服役载荷与结构参数对塔式太阳能定日镜光学精度的影响
Solar tower thermal power generation technology has the advantages of high light-electricity conversion efficiency and flexible energy storage, and it has become one of the most important ways to achieve the goal of “carbon peak and carbon neutrality”. The heliostat is the core device to realize solar energy pooling in the whole tower photovoltaic power plant. It can focus the solar energy within a certain range around the heat-absorbing tower, so as to complete the light-heat conversion in the heat-absorbing device with high quality. However, the heliostat is susceptible to structural deformation due to self-weight and wind load during service, and the distance between the heliostat and the heat-absorbing tower is relatively large. The small deformation of the reflecting mirror surface of the heliostat will lead to the reflected solar beam falling on the heat-absorbing device, which will directly lead to a decrease in the light-concentrating efficiency of the heliostat and a deterioration in the quality of the light-energy distribution of the heat-absorbing device. Therefore, it is important to investigate the service optical accuracy of the heliostat under self-weight and wind load, which can provide a basis for its lightweight and high-precision design and service performance guarantee.
In this study, we study a typical lightweight small-scale heliostat of 20 m2. First, we establishe the three-dimensional (3D) model and the finite element simulation model. Second, we adopte a universal optical-machine integration modeling method previously proposed by the team to consider the service deformation of solar concentrators and establishe an optical-mechanical integration analytical model of the heliostat. We also investigate the influence of the key structural parameters under the effect of self-weight and wind load on the optical accuracy of the heliostat in service. The structural parameters include the spacing d and the number N of the three rows of supporting bolts at the bottom of each plane mirror, the structural parameters of the frame supporting the mirrors, and the parameters of the beam cross-section. The conclusions of the study can provide an important basis for the design of lightweight high-precision heliostat and the maintenance of heliostat's performance in service.
When the wind pressure load on the mirror surface is equal, the self-load at a height angle of 90° has the most unfavorable influence on its optical accuracy. At N=7, the maximum deformation with height angle only increases from 2.07 mm to 2.63 mm, but Dx will significantly increase from 4.21 mrad to 5.35 mrad, and Dy will only increase from 1.36 mrad to 1.73 mrad (Fig. 3). Increasing the number of supporting bolts can reduce the concave deformation of the mirror surface between adjacent bolts in a single row, thereby reducing the slope error component Dy (Fig. 4). By comparing the distribution of mirror deformation and slope error, it can be clearly observed that the maximum slope error region does not occur in the maximum deformation region, which means that there is no non-linear positive correlation between mirror total slope error and mirror deformation (Fig. 5). The decrease in stiffness of the mirror itself is the main reason for the decrease in its optical accuracy, and a support rib plate structure should be added to the back of the mirror according to actual needs, so as to enhance its load-bearing stiffness (Fig. 6). Simply increasing the size of the cantilever secondary beam angle steel may not necessarily improve the optical accuracy of the mirror but rather result in material waste. To further improve optical accuracy, efforts should be made on the structural load-bearing stiffness of the mirror itself, such as adding a support plate on the back of the mirror (Figs. 7-8). The slope of the mirror total slope error variation curve at different height angles is basically the same, with only translation differences (Fig. 9).
