基于拉曼信号分段重构的分布式光纤测温空间分辨率提升研究
0 引 言
分布式光纤拉曼测温系统利用光纤中传输的背向拉曼散射信号实现空间温度场的实时监测[1-2],相比于传统测温方法[3-5],该技术不仅能够实现长距离温度监测,还具有抗电磁干扰、响应速度快、易于铺设等优点,在各领域有着广泛应用[6-9]。目前分布式光纤测温系统受激光脉冲宽度等因素的影响,空间分辨率指标无法得到有效提升[10]。传统方法是通过压缩入射激光脉冲宽度等方法来提高系统空间分辨率,但激光脉冲宽度不能无限压缩,且随着脉冲宽度压缩,系统信噪比也随之变差,测温精度难以保证[11-12],限制了实际应用效果和应用范围。
文中对高温区域处的原始拉曼信号进行分析,根据所在温度区域的不同对拉曼信号进行了分段,去除分段中已知温度段的信号,以待测温度段信号作为增益来重构拉曼信号,该方法通过消除已知温度段信号串扰来突破激光脉宽对分布式光纤测温系统空间分辨率的限制,提高测温精度和空间分辨率。
1 温度解调原理与改进
1.1 温度解调方法
分布式光纤拉曼测温系统根据自发背向拉曼散射产生的Stokes光和anti-Stokes光解析光纤温度信息,其中anti-Stokes光对温度敏感作为信号光,Stokes光对光敏感度低可作为参照光,利用anti-Stokes光与Stokes光比值消除激光器光功率波动等因素[13],公式(1)为温度解调公式:
式中:
1.2 拉曼信号分段与重构
拉曼信号分段与重构是通过分析高温区域拉曼信号特征,根据温度梯度将信号分为已知温度段和待测温度段,根据已知温度段与待测温度段的拉曼信号光强差重构高温区域信号。首先要确定待测温度区域的长度,由于Stokes光和anti-Stokes光对温度变化展现的特性一致,只是敏感度存在区别,且短距离内两路光的传播速度差异可以忽略,为了方便,将Stokes信号和anti-Stokes信号统称为拉曼信号。图1为光脉冲传输过程示意图,将一段长为
因此,当激光脉宽为系统空间分辨率的主要制约因素时,可将空间分辨率与测温精度关系分两种情况讨论,当待测区域处测试光纤长度大于等于激光脉冲宽度时,系统测温精度主要与信号信噪比有关。当待测区域处测试光纤长度小于激光脉冲宽度时,测温精度主要与激光脉冲宽度有关,随着激光脉宽的增加,系统测量温度值逐渐偏离待测区域真实温度值,该理论为空间分辨率受限时,测温精度变差的主要原因。其中该两种情况中受高温区影响的拉曼信号长度
式中:
式中:
当满足高温区域两旁温度相等都为
式中:
那么公式(3)和(4)的差值就为在长度
进一步求出在脉宽尺度
重构温度为
将采集的Stokes和anti-Stokes强度值分别用公式(3)~(7)进行修正,将修正后的拉曼信号代入公式(8)进行温度解调,得:
式中:
2 温度解调原理与改进
2.1 系统结构
