排斥抛物势中相互作用时空调控的超冷玻色气体孤子的反射、局域、穿越与振荡行为操控
The experimental realizations of collisionally inhomogeneous ultracold Bose gases have not only greatly broadened the research scopes of ultracold atomic physics but also provided an ideal platform to explore novel quantum phenomena. Especially, studies on the peculiar properties and the novel quantum states and their quantum control in collisionally inhomogeneous ultracold Bose gases have been hot topics in the fields of both ultracold atomic physics and quantum information. The bright solitons of ultracold Bose gases have potential applications in quantum information, atomic interferometers, and atom lasers. Gertjerenken et al. predicted that the bright-bright solitons could be used to generate Bell states. Helm et al. found that the division and fusion of bright solitons in the ultracold Bose gases trapped in the ring well could be used to implement the Sagnac interferometer. Furthermore, in the composite potential composed of harmonic trap potential and Rosen-Morse barrier, the collision of the bright soliton can also be applied to the interferometer. In addition, stable propagation of bright solitons can be used to achieve atomic lasers. The precise control of the bright soliton in ultracold Bose gases is particularly important for the application of ultracold Bose gases.
Experiments have confirmed that the ultracold Bose gases can be controlled by adjusting the external potential and the interaction strength between atoms. With the development of experimental techniques, atomic interactions can be tuned by utilizing Feshbach resonance. In theoretical studies, several forms of time-dependent atomic interactions, such as the exponential function and the periodic function, have been proposed. The changed atomic interaction has an important effect on the properties of bright solitons in the ultracold Bose gases. For example, as atomic interaction increases, the amplitude of the bright soliton in the ultracold Bose gases increases, and its width decreases. When the atomic interaction exceeds a critical value, a transition behavior from oscillation to localization is observed in the ultracold Bose gases.
Firstly, we use the Darboux transformation to obtain the analytical solution of solitons in ultracold Bose gases with the time-dependent atomic interactions. Then, we explore the dynamics of solitons in the ultracold Bose gases with spatiotemporally modulated interactions in an expulsive parabolic potential by Crank-Nicolson method.
Firstly, we obtain the analytical solution of solitons in ultracold Bose gases by Darboux transformation. It is found that the atomic interaction increasing (decreasing) exponentially with time has a drag (push) effect on solitons in ultracold Bose gases with an expulsive parabolic potential (Fig. 2). Then, the dynamics behavior of bright solitons is explored by Crank-Nicolson method. There is a critical initial speed of solitons in the ultracold Bose gases with unchanged atomic interaction. When the initial speed of solitons is less than the critical value, a reflection behavior of solitons can be observed (Fig. 3). When the initial speed of solitons exceeds the critical value, a transmission behavior of solitons can be found (Fig. 4). When the initial speed of solitons is equal to the critical value, a localization behavior of solitons can be observed (Fig. 5). In order to investigate the effect of expulsive parabolic potential on this localization behavior of solitons, we calculate localization behaviors of solitons at different trapping frequencies. It is found that the critical initial speed of solitons increases with the trapping frequency (Fig. 6).
Subsequently, we consider the exponentially time-dependent atomic interactions. When the initial speed of solitons is equal to the critical value, a transition behavior from localization to reflection (transmission) is observed in the ultracold Bose gases with exponentially increasing (decreasing) atomic interactions (Fig. 7). When the initial speed of solitons is less than the critical value, the transition behavior from reflection to transmission is observed in the ultracold Bose gases with exponentially decreasing atomic interactions (Fig. 8). When the initial speed of solitons exceeds the critical value, the transition behavior from transmission to reflection can be found in the ultracold Bose gases with exponentially increasing atomic interactions (Fig. 9).
Finally, we calculate the dynamics behavior of bright solitons in the ultracold Bose gases with spatially modulated interactions in an expulsive parabolic potential. The localization behavior of solitons in the ultracold Bose gases with an expulsive parabolic potential can also be found (Fig. 10). Compared with that in Fig. 6, the critical local speed of the soliton in Fig. 11 decreases. Even if the initial speed is 0, the soliton can also pass through the potential barrier (Fig. 12). It is mainly attributed to the position-dependent atomic interaction. Meanwhile, a periodic oscillation of solitons with an expulsive potential barrier is newly observed (Fig. 12). The oscillation period of solitons can be controlled by tuning the atomic interaction (Fig. 13) and the trapping frequency of potential (Fig. 14).
In this paper, we analyze the reflection, localization, transmission, and oscillation behaviors of solitons in ultracold Bose gases with spatiotemporally modulated interactions in an expulsive parabolic potential, and find that: 1) the atomic interaction increasing (decreasing) exponentially with time has a drag (push) effect on solitons in ultracold Bose gases with an expulsive parabolic potential; 2) there is a critical initial speed of solitons in the ultracold Bose gases with unchanged atomic interaction, and a localization behavior of soliton can be observed at the critical speed; 3) the localization-reflection and transmission-reflection transitions of solitons are obtained by tuning the atom interactions; 4) a periodic oscillation of solitons with an expulsive potential barrier is newly observed, and the oscillation period of solitons can be controlled by tuning the atomic interaction and the trapping frequency of potential. These results can provide some help for the application of ultracold Bose gases in quantum information.