If the frame structure is not deformed, the deformation and slope error of the mirror surface under the same wind pressure increase with the increase in the height angle of the heliostat mirror, and the slope error component Dx along the mirror surface in the transverse direction is significantly larger than Dy along its vertical direction; the increase in the number N of mirror back bolts does not have a significant effect on Dx, but it can significantly reduce Dy,
1 引言
塔式太阳能光热发电技术具有光-电转换效率高和灵活储能等优势,已成为助力“碳达峰、碳中和”目标实现的重要技术之一[1]。定日镜是整个塔式光热电站中实现太阳光能汇集的核心装置,要求将吸热塔周边一定范围内的太阳光能高质量地聚焦于吸热器内并完成光-热转换[2]。但在定日镜服役过程中,其易受自重和风载作用而发生结构变形,且定日镜与吸热塔距离较远,定日镜反射镜面的微小变形将会使反射的太阳光束落靶于吸热器,直接导致定日镜的聚光效率降低和吸热器的光能分布品质恶化[3-6]。因此,探究自重和风载荷作用下定日镜的服役光学精度尤为重要,能为其轻量高精度设计与服役性能保障等提供参考。
近年来,有大量关于典型风载荷以及支撑结构等方面的塔式定日镜研究[7-10],例如卢春玲等[8]提出一种新的湍流脉动流场产生方法,能够预测出定日镜顺风向的等效风荷载并且模拟出流场中的紊流与涡旋;吴卫祥等[9]通过实验得到定日镜的风压分布结果和结构抗风设计参数变化,结果表明定日镜阵风系数值由四周向中心减小,且随着方位角的增大,定日镜的整体风振系数呈先减小后增大的趋势;Luo等[10]研究了定日镜结构的疲劳寿命随着风向、海拔和形状系数变化的规律。但是这些研究仅仅讨论了风载荷对定日镜力学性能的影响,并未涉及到定日镜服役光学精度的评价。随着塔式太阳能电站的大规模建设与应用,部分学者已开始关注定日镜服役光学精度或聚光性能问题[11-13],例如Ji等[11]研究了风载荷下俯仰角和风向角对定日镜聚光效率的影响;Yang等[13]提出了一种“伞形”支撑结构,并在典型风载荷下与传统定日镜结构进行比较,对比其位移、应力值以及光学效率等方面,研究表明该结构能减少定日镜质量且能够保证光学效率。以上研究虽然关注了服役光学精度,但所涉及的都是大型定日镜或者具有新型支撑结构的定日镜,对于目前应用广泛的小型轻量定日镜的服役光学精度研究报道较少。本文以典型的面积为20 m2的轻质小型定日镜为对象,采用一种考虑太阳能聚光器服役变形的普适性光-机集成建模方法,建立定日镜的光-机集成分析模型,研究自重和风载荷作用下关键结构参数对定日镜服役光学精度的影响规律,包括各平面镜底部的三列支撑螺栓的间距d、每列支撑螺栓的数量N、支撑反射镜的机架结构参数和梁截面参数等,研究结论能为轻量化高精度定日镜设计、定日镜服役性能保证等提供重要参考。
