非偏振分光棱镜的偏振敏感度测量
下载: 504次
A non-polarizing beam splitter (NPBS) is commonly used in interferometer systems. Ideally, the NPBS can ensure consistency between the polarization state of the outgoing light and that of the incident light. However, because of defects in the actual process, the birefringence effect of NPBS has a non-negligible impact on the nonlinear error, polarization error, and measurement accuracy of the interference system. Therefore, it is necessary to measure the polarization sensitivity of an NPBS to compensate for the birefringence error. At present, the general system structure used to measure polarization sensitivity is relatively complex, and some methods require a combination of spectrometers or modulators and additional circuitry for measurement, which is cumbersome with respect to data processing and inconvenient to operate. In this paper, we propose a different method to measure the polarization sensitivity of NPBS, which has the advantages of fast measurement speed, simple system structure, efficient data processing, high repeatability, and can eliminate the influence of light source jitter, optical path system error, and PD responsivity inconsistency. This method is significant for evaluating the NPBS error to provide high-quality NPBSs for use in interferometer systems.
NPBS polarization sensitivity measurements include transmittance/reflectance measurement and transmission/reflection phase difference measurement. First, the measurement principles of the four characteristic parameters affecting the polarization sensitivity of NPBS are introduced. Regarding transmittance/reflectance measurements, circularly polarized light is incident to the NPBS, and the intensities of the s- and p-polarization directions in the transmitted/reflected light are measured synchronously. The voltage values corresponding to the light intensity of s light and p light are obtained by photodetector. The measurement results are then normalized to suppress the influence of light source jitter, optical path system error, and PD response inconsistency on the measurement results. Regarding the phase polarization sensitivity measurement, we use a polarization measurement system (PSGA) to measure the S2 and S3 Stokes components of the transmitted/reflected light. Using the Stokes parameter method to calculate the phase difference can eliminate the effect of light intensity jitter. The relative values of the transmission/reflection phase difference of the s light and p light of the NPBS are then obtained by the difference method to eliminate the influence of the optical path system error.
The accuracy of the proposed NPBS polarization sensitivity measurement method was verified experimentally. In terms of the verification of the transmittance/reflectance polarization sensitivity measurement system, the blank transmittance in air without NPBS was measured, and the transmittance after normalization correction was 1.0001, indicating that the transmittance/reflectance polarization sensitivity measurement of the system has good accuracy (error of 0.01%). This is an improvement compared to the result before normalization correction (3.32%) and clearly demonstrates the error correction effect obtained by normalization correction (Fig. 4). To verify the transmission/reflection phase difference polarization sensitivity measurement system, the measurement system was used to measure the phase retardation of the quarter-wave plate, and the measurement result deviated by 0.09° from the theoretical value of 90°, indicating the high measurement accuracy of the measurement system (Fig. 4). The polarization sensitivities of three customized NPBS samples were measured ten times repeatedly using the proposed measurement method. The measurement accuracy of the NPBS transmittance/reflectance is -0.08%-+0.08%, and the repeatability is better than 0.1%. The measurement accuracy of the NPBS transmission/reflection phase difference is -0.84%-+0.84%, and the repeatability is better than 1% (Fig. 5). These results demonstrate that the proposed measurement method has the advantages of good measurement stability and high measurement accuracy. Furthermore, the polarization sensitivities of the NPBS samples were measured at different incident wavelengths and incident angles. Within the range of 1540 nm-1560 nm, the change in the transmittance/reflectance was less than 0.02, and the transmission/reflection phase difference between s light and p light decreased with increasing wavelength (Fig. 6). When the incident yaw angle changes from -5° to +5°, the phase difference between s light and p light decreases (Fig. 7). The change in the NPBS reflection phase difference is larger than that in the NPBS transmission phase difference, indicating that the former is more sensitive to wavelength and angle changes.