为了验证拉曼信号分段与重构后的温度修正效果,搭建了实验测试平台,如图2所示。该平台由脉冲激光器、波分复用器(Wavelength Division Multiple-xing, WDM)、光电探测器(Avalanche Photo Diode, APD)、数据采集卡(Data Acquisition Card, DAC)、传感光纤、智能恒温槽(Intelligent Thermostat Tank)组成。
2.2 高温区域长度测试
用两组脉冲宽度来验证公式(2)高温区域长度检测方法的实际效果,实验中取14圈周长为0.24 m的测试光纤放入智能恒温槽,温度设定为80.0 ℃,其余光纤放置在室温(21.0 ℃)下,智能恒温槽内光纤长度即为高温区域长度。第一组实验将激光器脉宽设定为20 ns,第二组实验将激光器脉宽设定为50 ns。如图3(a)、(b)所示,Ratio为采集的anti-Stokes与Stokes的比值。受高温区域影响的拉曼信号长度为光脉冲刚接触高温区域至刚好完全离开高温区域,如图1中即为
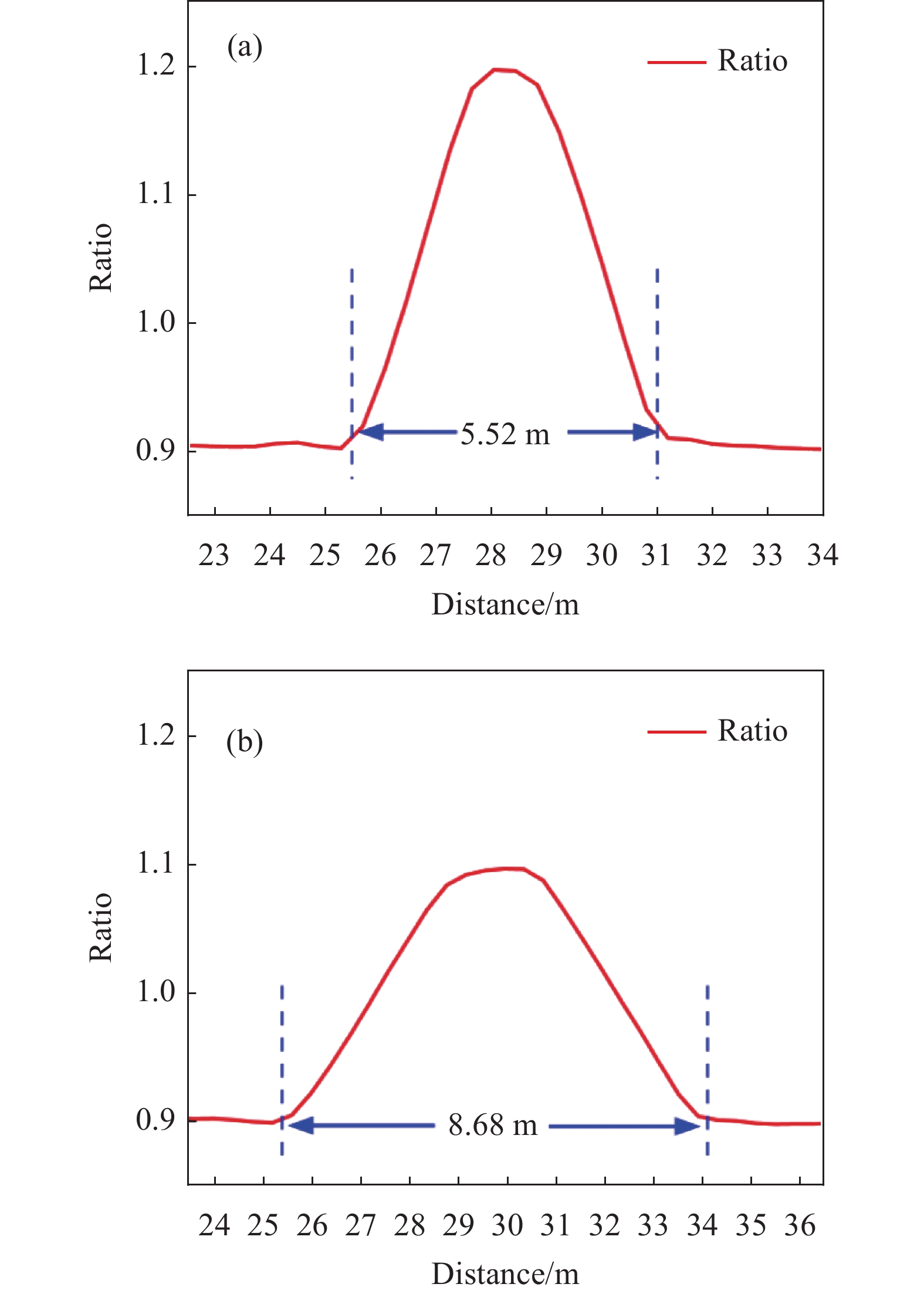
图 3. (a)在激光脉宽20 ns下的Ratio曲线 ;(b)在激光脉宽50 ns下的Ratio曲线
Fig. 3. (a) Ratio curve at a laser pulse width of 20 ns; (b) Ratio curve at a laser pulse width of 50 ns
2.3 拉曼信号分段重构测试结果
下面进行一个高温区域温度测试来验证该拉曼信号重构的实际效果。在实验中激光脉宽设置为20 ns,数据采集卡采样速率为250 MSPS,APD带宽为100 MHz,根据侯培国[16]给出的理论计算公式,系统理论空间分辨率经计算结果为2.27 m,由APD和数据采集卡限制的空间分辨率为1.07 m。取3圈周长为0.24 m、总长为0.72 m的测试光纤放入智能恒温槽,智能恒温槽中实际温度为92.1 ℃,传统解调算法下的温度曲线如图4所示,可以看出,传统温度解调算法下的测试光纤温度为58.2 ℃,温度误差为33.9 ℃。根据拉曼信号轨迹确定智能恒温槽影响的温度范围为1.13 m,经拉曼信号重构后温度解调结果为86.3 ℃,实测温度达到了实际温度的90%以上[17]。此前已有多次报道温度精度优于1 ℃的实验[18-19],这些方法是基于测试光纤足够长的情况下(大于空间分辨率),针对提升信噪比来优化测温精度。文中方法主要解决了当测温区域长度小于激光脉冲宽度的条件下造成的测温偏移,对于小范围泄漏监测具有突出优势。其中温度误差主要来源于APD带宽不足,该方法可随APD带宽的提高逐渐缩短可准确测量的温度区域长度和温度精度。