2 定日镜及其结构参数
塔式太阳能定日镜的结构示意如
定日镜的总采光面积取为常用的20 m2,单个平面镜的尺寸为2.5 m×4.0 m,玻璃银镜厚度为4.0 mm,参考青海中控德令哈电站,设置定日镜的最大工作风速为24 m/s。如
3 定日镜有限元模型与光-机集成建模方法
3.1 定日镜的有限元模型
采用Ansys Workbench 2021有限元软件建立了定日镜结构的有限元模型,如
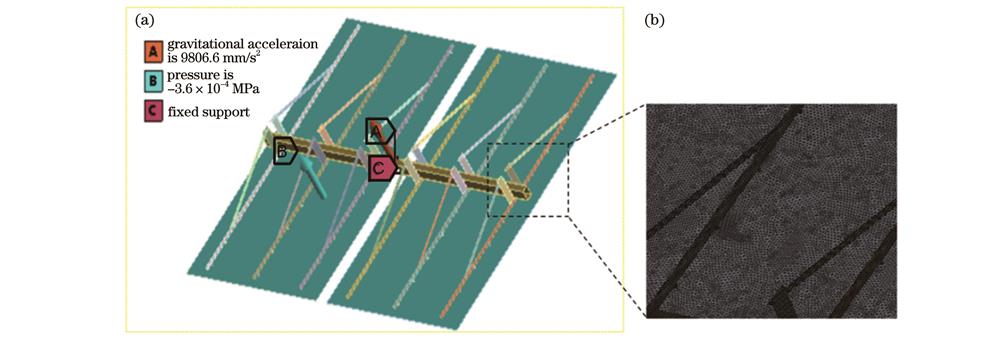
图 2. 定日镜结构有限元模型和局部网格。(a)定日镜结构有限元模型;(b)局部网格
Fig. 2. Finite element model and local mesh of heliostat structure. (a) Finite element model of heliostat structure; (b) local mesh
定日镜有限元模型的载荷及其边界约束如下:定日镜始终承受自重载荷,在不同的高度角下通过加速度分量来等效施加自重;假设定日镜的平面镜表面承受均匀的风压载荷,且风压方向由平面镜的前表面指向其后方,以最大工作风速24 m/s计算出的等效风压载荷为360 Pa。定日镜的机架部分原本与立柱顶端的双轴跟踪装置相连接,可实现其载荷传递和视日跟踪运动功能,由于所提模型仅模拟机架及其镜面的承载变形,因此将机架原连接部位均设置为固定约束。
3.2 光-机集成建模方法及镜面光学精度评价
服役载荷作用下定日镜的机械结构和反射镜面均会发生变形,从而降低其光学精度。本质而言,定日镜是以聚光为目标性能的光-机集成装置。为了实现机械变形到其光学信息的传递,采用一种考虑聚光器服役变形的光-机集成建模方法[15],该方法将聚光器的反射镜面离散成大量的平面微元,并由平面微元的变形信息直接建立其几何光学信息,实现反射镜的结构变形与数据的统一和集成,该方法适用于任何采用反射镜面的太阳能聚光系统[14-16]。采用此光-机集成建模方法,可实现载荷作用下定日镜中平面镜的变形结果到光学精度信息的传递与转换。具体的光机集成建模涉及的公式推导见文献[15]。
服役载荷作用下定日镜的反射镜面光学精度评价包括位置误差和斜率误差,其中位置误差与镜面变形后各反射点偏离理想曲面的距离有关,斜率误差是指镜面某点承载变形后的实际法线与该点理想法线之间的夹角,关注的是其法线矢量。对于定日镜而言,其对斜率误差尤为敏感,因其长距离反射会聚太阳光,微小的斜率误差会导致会集光线偏离目标区域。因此,重点关注局部镜面斜率误差及其分量,以及整体斜率误差指标。镜面上任意网格i的斜率误差[15]可表示为
式中:
镜面斜率误差
式中:M为定日镜反射镜面离散后网格的总数量;
4 结果与讨论
4.1 反射镜背部螺栓支撑间距与数量对服役光学精度的影响