In this paper, a method for measuring the polarization sensitivity of NPBS is proposed. The transmittance and reflectance are measured by simultaneously measuring the intensities of the transmitted/reflected circularly polarized light in the s- and p-polarization directions. The light source jitter, optical path system error, and error caused by inconsistencies in the detector response are eliminated through normalization correction. By using a polarization measurement system to measure the S2 and S3 Stokes components of the transmitted/reflected light, the s light and p light transmission/reflection phase difference of the NPBS can be obtained. Based on the above method, the measurement accuracy for transmittance and reflectance is -0.08%-+0.08% and the repeatability is better than 0.1%. The measurement accuracy of the transmission phase difference and reflection phase difference is -0.84%-+0.84%, and the repeatability is better than 1%. The proposed NPBS polarization sensitivity characteristic measurement method has the advantages of simple system structure, efficient data processing, high repeatability, and can eliminate the influence of light source jitter, optical path system error, and PD responsivity inconsistency. The method is significant for analyzing and compensating for the errors caused by an NPBS to obtain high-quality interferometer systems.
1 引言
非偏振分光棱镜(NPBS)是干涉仪中常用的光学器件,具有偏振态的分离度低、应力小、保偏能力强、成本低等显著优点,已被广泛应用于各种干涉仪中[1-4]。理想情况下的NPBS保持出射光的偏振态与入射光一致,即s光、p光分量的透反射比相同,相位差的变化量为0°,但实际的加工工艺会导致NPBS具有一定的双折射效应,使得出射光的偏振态发生变化。NPBS引入的附加相移以及s、p分量之间的分光比误差会增大干涉仪系统的非正交相移误差[5]。NPBS偏振敏感度不仅会导致外差干涉仪产生非线性误差,还会影响椭偏仪的测量精度[6-7]。因此,NPBS对干涉仪系统误差的影响不容忽视,需要精确测量其自身产生的s光、p光分量的强度变化和相位差变化,以便在使用时能够采取相应的补偿方法消除NPBS对干涉系统造成的影响。
