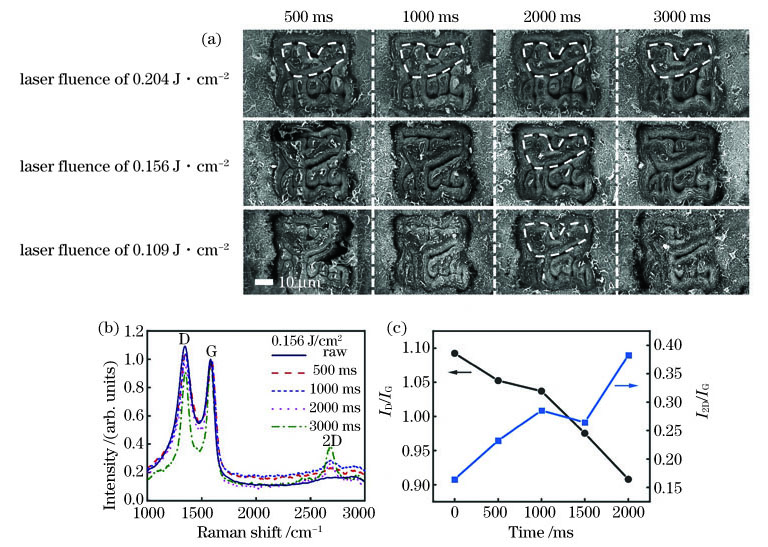
图 3. 不同辐照时间和激光通量下空间整形激光辐照法的加工结果。(a)不同辐照时间和激光通量下辐照加工结果的微观形貌;(b)加工区域的拉曼光谱(以G峰强度为基础进行归一化);(c) ID/IG和I2D/IG随时间的变化趋势
Fig. 3. Processing results of spatially shaped laser irradiation method under different irradiation time and laser fluence. (a) Micromorphology of processing results under different irradiation time and laser fluence; (b) Raman spectra of processed regions (normalized based on intensity of peak G); (c) variations of ID/IG and I2D/IG with irradiation time
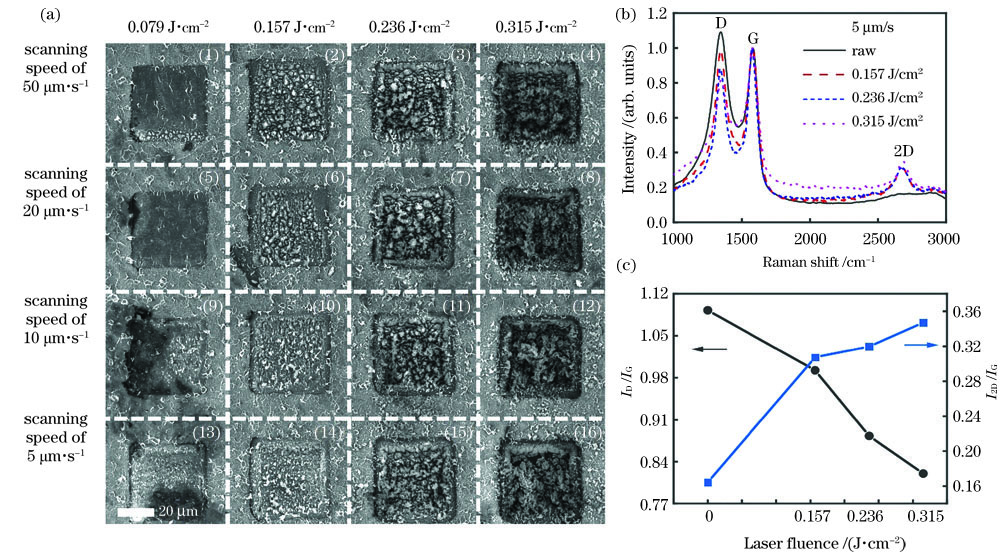
图 4. 不同激光通量和扫描速度下的激光逐点扫描法加工结果。(a)不同激光通量和扫描速度下加工区域的微观形貌;(b)不同通量的激光扫描后的拉曼光谱结果;(c) ID/IG和I2D/IG随激光通量的变化趋势
Fig. 4. Laser point-by-point scanning results under different laser fluence and scanning speed. (a) Micromorphologies of processed region for different fluence and scanning speed; (b) Raman spectra after scanning by laser under varying fluence; (c) variations of ID/IG and I2D/IG with laser fluence

图 5. 空间整形激光辐照法得到的字母图案。(a)三个字母对应的相位图;(b)根据三个相位图计算的焦点处光场分布;(c)~(e)辐照加工的三个字母的微观形貌及局部放大图
Fig. 5. Patterns of three letters fabricated by spatially shaped laser irradiation. (a) Phases corresponding to three letters; (b) simulated optical fields at focused point corresponding to three phase patterns; (c)--(e) micromorphologies of three letter patterns obtained by spatially shaped laser irradiation and local amplification maps
4 结论
本文实现了一种基于激光空间整形技术对氧化石墨烯进行还原图案化加工的方法,即空间整形激光辐照法。通过比较空间整形激光辐照法和激光逐点扫描法,发现使用两种方法得到的加工区域的微观形貌不同,空间整形激光辐照法可以减少加工过程中碎屑堆积的现象。加工区域的微观形貌表明空间整形激光辐照法加工的图案具有良好的可重复性,如果SLM加载的相位图不发生变化,则多次加工产生的图案一致。两种加工方法均对GO有还原效果,在实验参数范围内,对于空间整形激光辐照法,长辐照时间和高激光通量有助于提高还原程度,而对于激光逐点扫描法,低扫描速度和高激光通量有助于GO的还原。进行还原图案化加工时,空间整形激光辐照法需要的时间少于激光逐点扫描法,这表明采用空间整形技术可以使图案化加工GO的效率提升。对加工图案进行合理的分割,使用空间整形飞秒激光进行分区域加工可以得到形状更复杂的图案,这显示了该方法较好的图案灵活性。空间整形激光辐照法对GO进行还原图案化加工时具有较好的可重复性和图案灵活性,且图案化加工效率得到提升,该方法在制备GO基底微电路、微器件等方面具有广泛的应用前景。
[2] Novoselov K S, Geim A K, Morozov S V, et al. Electric field effect in atomically thin carbon films[J]. Science, 2004, 306(5696): 666-669.