定日镜中反射镜承受的服役载荷均通过其背部支撑螺栓传递给机架,这些螺栓的支撑分布是影响镜面服役光学精度的首要因素。本节假定机架结构为非变形的刚体,主要分析螺栓支撑位置参数对其服役光学精度的影响。首先,固定施加360 Pa均匀风压载荷且螺栓支撑间距d设置为900 mm,可以得到各高度角下的平面镜最大形变与斜率误差变化结果,如
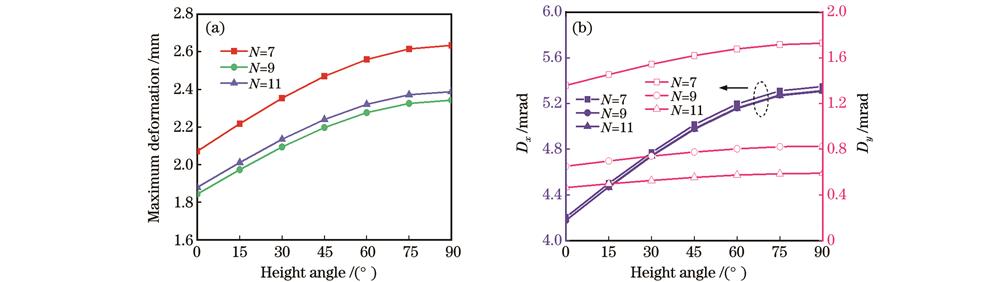
图 3. 螺栓支撑间距d=900 mm时定日镜最大变形与斜率误差随高度角的变化。(a)平面镜的最大变形;(b)镜面斜率误差分量
Fig. 3. Variation of maximum deformation and slope error of heliostat with height angle when bolt support spacing d=900 mm. (a) Maximum deformation of planar mirror; (b) specular slope error component
设置高度角为90°,在自重和最大工作风压360 Pa载荷作用下,得到不同螺栓列间距d和数量N影响下的定日镜最大变形与斜率误差结果,如
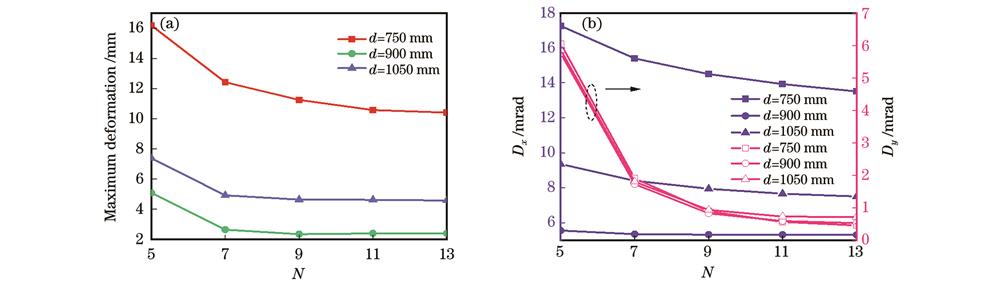
图 4. 不同螺栓支撑间距d和数量N下的定日镜最大变形与斜率误差变化。(a)平面镜的最大变形;(b)斜率误差分量
Fig. 4. Variation of maximum deformation and slope error of heliostat under different bolt support spacing d and quantity N.(a) Maximum deformation of planar mirror; (b) mirror slope error component
当反射镜背部单列支撑螺栓数量N一定时,不同螺栓支撑间距下的斜率误差分量Dy基本一致,但Dx相差明显,且反射镜的最大变形和斜率误差分量Dx并不是随着螺栓列间距d的增大呈线性变化,而是呈先减小后增大的变化趋势。螺栓支撑列间距不同会导致镜面变形区域也不同,因此需要选取合理的螺栓支撑列间距使反射镜光学精度达到最佳。如
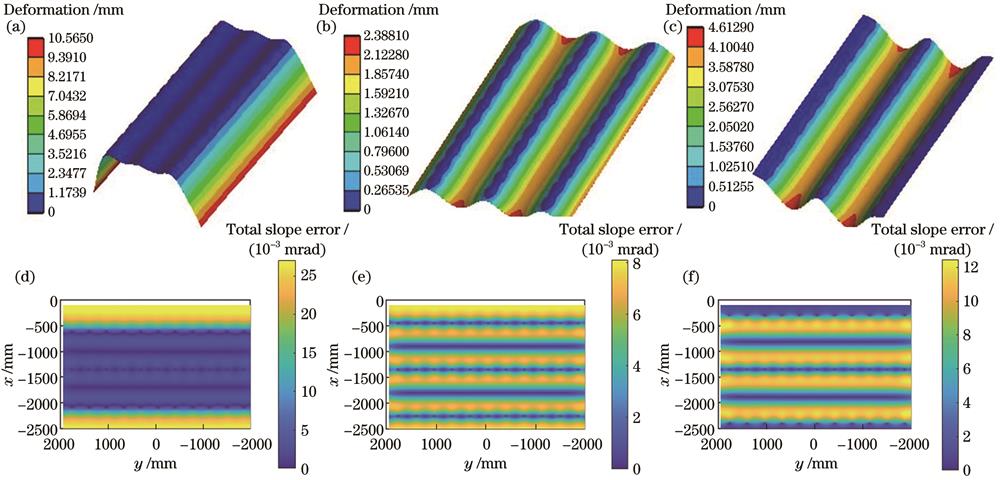
图 5. N=11时不同螺栓支撑间距d下反射镜的变形和总斜率误差分布。(a)(d)d=750 mm;(b)(e)d=900 mm;(c)(f)d=1050 mm
Fig. 5. Distribution of deformation and total slope error of mirrors under different bolt support spacing d when N=11. (a)(d) d=750 mm; (b)(e) d=900 mm; (c)(f) d=1050 mm
4.2 机架结构参数对服役光学精度的影响
4.2.1 斜支撑杆位置的影响
定日镜机架中悬臂副梁和斜支撑杆均为50 mm×50 mm×5 mm的角钢型材,考虑在最大工作风压和90°高度角自重服役载荷的条件下,斜支撑杆的不同高度位置H和末端与副梁连接位置L下反射镜服役光学精度结果,如