NPBS透/反射率偏振敏感度一般采用起偏/检偏的方法进行测量[8]。在测量过程中,如何消除光源抖动、探测器偏振响应不一致性等影响因素是保证测量精度必须解决的问题。NPBS透/反射相位差偏振敏感度的测量可以借鉴波片相位延迟测量方法,主要有迈克耳孙干涉法[9]、弹光调制法[10-13]、穆勒矩阵法[14]、光强调制法和椭圆偏振法等[15-17],但这些测量方法所使用的系统一般比较复杂,往往需要用到多个光学器件,有的还需要结合光谱仪(或调制器)和电路结构进行测量,且数据处理繁琐,操作不便。另外,如何消除光源抖动以及光路系统、PD偏振响应不一致性对测量结果的影响,也是保证测量结果精度必须要考虑的主要问题。
本课题组提出了一种NPBS偏振敏感度测量技术,并搭建了一套测量NPBS偏振敏感度的实验系统,采用该系统对通过NPBS的s、p偏振光之间的透射比、反射比、透射相位差和反射相位差进行测量,并进行了不同波长和不同入射角度下的NPBS偏振敏感度测量实验。本文提出的NPBS偏振敏感度测试方法具有测量速度快、系统结构简单、数据处理方便、重复性高等优点,而且能够消除光源抖动、光路系统误差和PD响应度不一致性的影响,在评估NPBS对干涉仪系统造成的误差以及选择高质量NPBS等方面具有重要意义。
2 测量原理
NPBS的结构如
式中:
采用圆偏振光对NPBS的透/反射比偏振敏感度进行测量。圆偏振光的两个正交偏振分量的振幅大小相同,经过NPBS的透/反射光通过PBS偏振分光后进行强度测量。利用两个光电探测器(PD)同时进行测量,得到s光、p光光强对应的电压值,最后经过数据处理便可得到透/反射比偏振敏感度。然而,在实际测量光路中,光源抖动、光路系统误差以及两个光电探测器的响应不一致性均会影响NPBS透/反射比(
在NPBS偏振相位敏感度测量方面,通常采用斯托克斯参量来计算透射相位差和反射相位差。如
式中:
式中:S0、S1、S2、S3为4个斯托克斯分量;
当一束45°线偏振光入射NPBS后,进入偏振测量系统的光的4个斯托克斯参量归一化后为:S0=1,S1=0,S2=1,S3=0。入射NPBS的光的s分量与p光分量之间的相位差
式中:
由
3 测量方案及实验结果
NPBS的透射比和反射比测试系统如
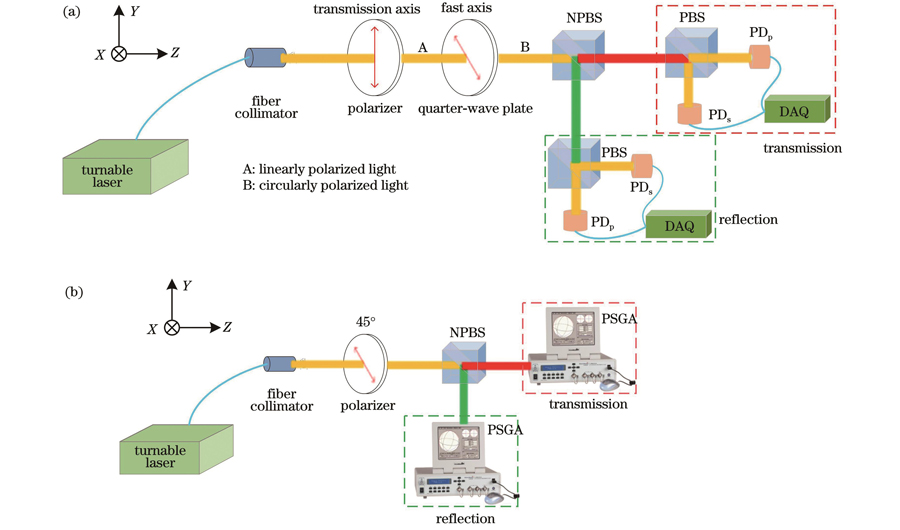
图 2. NPBS偏振敏感度测量系统。(a)NPBS透/反射比偏振敏感度测量系统;(b)NPBS透/反射相位差偏振敏感度测量系统
Fig. 2. NPBS polarization sensitivity measurement system. (a) NPBS transmittance/reflectance polarization sensitivity measurement system; (b) NPBS transmission/reflection phase difference polarization sensitivity measurement system
对于NPBS的透/反射比偏振敏感度测量系统来说,会对测量结果产生影响的主要误差包括光源抖动、入射NPBS的光为非理想圆偏振光、PBS对p光和s光的透/反射率不同等。当起偏器透射轴和1/4波片快轴的夹角与45°存在偏差,以及1/4波片的相位延迟量与90°存在偏差时,将导致入射至被测NPBS的光为非理想圆偏振光,由此对s光和p光光强产生的误差系数分别为
式中:
考虑到光电探测器的光电响应偏振敏感度以及起偏器和PBS的消光比、光电探测器暗电流以及电压信号处理电路引入的偏置量会对系统造成影响,将光路中未加入NPBS时s光、p光对应的两个光电探测器的输出电压
式中:
式中:
NPBS的透射相位差和反射相位差测试系统如
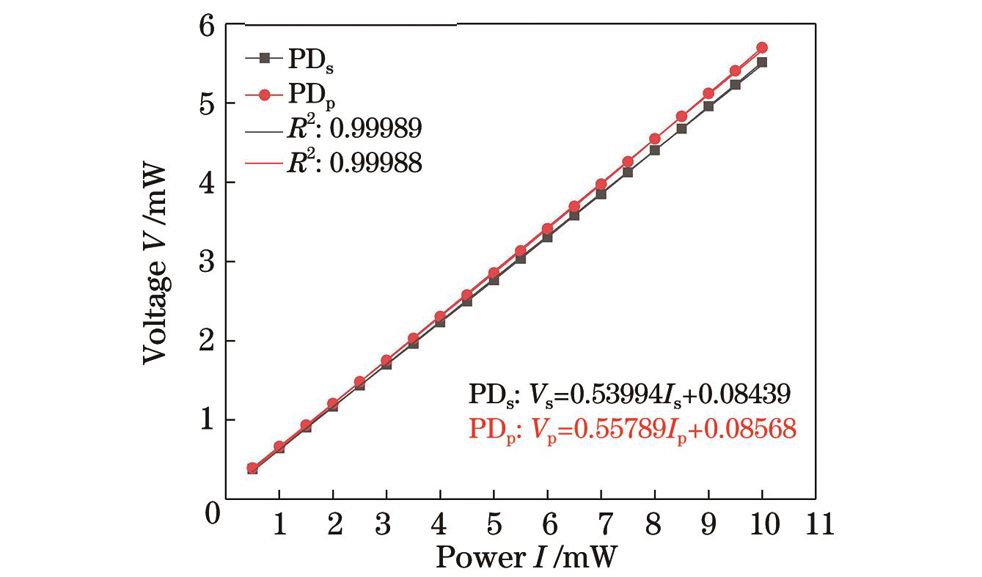
图 3. 实验测量系统中PDs和PDp的归一化校正拟合曲线图
Fig. 3. PDs and PDp normalized calibration fitting curves in experimental measurement system