1 引言
相互作用时空调控的超冷玻色气体的实验实现,不仅极大地拓宽了超冷原子物理的研究范围,也为研究新奇量子现象提供了理想平台,研究超冷玻色气体奇异物性和新奇量子态及其量子调控已成为当前玻色-爱因斯坦凝聚体(BEC)领域的热点之一[1-12]。大量的研究证实凝聚体中亮孤子在原子激光[12]和原子干涉仪[13-17]等方面有潜在的应用价值,例如:稳定传播的亮孤子可用来实现原子激光[18];BEC中孤子的融合和分裂可应用于Sagnac干涉仪[15];亮孤子通过Rosen-Morse 势垒的碰撞分裂现象可用来实现干涉仪[17]。此外,通过调节双组分BEC中原子间相互作用,可控制亮-亮孤子的分裂和碰撞融合行为,并产生贝尔态,因此BEC在量子信息方面也具有潜在的应用价值[19]。
BEC的物性可通过外部囚禁势阱和原子间相互作用来调控[20-23]。实验中,人们发展了多种方法来调节凝聚体中原子间相互作用随时间或者空间坐标的变化,例如光学Feshbach共振技术、磁-光Feshbach共振技术等。然而,利用这些技术只能调节原子间相互作用在很小的范围内变化,并且在这个过程中BEC因丢失原子而不稳定。在近年来的实验中,基于磁Feshbach共振技术结合空间梯度磁场,可调节BEC中原子间相互作用随空间坐标的变化,并导致凝聚体中的原子在空间中分布不均,从而形成长时间存在的相互作用时空调控的凝聚体[24]。同时,在实验中观察到亮孤子的形成,相互作用时空调控的BEC的动力学行为也被首次研究[24]。理论上,通过一类变换法,张解放课题组得到了谐振势中相互作用时空调控的BEC的孤子解析解,发现孤子数量与化学势和谐振势的能级有关[25]。利用相似变换,王灯山课题组研究了一维和二维相互作用时空调控的BEC,发现在其中都存在稳定的局域非线性物质波[26-27]。当相互作用时空调控的BEC中原子间相互作用增加到一定值时,光晶格中的离散亮孤子在晶格中可保持稳定[28];当相互作用时空调控的BEC中原子间相互作用呈高斯分布时,在雪茄型凝聚体中也可形成法拉第波[29]。Sudharsan等[30]发现化学势超过临界值时,在二维相互作用时空调控的BEC中可形成稳定涡旋。大量的理论研究工作已经证明可以获得相互作用时空调控的BEC中孤子等非线性结构的解析解,但很少涉及其动力学行为。基于此,本文通过数值模拟来研究相互作用时空调控的凝聚体中亮孤子的动力学行为。