[3] Chen Z, Ren W, Gao L, et al. Three-dimensional flexible and conductive interconnected graphene networks grown by chemical vapour deposition[J]. Nature Materials, 2011, 10(6): 424-428.
[5] WeiG. The chemistry of graphene oxide[M] //Graphene oxide. Cham: Springer International Publishing, 2015: 61- 95.
[6] Mkhoyan K A, Contryman A W, Silcox J, et al. Atomic andelectronic structure of graphene-oxide[J]. Nano Letters, 2009, 9(3): 1058-1063.
[7] Pei S F, Cheng H M. The reduction of graphene oxide[J]. Carbon, 2012, 50(9): 3210-3228.
[8] Gilje S, Han S, Wang M S, et al. Achemical route to graphene for device applications[J]. Nano Letters, 2007, 7(11): 3394-3398.
[9] Wei Z, Wang D, Kim S, et al. Nanoscale tunable reduction of graphene oxide for graphene electronics[J]. Science, 2010, 328(5984): 1373-1376.
[10] Wan Z F, Streed E W, Lobino M, et al. Laser-reduced graphene: synthesis, properties, and applications[J]. Advanced Materials Technologies, 2018, 3(4): 1700315.
[11] Arul R, Oosterbeek R N, Robertson J, et al. The mechanism of direct laser writing of graphene features into graphene oxide films involves photoreduction and thermally assisted structural rearrangement[J]. Carbon, 2016, 99: 423-431.
[12] Wan Z F, Wang S J, Haylock B, et al. Tuning the sub-processes in laser reduction of graphene oxide by adjusting the power and scanning speed of laser[J]. Carbon, 2019, 141: 83-91.
[14] Gao W, Singh N, Song L, et al. Direct laser writing of micro-supercapacitors on hydrated graphite oxide films[J]. Nature Nanotechnology, 2011, 6(8): 496-500.
[15] Kurra N, Jiang Q, Nayak P, et al. Laser-derived graphene: a three-dimensional printed graphene electrode and its emerging applications[J]. Nano Today, 2019, 24: 81-102.
[16] 柯伟铭, 李振华, 周智翔, 等. 基于还原氧化石墨烯的干涉型光纤温度传感器[J]. 光学学报, 2019, 39(12): 1206007.
[17] Ni J, Wang C, Zhang C, et al. Three-dimensional chiral microstructures fabricated by structured optical vortices in isotropic material[J]. Light: Science & Applications, 2017, 6(7): e17011.
[18] Wang C, Yang L, Hu Y, et al. Femtosecond Mathieu beams for rapid controllable fabrication of complex microcages and application in trapping microobjects[J]. ACS Nano, 2019, 13(4): 4667-4676.
[19] Wang A D, Jiang L, Li X W, et al. Mask-free patterning of high-conductivity metal nanowires in open air by spatially modulated femtosecond laser pulses[J]. Advanced Materials, 2015, 27(40): 6238-6243.
[20] Kuchmizhak A A, Porfirev A P, Syubaev S A, et al. Multi-beam pulsed-laser patterning of plasmonic films using broadband diffractive optical elements[J]. Optics Letters, 2017, 42(14): 2838-2841.
[21] Cheng J, Gu C, Zhang D, et al. High-speed femtosecond laser beam shaping based on binary holography using a digital micromirror device[J]. Optics Letters, 2015, 40(21): 4875-4878.
[22] 刘思垣, 张静宇. 基于空间光调制器的超快激光加工原理及应用[J]. 激光与光电子学进展, 2020, 57(11): 111431.
[23] 吴培超, 张晨初, 杨亮, 等. 基于空间光调制器的飞秒激光双模式快速加工[J]. 中国激光, 2018, 45(10): 1001005.
[24] Li B H, Jiang L, Li X W, et al. Flexible gray-scale surface patterning through spatiotemporal-interference-based femtosecond laser shaping[J]. Advanced Optical Materials, 2018, 6(24): 1801021.
[25] Zhang C C, Hu Y L, Du W Q, et al. Optimized holographic femtosecond laser patterning method towards rapid integration of high-quality functional devices in microchannels[J]. Scientific Reports, 2016, 6(1): 33281.
[26] Cheng H H, Liu J, Zhao Y, et al. Graphene fibers with predetermined deformation as moisture-triggered actuators and robots[J]. Angewandte Chemie, 2013, 125(40): 10676-10680.
[27] Yeh T F, Syu J M, Cheng C, et al. Graphite oxide as a photocatalyst for hydrogen production from water[J]. Advanced Functional Materials, 2010, 20(14): 2255-2262.
郭恒, 闫剑锋, 李欣, 曲良体. 空间整形飞秒激光图案化加工氧化石墨烯[J]. 中国激光, 2021, 48(2): 0202018. Heng Guo, Jianfeng Yan, Xin Li, Liangti Qu. Patterned Graphene Oxide by Spatially-Shaped Femtosecond Laser[J]. Chinese Journal of Lasers, 2021, 48(2): 0202018.