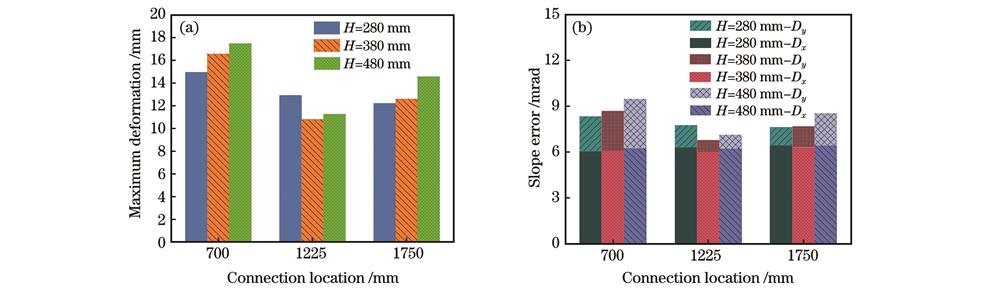
图 6. 在不同斜支撑杆的位置参数(H和L)下反射镜服役光学精度结果。(a)平面镜的最大变形;(b)镜面斜率误差分量
Fig. 6. Results of optical accuracy of mirrors in service under different position parameters (H and L) of oblique support rods. (a) Maximum deformation of planar mirror; (b) mirror slope error component
4.2.2 副梁和斜支撑杆型材截面尺寸的影响
考虑在最大工作风压和90°高度角自重服役载荷的条件下,副梁和斜支撑杆取不同等边角钢截面参数下反射镜服役光学精度结果,如

图 7. 不同角钢截面参数(a和t)下反射镜服役光学精度结果。(a)平面镜的最大变形;(b)镜面斜率误差分量
Fig. 7. Results of optical accuracy of mirrors in service under different angle steel cross-section parameters (a and t). (a) Maximum deformation of planar mirror; (b) mirror slope error component
考虑在最大工作风压和90°高度角自重服役载荷的条件下,

图 8. 不同角钢截面参数(a和t)下反射镜的变形和总斜率误差分布。(a)(c)a=50 mm,t=5.0 mm;(b)(d)a=30 mm,t=4.0 mm
Fig. 8. Distribution of deformation and total slope error of mirrors under different angle steel cross-sectional parameters (a and t). (a)(c) a=50 mm, t=5.0 mm; (b)(d) a=30 mm, t=4.0 mm
4.3 不同风压下的镜面光学精度
基于4.1~4.2节分析选取的最佳结构参数,即d=950 mm、N=11、H=380 mm、L=1225 mm、a=30 mm和t=3.0 mm,进一步模拟得到不同风压(0~360 Pa)和高度角(0°、45°、90°)组合情况下的反射镜的最大变形和斜率误差变化如
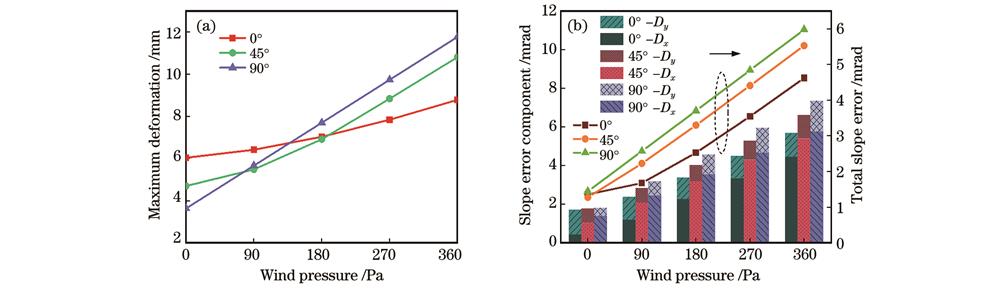
图 9. 不同风压和高度角下反射镜服役光学精度结果。(a)平面镜的最大变形;(b)镜面斜率误差分量
Fig. 9. Results of optical accuracy of mirrors in service at different wind pressures and altitude angles. (a) Maximum deformation of planar mirror; (b) mirror slope error component