结果发现,当原子间相互作用不变时,存在一个临界速率:如果孤子的初速率大于临界速率,孤子可以穿过排斥势垒;如果孤子的初速率小于临界速率,孤子运动到势垒中心时会被势垒反射回来;如果孤子的初速率等于临界速率,孤子运动到势垒中心后形成一个局域态。当原子间相互作用随时间呈指数增加时,可以实现孤子运动的局域-反射和穿越-反射转变;当原子间相互作用随时间呈指数减小时,可以实现孤子运动的局域-穿越和反射-穿越转变。此外,当原子间相互作用随空间坐标变化时,不但孤子的临界局域速度会变小,而且即使初速度为0,孤子也可穿过势垒,并产生一个周期性振荡。
2 模型
考虑相互作用时空调控的凝聚体囚禁于排斥抛物势阱中,其性质可用无量纲的非线性薛定谔方程来描述,即
式中:
3 达布变换和孤子解
为研究原子间相互作用变化对BEC中孤子动力学性质的影响,首先选取
Lax对的可积条件为
式中:
将得到的Lax对的解代入一次达布变换式
即可得到
式中:
根据
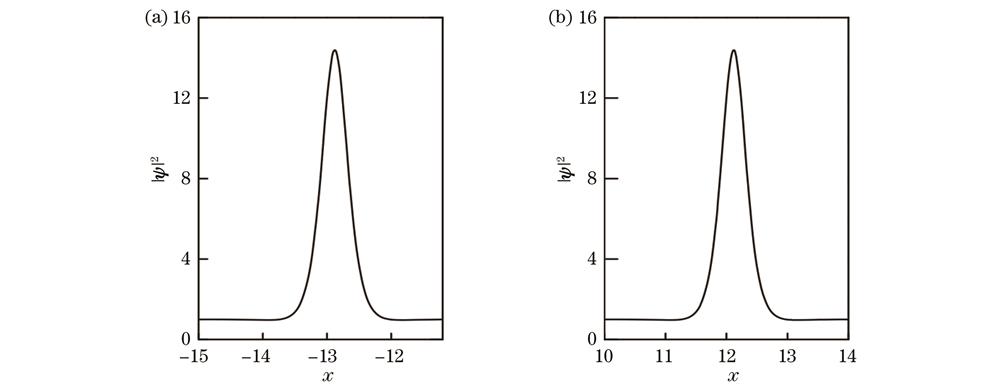
图 1. 初始时刻凝聚体密度分布( )。(a)原子间相互作用随时间呈指数增加(A=0.02);(b)原子间相互作用随时间呈指数减小(A=-0.02)
Fig. 1. Density distribution of the condensate at initial time ( ). (a) Atom interaction increases exponentially with time (A=0.02); (b) atom interaction decreases exponentially with time (A=-0.02)
4 孤子反射、局域与隧穿
考虑原子间相互作用随空间坐标x变化后,
式中:v为初始速率;x0为初始位置。为了对比,首先考虑原子间相互作用不变,选取
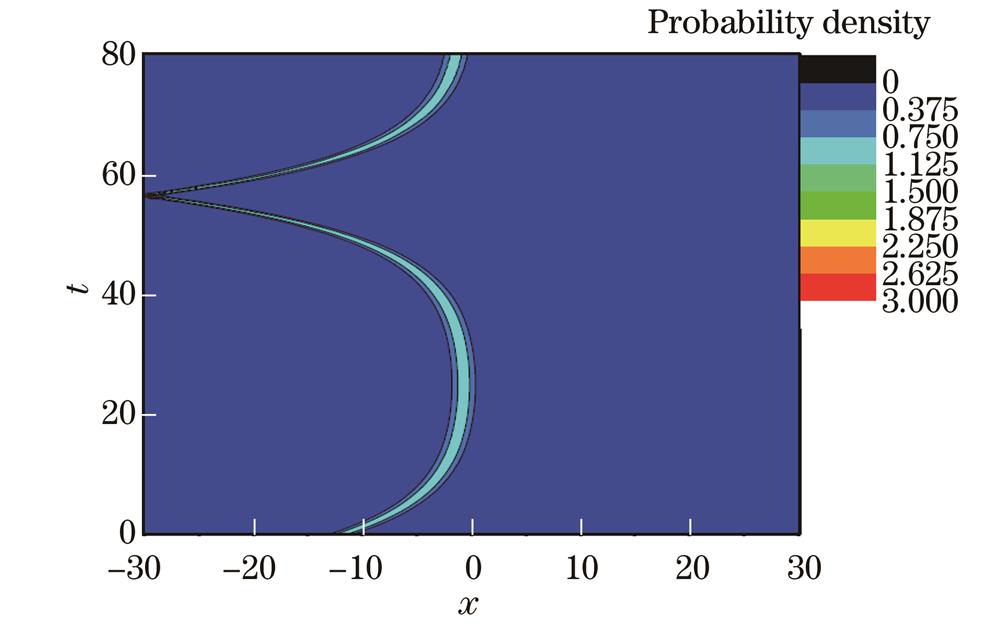
图 3. 、 、 、 时原子间相互作用不变的凝聚体中的孤子反射行为
Fig. 3. Reflection behavior of solitons in condensates with unchanged atom interaction when , , , and
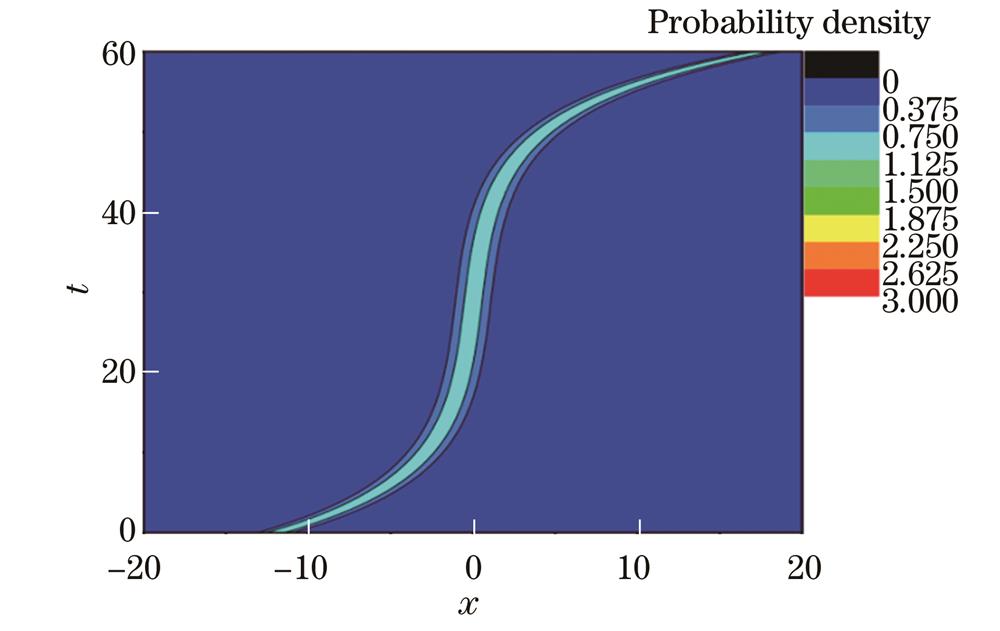