接下来对NPBS偏振敏感度测量系统的测量准确性进行实验验证。首先进行透/反射比偏振敏感度测量准确度的实验验证。对未放入NPBS的空气空白进行p光、s光透/反射比测量,验证系统的测量准确度。由理论分析可知,理想情况下未放入NPBS时,s光、p光的透射比为1。对空气空白进行10次重复测量,透射比测量结果如
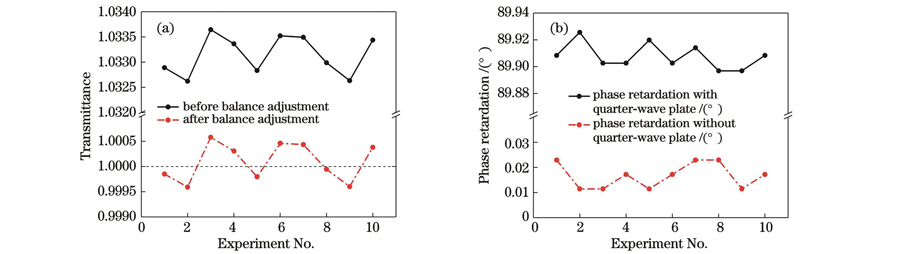
图 4. NPBS偏振敏感度测量系统准确度验证实验。(a)NPBS透/反射比偏振敏感度测量系统准确度验证实验测试结果;(b)NPBS透/反射相位差偏振敏感度测量系统准确度验证实验测试结果
Fig. 4. Accuracy verification experiment of NPBS polarization sensitivity measurement system. (a) Test results of NPBS transmittance/reflectance polarization sensitivity measurement system accuracy verification experiment; (b) test results of NPBS transmission/reflection phase difference polarization sensitivity measurement system accuracy verification experiment
接着进行透/反射相位差偏振敏感度测量准确度的实验验证。光路中不放入NPBS,对空气空白进行测量,并使用该系统对Thorlabs公司的1/4波片(WPQ05ME-1550 nm)进行测量,测量结果如
随后,利用上述实验系统对3个定制加工的宽带NPBS(分光比为50∶50)进行测量实验,每个NPBS的透射比和反射比、透射相位差和反射相位差均重复测量10次。3个NPBS样品的透/反射比和透/反射相位差的10次测试结果分别如
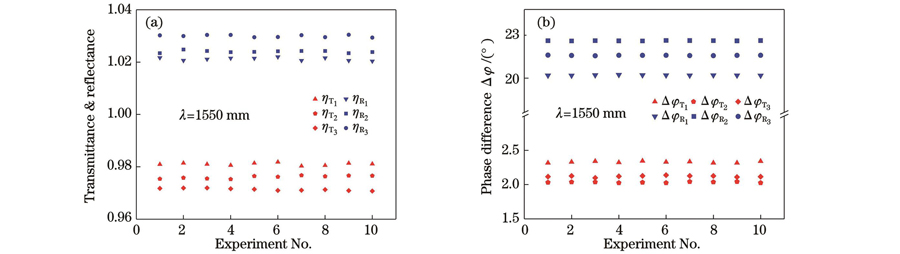
图 5. 3个NPBS样品透/反射比、透/反射相位差的10次重复测量结果。(a)透/反射比的10次重复测量结果;(b)透/反射相位差的10次重复测量结果
Fig. 5. Ten repeatable measurements of transmittance/reflectance and transmission/reflection phase difference of three NPBS samples. (a) Ten repeatable measurements of transmittance/reflectance; (b) ten repeatable measurements of transmission/reflection phase difference
此外,本课题组对NPBS在不同入射波长(1540~1560 nm)下的偏振敏感度进行了测试,以研究NPBS在不同波长下透/反射比和透/反射相位差的变化。实验过程中,每改变一次波长就对测试系统进行一次归一化校正,以消除波长变化对测量系统的影响。实验结果如

图 6. 不同入射波长下NPBS样品偏振敏感度测量实验结果。(a)NPBS透/反射比随入射波长的变化;(b)NPBS透/反射相位差随入射波长的变化
Fig. 6. Experimental results of polarization sensitivity measurement of NPBS samples at different incident wavelengths. (a) Variation of NPBS transmittance/reflectance with incident wavelength; (b) variation of NPBS transmission/reflection phase difference with incident wavelength