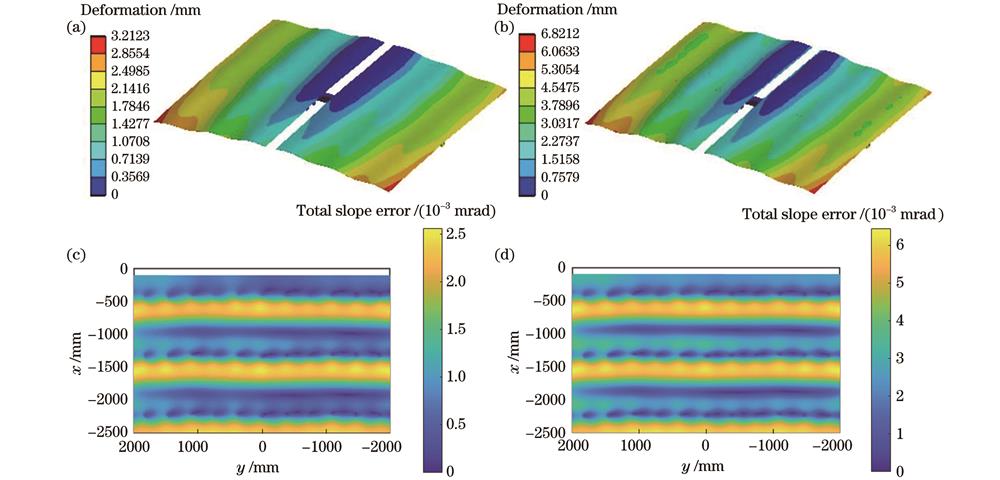
图 10. 高度角为90°时不同风压下反射镜的变形和总斜率误差分布。(a)(c)风压为0 Pa;(b)(d)风压为180 Pa
Fig. 10. Distribution of deformation and total slope error of mirrors under different wind pressures when height angle is 90°. (a)(c) Wind pressure is 0 Pa; (b)(d) wind pressure is 180 Pa
需要注意,定日镜会聚光于不同高度角,且在相同来流风速的条件下,定日镜的风载荷会随着高度角的增加而显著减小,尤其是在高度角β=90°时,风载荷最小。由文献[17]的风洞实验结果可知,定日镜正面迎风的高度角分别为0°、45°和90°的平均净风压系数分别约为1.00、0.65和0.20。由此可知,当定日镜工作于高度角β=90°时,即使来流风速达到24 m/s,也仅相当于承受72 Pa的均匀风压载荷,其服役光学精度仍非常优异。
进一步选取典型太阳位姿和定日镜场参数,初步展示载荷作用下聚焦光斑的变化。塔式电站全局坐标系o1-x1y1z1位于吸热塔底部的地平面,+z1指向天顶,+x1指向正西方,+y1指向正南方;以200 m高的塔顶为中心建立位于o1-x1z1平面的平面接收器,选取球面焦距为250 m的定日镜反射镜,建立定日镜有限元模型,首先在高度角β=90°且风压为360 Pa时,将平面镜和球面镜分别模拟得到的Dx、Dy进行对比,其Dx差值为0.32×10-4 mrad,Dy差值为0.55×10-4 mrad,且镜面变形、斜率误差分布均一致,说明大半径球面镜可采用平面镜来替代。对聚光结果进行分析,必须以球面反射镜建模。设置定日镜的反射镜面中心的镜场坐标为(0,-150,2.5),单位为m,太阳高度角为60°,方位角为90°或135°两种情况(正东方向的方位角为0°)。在理想、仅自重、自重+180 Pa风压条件下,塔顶平面接收器的聚焦光斑变化如
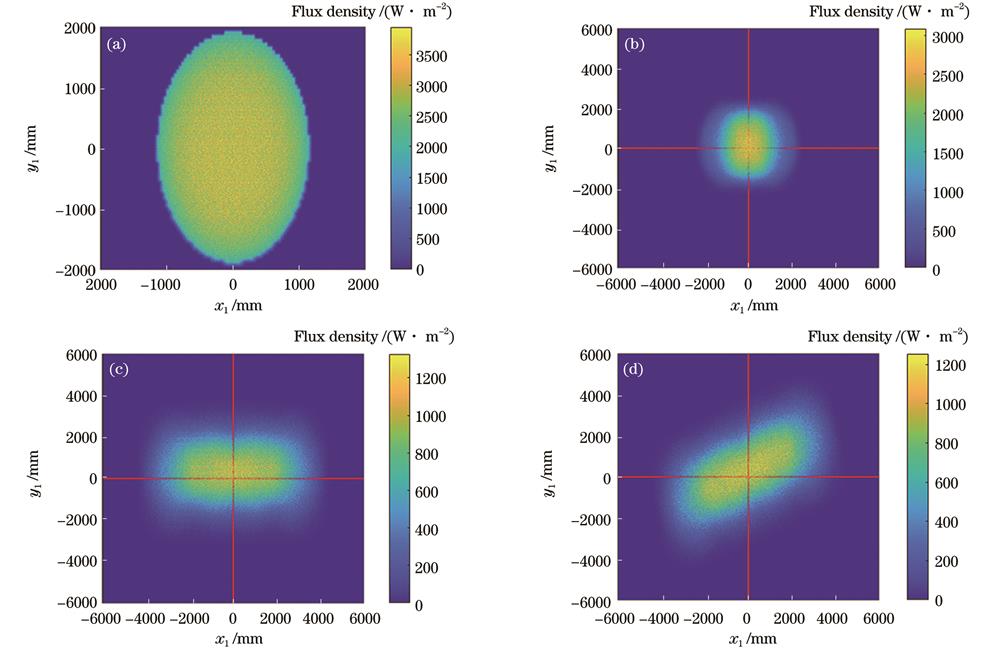
图 11. 不同情况下聚焦光斑能流密度分布(太阳直射辐照强度为1000 W/m2)。(a)~(c)太阳高度角和方位角分别为60°和90°时理想镜面、仅受自重、自重+180 Pa风压的结果;(d)太阳高度角和方位角分别为60°和135°时自重+180 Pa风压工况的结果
Fig. 11. Focused spot flux density distribution under different conditions (intensity of direct sunlight irradiation is 1000 W/m2). (a)-(c) Results of ideal mirror, self-weight only, and self-weight +180 Pa wind pressure at solar altitude and azimuth angles of 60° and 90°, respectively; (d) result of self-weight +180 Pa wind pressure at solar altitude and azimuth angles of 60° and 135°.