测试该方法不同的温度点,表1为40~90 ℃的实验测试,图5为传统解调方式下和拉曼信号重构后温度解调下的误差对比,图中左轴为传统解调方法温度误差,右轴为拉曼信号分段重构方法的温度误差。由于系统空间分辨率不足,在传统解调方法下产生了较大的测温误差,经该方法提升空间分辨率后,温度误差由最大的33.9 ℃提升至5.8 ℃以内,在0.72 m的测试光纤下达到了真实温度的90%以上,可以看出,经重构后系统温度精度和空间分辨率都得到了较高的提升。
表 1. 传统温度解调与重构后温度解调对比
Table 1. Comparison of traditional temperature demodulation and reconfigured temperature demodulation
|
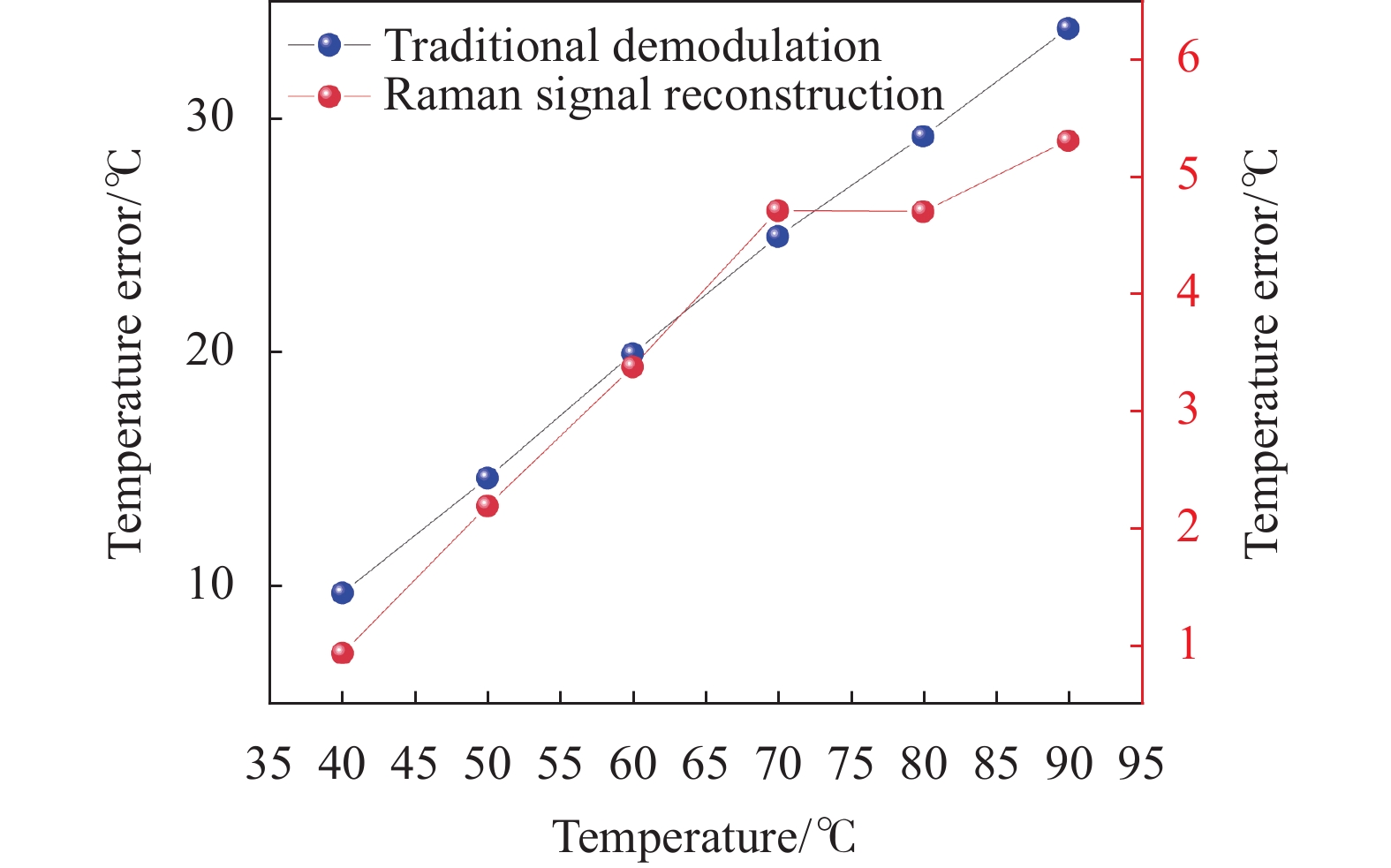
图 5. 传统解调方法与拉曼信号分段与重构方法温度误差对比
Fig. 5. Temperature error between conventional demodulation method and Raman signal segmentation and reconstruction method
3 结 论
文中分析了分布式光纤测温系统激光脉冲覆盖的高温区域拉曼信号特征,提出了一种拉曼信号分段与重构方法,根据温度梯度对高温区域拉曼信号进行分段,分为已知温度段和待测温度段,根据不同温度下待测温度段与已知温度段的拉曼信号强度差值实现信号重构。并采用20 ns脉宽激光光源与0.72 m光纤测试段进行了不同温度实验,结果表明,使用该方法后系统空间分辨率从2.27 m提升至1.13 m,测试段光纤最大温度误差从33.9 ℃减小到5.8 ℃。该方法可为油气储罐密封圈火灾、高温炉体裂缝等高空间分辨率温度监测应用提供有力支撑。
[1] 何祖源, 刘银萍, 马麟, , et al. 小芯径多模光纤拉曼分布式温度传感器[J]. 红外与激光工程, 2019, 48(4): 0422002.
[2] 许扬, 李健, 张明江. 拉曼分布式光纤温度传感仪的研究进展[J]. 应用科学学报, 2021, 39(5): 713-732.
[3] 廖光萌, 何建新, 朱玉琴, et al. 光纤光栅传感器及其应用[J]. 装备环境工程, 2022, 19(11): 142-149.
Liao Guangmeng, He Jianxin, Zhu Yuqin, . Fiber grating sensor and application[J]. Equipment Environmental Engineering, 2022, 19(11): 142-149.
[4] 杨甜甜, 郭涛, 陈展, 等. 温度传感器的应用研究[J]. 内江科技, 2020, 41(3): 38-42.
[5] 张利军. 工业热电偶测温原理及故障分析[J]. 科技资讯, 2022, 20(2): 42-44.