本课题组还对不同入射角度(偏摆角度为-5°~+5°)下的NPBS偏振敏感度进行了测试,以探究NPBS在不同入射角度情况下的透/反射比和透/反射相位差的变化,实验结果如
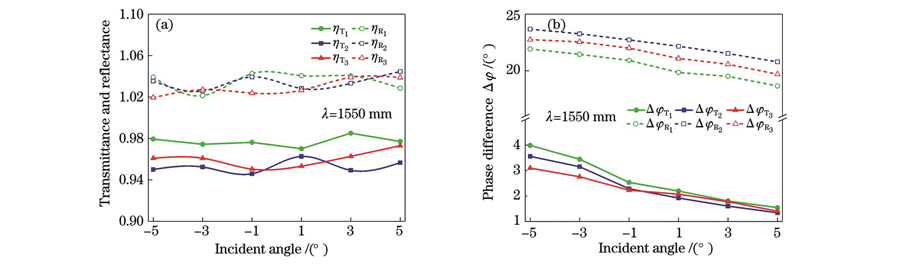
图 7. 不同入射角度下NPBS样品偏振敏感度测量实验结果。(a)NPBS透/反射比随入射角度的变化;(b)NPBS透/反射相位差随入射角度的变化
Fig. 7. Experimental results of polarization sensitivity measurement of NPBS samples at different incident angles. (a) Variation of NPBS transmittance/reflectance with incident angle; (b) variation of NPBS transmission/reflection phase difference with incident angle
4 结论
针对NPBS的偏振敏感度特性,本文提出了一种测量NPBS透/反射比和透/反射相位差的方法,用透射比、反射比、透射相位差和反射相位差共同表征偏振敏感度。通过同步测量透/反射圆偏振光s、p偏振方向的强度来测量NPBS的s光分量与p光分量的透射比、反射比,并通过归一化校正消除了光源抖动、光路系统误差和光电探测器响应不一致性带来的误差。采用偏振测量系统测量透/反射光的斯托克斯分量S2和S3,得出NPBS的透/反射相位差,然后用斯托克斯参量法计算相位差,消除了光强抖动带来的影响。实验结果表明,基于上述方法实现了NPBS的透/反射比测量精度为-0.08%~+0.08%,重复性优于0.1%,NPBS的透/反射相位差测量精度为-0.84%~+0.84%,重复性优于1%。
所提NPBS偏振敏感度特性测试方法具有系统结构简单、数据处理方便、重复性高等优点,而且能够消除光源抖动、光路系统误差和光电探测器响应不一致性的影响。NPBS引入的附加相移和透/反射比对干涉仪测量系统的非线性误差、偏振误差和测量精度等具有不可忽视的影响。所提测量方法在选择高质量NPBS以及分析NPBS对干涉仪系统造成的误差并对误差进行补偿等方面具有重要意义。
[1] 张博, 严利平, 刁晓飞. 波片阵列对准误差对激光干涉非线性误差的影响[J]. 中国激光, 2022, 49(9): 0904003.
[2] 张鹏, 崔建军. 外差干涉仪非线性误差补偿抑制与测量研究进展[J]. 激光与光电子学进展, 2021, 58(11): 1100003.
Zhang P, Cui J J. Research progress in nonlinear error compensation suppression and measurement of heterodyne interferometer[J]. Laser&Optoelectronics Progress, 2021, 58(11): 1100003.
[3] 于海娇, 赵国罡. 基于振动节点优化提高双频激光干涉仪的测量精度[J]. 激光与光电子学进展, 2020, 57(15): 151202.
Yu H J, Zhao G G. Improving measurement accuracy of dual-frequency laser interferometer based on vibration node optimization[J]. Laser&Optoelectronics Progress, 2020, 57(15): 151202.
[4] Zhao S J, Wei H Y, Zhu M H, et al. Green laser interferometric metrology system with sub-nanometer periodic nonlinearity[J]. Applied Optics, 2016, 55(11): 3006-3011.