图 4. 、 、 、 时原子间相互作用不变的凝聚体中的孤子穿越行为
Fig. 4. Transmission behavior of solitons in condensates with unchanged atom interaction when , , , and

图 5. 、 、 、 时原子间相互作用不变的凝聚体中的孤子局域行为
Fig. 5. Localization behavior of solitons in condensates with unchanged atom interaction when , , , and
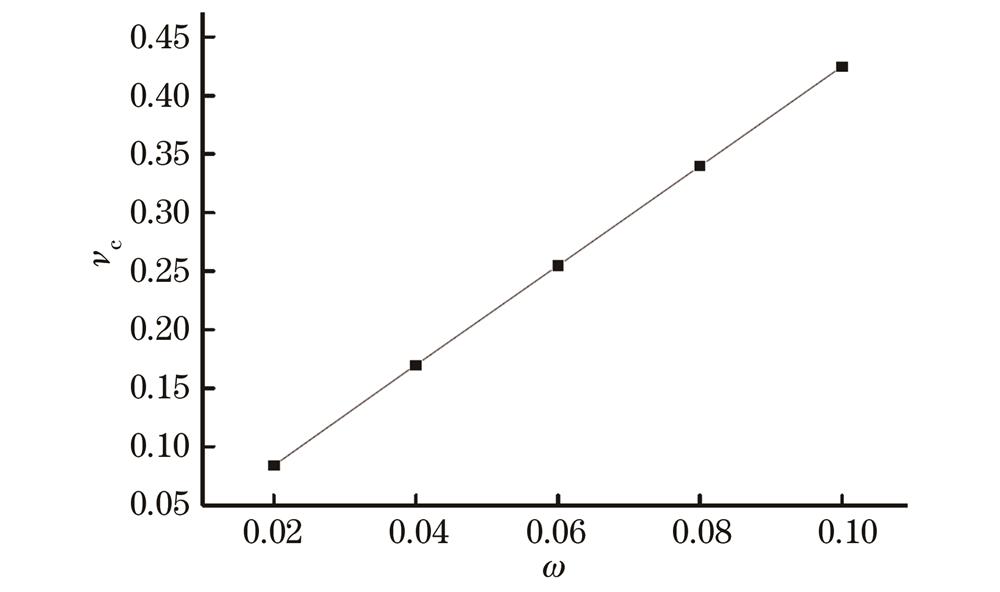
图 6. 、 时原子间相互作用不变的凝聚体中孤子的临界局域速率随囚禁频率的变化关系
Fig. 6. Critical local speed of solitons in condensates with unchanged atom interaction varying with potential frequency when and
为了研究随时间变化的原子间相互作用对孤子局域、反射和穿越行为的影响,考虑
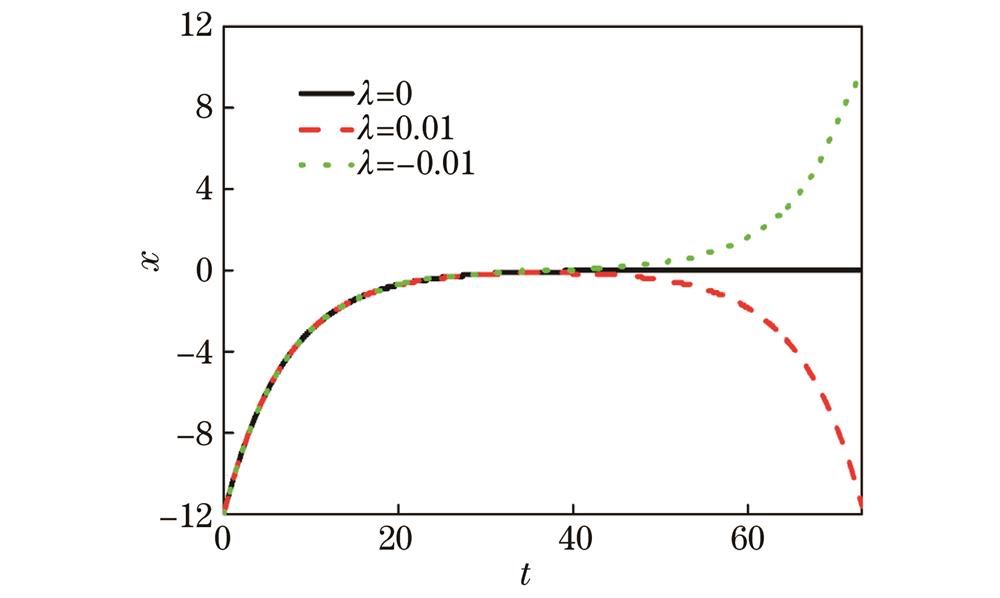
图 7. 、 、 时不同原子间相互作用下孤子的位置变化
Fig. 7. Change of soliton position under different atom interactions when , , and
接下来选取孤子的初速率小于临界局域速率,即
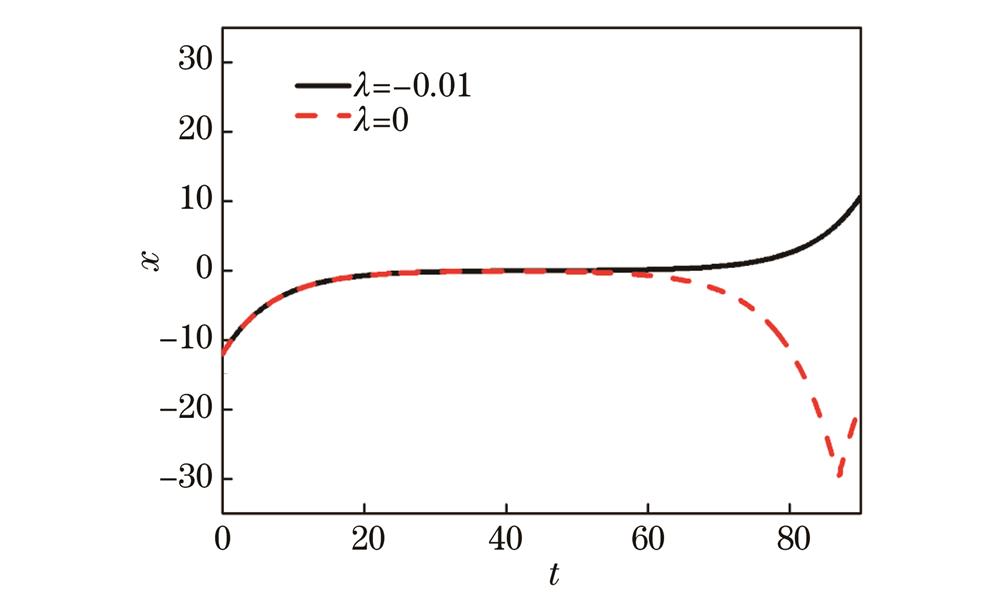
图 8. 、 、 时凝聚体中孤子的反射-穿越转变
Fig. 8. Reflection-transmission transition of solitons in condensates when , , and
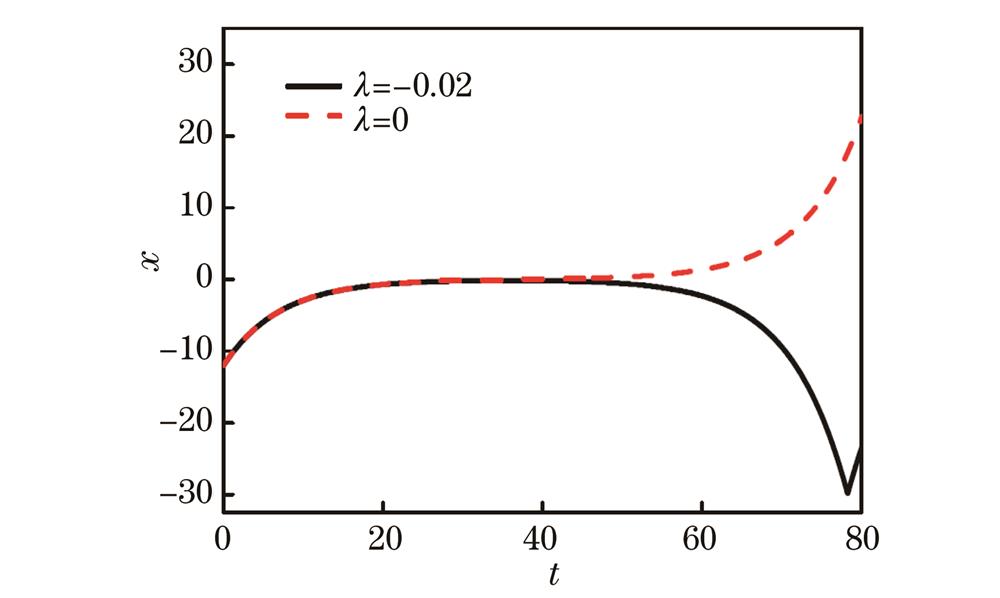
图 9. 、 、 时凝聚体中孤子的穿越-反射转变
Fig. 9. Transmission-reflection transition of solitons in condensates when , , and