Zhang Lijun. Temperature measurement principle and fault analysis of industrial thermocouple[J]. Science & Technology Information, 2022, 20(2): 42-44.
[7] 李震, 冷先伦, 殷秋雨. 基于分布式光纤监测的隧道火灾温度分布特征研究[J]. 现代隧道技术, 2022, 59(6): 132-139.
[8] 刘翔. 分布式光纤测温系统在核电厂的应用实践[J]. 电子技术应用, 2022(S1): 38-43.
[9] 周铂承, 樊昕昱, 何祖源. 基于强度调制型啁啾脉冲压缩法的分布式喇曼温度传感器[J]. 光通信技术, 2021, 45(10): 30-33.
[13] 鲁佳慧, 万成功, 金恺, , et al. 分布式光纤测温系统精度和实时性优化研究[J]. 激光杂志, 2023, 44(1): 62-67.
[14] 张汝山, 吴硕, 涂勤昌, , et al. 高空间分辨率分布式光纤测温系统的设计及应用[J]. 光学仪器, 2015, 37(1): 79-82.
[15] 孙苗, 杨爽, 汤玉泉, , et al. 基于拉曼散射光动态校准的分布式光纤温度传感系统[J]. 物理学报, 2022, 71(20): 200701.
[16] 侯培国. 分布式光纤温度传感系统的理论与实验研究[D]. 燕山大学, 2003.
Hou Peiguo. They experiment research on fiber optic distributed temperature sens system[D]. Qinhuangdao: Yanshan University, 2003. (in Chinese)
[17] 王玎睿, 邓霄, 张均, , et al. 面向冰盖剖面的高空间分辨率分布式光纤测温系统设计[J]. 应用光学, 2021, 42(5): 941-948.
Article Outline
李硕, 王纪强, 高忠国, 高建新, 侯泽民, 姜龙, 侯墨语. 基于拉曼信号分段重构的分布式光纤测温空间分辨率提升研究[J]. 红外与激光工程, 2023, 52(10): 20230076. Shuo Li, Jiqiang Wang, Zhongguo Gao, Jianxin Gao, Zemin Hou, Long Jiang, Moyu Hou. Research on spatial resolution improvement of distributed optical fiber temperature measurement based on Raman signal segmentation and reconstruction[J]. Infrared and Laser Engineering, 2023, 52(10): 20230076.