即使反射镜面具有相同的变形,但塔式电站镜场中不同位置处定日镜和不同服役时刻(太阳位姿)的聚焦光斑也会存在显著差异。总的来说,服役载荷下定日镜聚光性能具有复杂的时-空变化特性,未来需要更深入探索此规律,为高效高质量聚光服役提供基础。
5 结论
以典型小型轻质定日镜为对象,采用光-机集成建模方法,研究了自重和风载荷作用下关键结构参数对定日镜服役光学精度的影响规律,包括各平面镜底部的三列支撑螺栓的间距d、每列支撑螺栓的数量N、副梁和斜支撑杆的角钢截面几何参数,以及斜支撑杆的位置参数(H和L)等,为设计高精度定日镜、保持定日镜服役性能等方面提供重要依据。主要结论如下:
1)假定机架结构未变形,相同风压下镜面的变形和斜率误差均随定日镜高度角的增加而增大,且沿镜面横向的斜率误差分量Dx要明显大于沿其竖向的Dy;反射镜背部支撑螺栓数量N增加对Dx影响不显著,但能显著减小Dy,不过当N≥11时改善效果不再明显;反射镜背部支撑螺栓的列间距d存在合理值使其光学精度达到最佳。结果表明在最佳参数d=950 mm和N=11条件及360 Pa风压和自重作用下,镜面最大变形只有2.38 mm,镜面斜率误差Dx和Dy分别为5.31 mrad、0.59 mrad。
2)机架结构变形对反射镜面的Dx和Dy的贡献分别为0.72 mrad、0.17 mrad,即机架结构刚度较强,反射镜面自身刚度的减小是其服役光学精度下降的主要原因。
3)机架中角钢型材的边长a在30~50 mm范围内对服役光学精度影响较小,而厚度t的影响更为显著。当t=4.0 mm时,a=30 mm与a=50 mm的服役光学性能相当,以青海中控德令哈50 MW塔式太阳能电站为例,前者能减少1302吨钢材。
4)优选结构参数为d=950 mm、N=11、H=380 mm、L=1225 mm、a=30 mm和t=3.0 mm,在不同风压(0~360 Pa)和高度角(0°、45°、90°)组合下,结构变形与镜面斜率误差均随着风压的增加而线性增加,最大变形在3.63~11.76 mm范围内;而不同高度角β下总镜面斜率误差变化曲线的斜率基本一致,仅存在平移差异。当β=90°时,得到的镜面总斜率误差拟合曲线斜率为0.0126,截距为1.439;在仅自重载荷作用下,高度角0°~90°内总斜率误差在1.27~1.45 mrad。
5)镜面变形与其斜率误差分布规律完全不同,镜面斜率误差与镜面变形之间并非呈线性正比例关系,工程实践中如以镜面变形为约束来优化设计或评估定日镜结构,则在服役载荷作用下不一定能获得满意的光学精度,应该用光学精度对其进行评价和指导定日镜结构优化设计。
[1] 王志峰, 何雅玲, 康重庆, 等. 明确太阳能热发电战略定位促进技术发展[J]. 华电技术, 2021, 43(11): 1-4.