为了研究相互作用时空调控的凝聚体中的孤子动力学行为,考虑S波散射长度
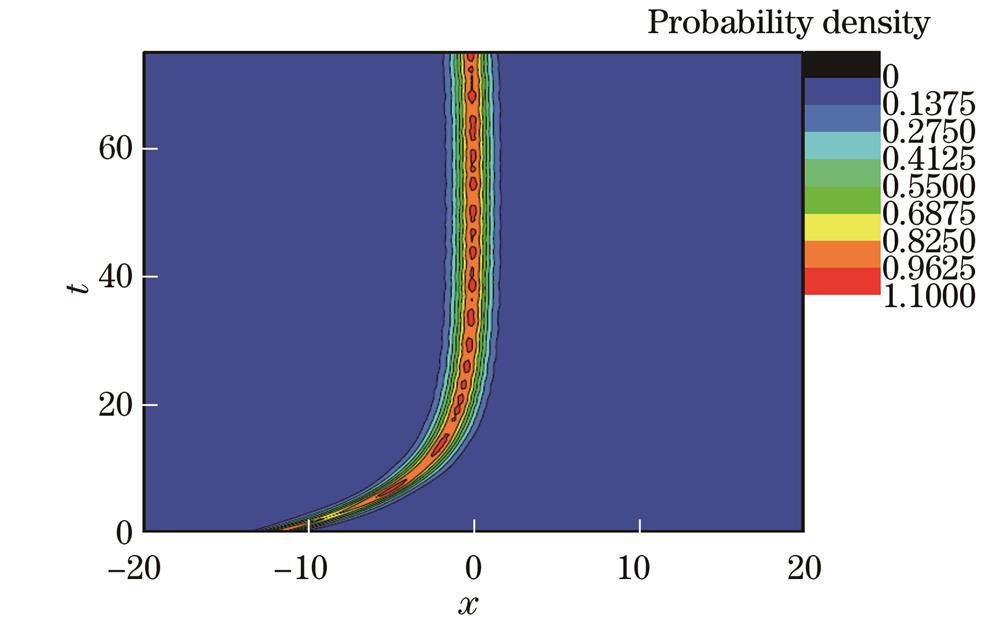
图 10. 、 、 、 时相互作用时空调控凝聚体中孤子的局域行为
Fig. 10. Localization behavior of solitons in inhomogeneous condensates when , , , and
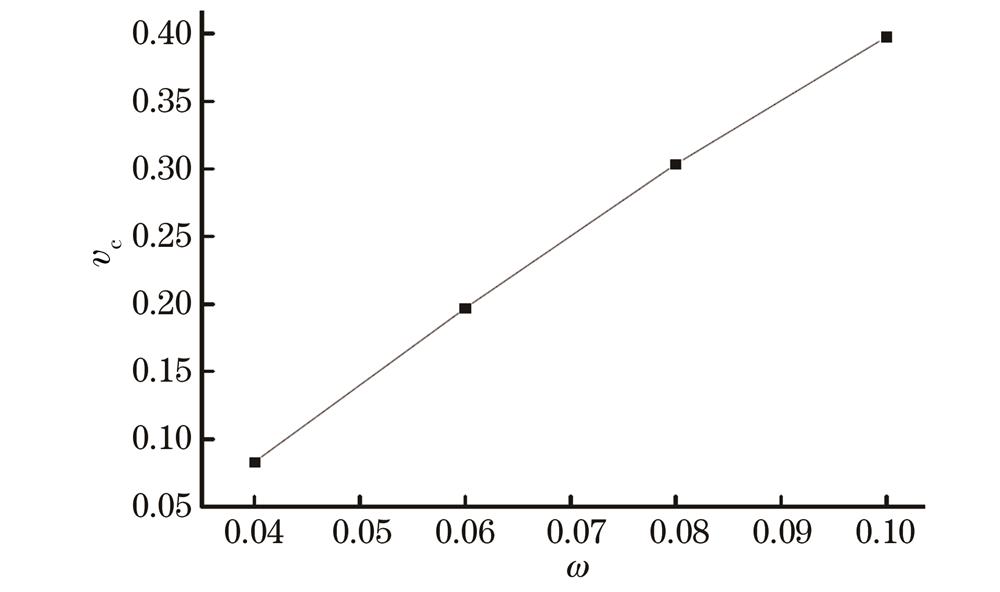
图 11. 、 时相互作用时空调控凝聚体中孤子的临界局域速率随囚禁频率的变化关系
Fig. 11. Critical local speed of solitons in inhomogeneous condensates varying with the potential frequency when and
为了研究推力

图 12. 、 、 、 时凝聚体中孤子的周期性振荡行为
Fig. 12. Periodic oscillation behavior of solitons in condensates when , , , and
为了研究原子间相互作用变化对孤子周期性振荡的影响,计算不同k值下孤子的振荡行为,相应的振荡周期随k值的变化如
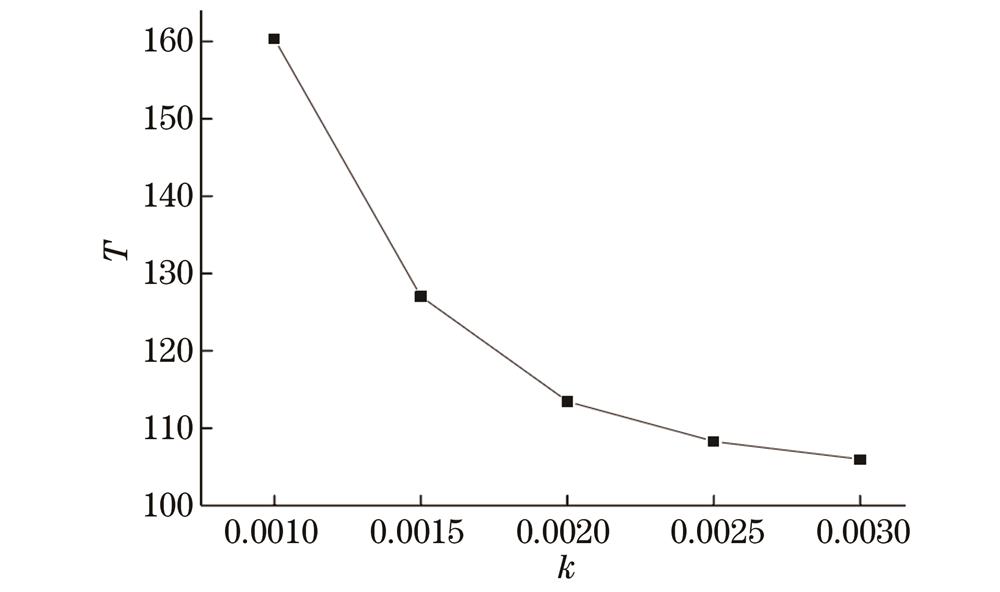
图 13. 、 、 时不同原子间相互作用下孤子的振荡周期
Fig. 13. Oscillation period of solitons under different interatomic interactions when , , and
最后,为了研究囚禁势阱对孤子周期振荡行为的影响,计算了不同囚禁频率下孤子的振荡行为,相应的振荡周期随频率
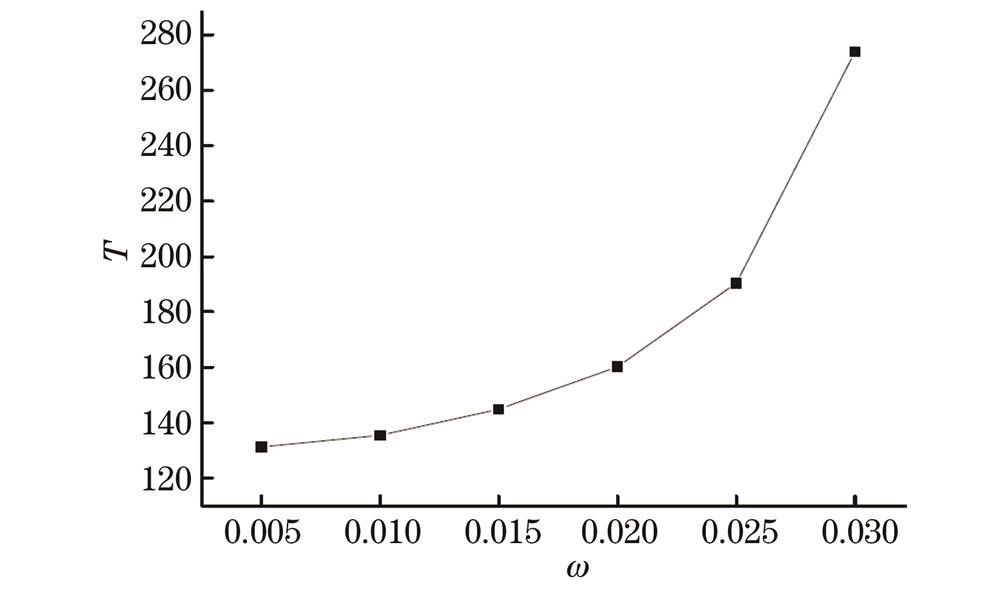
图 14. 、 、 时不同势阱囚禁频率下孤子的振荡周期
Fig. 14. Oscillation period of solitons at different potential frequencies when , , and
5 结论