[5] 崔俊宁, 李伟, 边星元, 等. 面向大振幅、长周期振动校准的零差正交激光干涉测振方法[J]. 红外与激光工程, 2021, 50(6): 20200329.
[6] Deng Y L, Li X J, Geng Y F, et al. Effect of nonpolarizing beam splitter on measurement error in heterodyne interferometric ellipsometers[J]. Measurement Science and Technology, 2012, 23(8): 085204.
[7] 邓元龙, 李学金, 耿优福, 等. 非偏振分光镜对外差干涉仪非线性误差的影响[J]. 光学学报, 2012, 32(11): 1112008.
[8] 王政平, 张尚婷, 郝蕴绮. NPBS参量温度特性及其对OCRCS影响的理论研究[J]. 哈尔滨工程大学学报, 2008, 29(1): 98-101.
Wang Z P, Zhang S T, Hao Y Q. Theoretical investigation on temperature features of NPBS parameters and their influences on OCRCS[J]. Journal of Harbin Engineering University, 2008, 29(1): 98-101.
[9] 王军, 陈磊, 吴泉英, 等. 一种基于白光迈克耳孙干涉仪波片延迟量的测量方法[J]. 中国激光, 2011, 38(5): 0508001.
[10] Li K W, Zhang R, Jing N, et al. Fast and full range measurements of ellipsometric parameters using a 45° dual-drive symmetric photoelastic modulator[J]. Optics Express, 2017, 25(5): 5725-5733.
[11] FitzGerald W R, Hore D K. Photoelastic modulator-based broadband mid-infrared stokes polarimeter[J]. Journal of Modern Optics, 2018, 65(1): 75-84.
[12] 王金伟, 李克武, 景宁, 等. 波片相位延迟量测量和快轴标定系统[J]. 红外与激光工程, 2019, 48(2): 0217002.
[13] 张瑞, 陈媛媛, 景宁, 等. 基于双弹光差频调制的中红外波片相位延迟高精度测量[J]. 光学学报, 2019, 39(3): 0312002.
[14] 黄书强, 江秀美, 冯元华. 光谱编码型穆勒矩阵测量中的相位延迟量误差分析[J]. 激光与光电子学进展, 2021, 58(19): 1926001.
Huang S Q, Jiang X M, Feng Y H. Analysis of phase error of retarders in spectrally encoded Mueller matrix measurement[J]. Laser&Optoelectronics Progress, 2021, 58(19): 1926001.
[15] 张志勇, 邓元勇, 王东光, 等. 几种波片位相延迟测量方法的比较[J]. 光学 精密工程, 2007, 15(11): 1678-1685.
[16] 刘维新, 魏志伟, 赵文谦, 等. 光学波片相位延迟测量仪设计[J]. 红外与激光工程, 2019, 48(7): 0718001.
[17] 袁凯华, 邓剑勋, 刘超, 等. 一种快速同时测量波片相位延迟量和快轴方位角的方法[J]. 激光与光电子学进展, 2018, 55(2): 021202.
Yuan K H, Deng J X, Liu C, et al. A fast and simultaneous method for measuring wave plate phase retardation and fast axis azimuth[J]. Laser&Optoelectronics Progress, 2018, 55(2): 021202.
[18] 郭忠义, 康乾龙, 彭志勇, 等. 基于超表面操控的偏振信息探测技术研究进展[J]. 红外与激光工程, 2020, 49(9): 20201041.
[19] 王政平, 阮顺龄, 孙伟民, 等. 单层介质膜反射棱镜式光学相位延迟器件研究[J]. 光子学报, 1997, 26(6): 555-560.
Wang Z P, Ruan S L, Sun W M, et al. Reflecting prism-retarders with a single medium layer[J]. Acta Photonica Sinica, 1997, 26(6): 555-560.
Article Outline
孟晨, 高珊, 姚晓天, 崔省伟, 郝鹏. 非偏振分光棱镜的偏振敏感度测量[J]. 中国激光, 2023, 50(5): 0504004. Chen Meng, Shan Gao, X. Steve Yao, Shengwei Cui, Peng Hao. Polarization Sensitivity Measurement Method for Non-Polarizing Beam Splitters[J]. Chinese Journal of Lasers, 2023, 50(5): 0504004.