Wang Z F, He Y L, Kang C Q, et al. Strategic positioning of solar thermal power generation to promote technological progress[J]. Huadian Technology, 2021, 43(11): 1-4.
[2] 魏秀东, 卢振武, 林梓, 等. 塔式太阳能热发电站镜场的优化设计[J]. 光学学报, 2010, 30(9): 2652-2656.
[3] He Y L, Qiu Y, Wang K, et al. Perspective of concentrating solar power[J]. Energy, 2020, 198: 117373.
[4] Blume K, Röger M, Pitz-Paal R. Simplified analytical model to describe wind loads and wind-induced tracking deviations of heliostats[J]. Solar Energy, 2023, 256: 96-109.
[6] 戴贵龙, 庄莹, 王孝宇, 等. 曲面太阳辐射能流密度测量实验研究[J]. 激光与光电子学进展, 2023, 60(5): 0529001.
[7] Gong B, Li Z N, Wang Z F, et al. Wind-induced dynamic response of heliostat[J]. Renewable Energy, 2012, 38(1): 206-213.
[8] 卢春玲, 陈建通, 陈锦焜, 等. 基于LES和DES的定日镜结构风致响应分析[J]. 振动与冲击, 2022, 41(11): 298-306.
Lu C L, Chen J T, Chen J K, et al. Wind induced response analysis of heliostat structure based on LES and DES[J]. Journal of Vibration and Shock, 2022, 41(11): 298-306.
[9] 吴卫祥, 李正农, 王志峰. 塔式太阳能定日镜抗风设计参数研究[J]. 太阳能学报, 2021, 42(6): 191-197.
Wu W X, Li Z N, Wang Z F. Investigation on wind-resistant design parameters of solar power tower's heliostat based on wind tunnel experiments[J]. Acta Energiae Solaris Sinica, 2021, 42(6): 191-197.
[10] Luo H, Li Z, Xiong Q, et al. Study on the wind-induced fatigue of heliostat based on the joint distribution of wind speed and direction[J]. Solar Energy, 2020, 207: 668-682.
[11] Ji B F, Qiu P H, Xu F, et al. Concentrating efficiency loss of heliostat with multiple sub-mirrors under wind loads[J]. Energy, 2023, 281: 128281.
[12] Thalange V C, Dalvi V H, Mahajani S M, et al. Design, optimization and optical performance study of tripod heliostat for solar power tower plant[J]. Energy, 2017, 135: 610-624.
[13] Yang M C, Zhang Y L, Wang Q G, et al. A coupled structural-optical analysis of a novel umbrella heliostat[J]. Solar Energy, 2022, 231: 880-888.
[14] 颜健, 彭佑多, 刘永祥, 等. 载荷作用下太阳能聚光器两种光-机集成建模方法的验证[J]. 光学学报, 2022, 42(20): 2022001.
[15] Jian Y, Peng Y D, Liu Y X. An optical-mechanical integrated modeling method of solar dish concentrator system for optical performance analysis under service load[J]. Energy, 2022, 261: 125283.
[16] Yan J, Peng Y D, Liu Y X. Wind load and load-carrying optical performance of a large solar dish/stirling power system with 17.7 m diameter[J]. Energy, 2023, 283: 129207.
[17] 刘镇华, 牛华伟, 李红星, 等. 基于刚性模型与气弹模型风洞试验对比的塔式定日镜风振响应研究[J]. 振动与冲击, 2022, 41(8): 134-140, 187.
Liu Z H, Niu H W, Li H X, et al. A study on wind vibration response of a tower heliostat based on comparison of a rigid model and an aeroelastic model wind tunnel test[J]. Journal of Vibration and Shock, 2022, 41(8): 134-140, 187.
Article Outline
李彬, 颜健, 周炜, 彭佑多. 服役载荷与结构参数对塔式太阳能定日镜光学精度的影响[J]. 光学学报, 2024, 44(6): 0623001. Bin Li, Jian Yan, Wei Zhou, Youduo Peng. Influence of Service Load and Structural Parameters on Optical Accuracy of Solar Tower Heliostat[J]. Acta Optica Sinica, 2024, 44(6): 0623001.