对凝聚体中孤子的穿越、反射、局域和周期性振荡行为进行数值模拟。首先基于Darboux变换法,得到一维无量纲的非线性薛定谔方程的孤子解,发现随时间呈指数增加(减小)的原子间相互作用对孤子有一个阻力(推力)作用。然后,利用Crank-Nicolson方法对孤子的动力学行为进行数值模拟。当原子间相互作用不变时,存在一个临界速率:如果孤子的初速率大于临界速率,孤子可以穿过排斥势垒;如果孤子的初始速率小于临界速率,孤子运动到势垒中心处时被势垒反射回来;如果孤子的初速率等于临界速率,孤子运动到势垒中心后形成一个局域态,并且临界局域速率随着囚禁频率的增加而线性增大,即孤子局域行为可通过调节外部势来控制。当原子间相互作用随时间呈指数增加时,孤子的局域行为消失,并且即使孤子的初始速率大于临界速率,也会被势垒反射回来,实现孤子运动的局域-反射和穿越-反射转变;当原子间相互作用随时间呈指数减小时,孤子的局域现象同样消失,并且即使孤子的初始速率小于临界速率,也可以穿过势垒,实现孤子运动的局域-穿越和反射-穿越转变。此外,当原子间相互作用随空间坐标
[1] Kengne E, Liu W M, Malomed B A. Spatiotemporal engineering of matter-wave solitons in Bose-Einstein condensates[J]. Physics Reports, 2021, 899: 1-62.
[2] Kengne E, Lakhssassi A, Liu W M, et al. Phase engineering, modulational instability, and solitons of Gross-Pitaevskii-type equations in 1+1 dimensions[J]. Physical Review E, 2013, 87(2): 022914.
[3] Sakkaf L A, Uthayakumar T, Khawaja U A. Quantum reflection of dark solitons scattered by reflectionless potential barrier and position-dependent dispersion[J]. Physical Review E, 2022, 105(6): 064207.
[4] Kim M, Rabga T, Lee Y, et al. Suppression of spontaneous defect formation in inhomogeneous Bose gases[J]. Physical Review A, 2022, 106(6): L061301.
[5] Rakhimov A, Abdurakhmonov T, Narzikulov Z, et al. Self-consistent theory of a homogeneous binary Bose mixture with strong repulsive interspecies interaction[J]. Physical Review A, 2022, 106(3): 033301.
[6] He X G, Zhao D, Li L, et al. Engineering integrable nonautonomous nonlinear Schrödinger equations[J]. Physical Review E, 2009, 79(5): 056610.
[7] Cheng Y S, Adhikari S K. Localization of collisionally inhomogeneous condensates in a bichromatic optical lattice[J]. Physical Review A, 2011, 83(2): 023620.
[8] Zhong W P, Belić M R, Malomed B A, et al. Solitary waves in the nonlinear Schrödinger equation with Hermite-Gaussian modulation of the local nonlinearity[J]. Physical Review E, 2011, 84(4): 046611.
[9] Liu Q H, Qian D B. Construction of modulated amplitude waves via averaging in collisionally inhomogeneous Bose-Einstein condensates[J]. Journal of Nonlinear Mathematical Physics, 2012, 19(2): 255-268.
[10] Zhang S L, Zhou Z W, Wu B A. Superfluidity and stability of a Bose-Einstein condensate with periodically modulated interatomic interaction[J]. Physical Review A, 2013, 87(1): 013633.
[11] Yan Z Y, Konotop V V, Yulin A V, et al. Two-dimensional superfluid flows in inhomogeneous Bose-Einstein condensates[J]. Physical Review E, 2012, 85(1): 016601.
[12] 陈影, 周昱, 马晓栋. 玻色-爱因斯坦凝聚均匀系统中集体激发的朗道阻尼[J]. 光学学报, 2022, 42(16): 1627001.
[13] Helm J L, Billam T P, Gardiner S A. Bright matter-wave soliton collisions at narrow barriers[J]. Physical Review A, 2012, 85(5): 053621.
[14] Helm J L, Rooney S J, Weiss C, et al. Splitting bright matter-wave solitons on narrow potential barriers: quantum to classical transition and applications to interferometry[J]. Physical Review A, 2014, 89(3): 033610.
[15] Helm J L, Cornish S L, Gardiner S A. Sagnac interferometry using bright matter-wave solitons[J]. Physical Review Letters, 2015, 114(13): 134101.
[16] Polo J, Ahufinger V. Soliton-based matter-wave interferometer[J]. Physical Review A, 2013, 88(5): 053628.
[17] Li S C, Fu L B, Duan W S, et al. Nonlinear Ramsey interferometry with Rosen-Zener pulses on a two-component Bose-Einstein condensate[J]. Physical Review A, 2008, 78(6): 063621.
[18] He Z M, Wang D L, Ding J W, et al. Effect of interspecies interactions on the collision properties of bright-bright solitons in two-species Bose-Einstein condensates[J]. The European Physical Journal D, 2012, 66(5): 139.
[19] Gertjerenken B, Billam T P, Blackley C L, et al. Generating mesoscopic bell states via collisions of distinguishable quantum bright solitons[J]. Physical Review Letters, 2013, 111(10): 100406.
[20] 文林, 梁毅, 周晶, 等. 线性塞曼劈裂对自旋-轨道耦合玻色-爱因斯坦凝聚体中亮孤子动力学的影响[J]. 物理学报, 2019, 68(8): 080301.
Wen L, Liang Y, Zhou J, et al. Effects of linear Zeeman splitting on the dynamics of bright solitons in spin-orbit coupled Bose-Einstein condensates[J]. Acta Physica Sinica, 2019, 68(8): 080301.
[21] Dong G J, Hu B, Lu W P. Ground-state properties of a Bose-Einstein condensate tuned by a far-off-resonant optical field[J]. Physical Review A, 2006, 74(6): 063601.
[22] Dong G J, Hu B. Management of Bose-Einstein condensates by a spatially periodic modulation of the atomic s-wave scattering length[J]. Physical Review A, 2007, 75(1): 013625.
[23] Mayteevarunyoo T, Malomed B A, Dong G J. Spontaneous symmetry breaking in a nonlinear double-well structure[J]. Physical Review A, 2008, 78(5): 053601.
[24] Di Carli A, Henderson G, Flannigan S, et al. Collisionally inhomogeneous Bose-Einstein condensates with a linear interaction gradient[J]. Physical Review Letters, 2020, 125(18): 183602.
[25] Tian Q, Wu L, Zhang J F, et al. Exact soliton solutions and their stability control in the nonlinear Schrödinger equation with spatiotemporally modulated nonlinearity[J]. Physical Review E, 2011, 83(1): 016602.
[26] Wang D S, Hu X H, Hu J P, et al. Quantized quasi-two-dimensional Bose-Einstein condensates with spatially modulated nonlinearity[J]. Physical Review A, 2010, 81(2): 025604.
[27] Wang D S, Hu X H, Liu W M. Localized nonlinear matter waves in two-component Bose-Einstein condensates with time- and space-modulated nonlinearities[J]. Physical Review A, 2010, 82(2): 023612.
[28] Gligorić G, Maluckov A, Hadžievski L, et al. Discrete localized modes supported by an inhomogeneous defocusing nonlinearity[J]. Physical Review E, 2013, 88(3): 032905.
[29] Balaž A, Paun R, Nicolin A I, et al. Faraday waves in collisionally inhomogeneous Bose-Einstein condensates[J]. Physical Review A, 2014, 89(2): 023609.
[30] Sudharsan J B, Radha R, Fabrelli H, et al. Stable multiple vortices in collisionally inhomogeneous attractive Bose-Einstein condensate[J]. Physical Review A, 2015, 92(5): 053601.
Article Outline
何章明, 朱钱泉, 潘湘. 排斥抛物势中相互作用时空调控的超冷玻色气体孤子的反射、局域、穿越与振荡行为操控[J]. 光学学报, 2023, 43(13): 1327001. Zhangming He, Qianquan Zhu, Xiang Pan. Reflection, Localization, Transmission, and Oscillation Behaviors of Solitons in Ultracold Bose Gases with Spatiotemporally Modulated Interactions in an Expulsive Parabolic Potential[J]. Acta Optica Sinica, 2023, 43(13): 1327001.