探测气溶胶-水汽的拉曼-米散射激光雷达系统
0 引 言
随着社会经济的不断发展,环境问题日益严重,获得大气参数(如气溶胶、水汽、温度等)越来越受到环保和气象部门的重视。开展大气气溶胶和水汽的连续探测研究,可以提高天气预报的准确性,对研究大气污染气溶胶特性及扩散机理、云的形成、降雨等具有重要的科学意义。激光雷达作为一种主动遥感探测工具,具备传统大气探测方法无法比拟的时空分辨率及连续性,在大气参数测量中发挥着重要作用[1-4]。
2004年,谢晨波等[5]研制的国内首台车载式大气探测激光雷达系统,用于探测大气气溶胶和水汽,出射波长为355 nm (300 mJ)和532 nm (150 mJ)。2010年, 吕炜煜等[6]研制的车载激光雷达系统,用于探测对流层气溶胶和水汽,出射波长为355 nm(400 mJ)和1064 nm(400 mJ)。2014年,谭敏等[7-8]研制的温度、水汽和气溶胶拉曼激光雷达,用于探测大气温度、水汽和气溶胶,出射波长分别为355 nm (500 mJ)、532 nm (200 mJ)和1064 nm (300 mJ)。通过对以上激光雷达系统的分析可知,利用出射波长355 nm的氮气拉曼散射回波信号和水汽拉曼散射回波信号探测水汽混合比,能量在300 mJ以上;355、532、1064 nm的米散射用于探测大气气溶胶,能量在150 mJ以上。这种采用大能量的出射波长,可以有效提高白天的信噪比,实现水汽的白天探测,但所设计的激光雷达系统具有成本高、尺寸大、结构复杂等缺点,很难实现其市场化。
随着激光雷达技术的不断成熟,激光雷达向着小型化、产品化及简单化方向发展,为了满足国家环保部门及时了解大气参数的测量要求,中国科学院安徽光学精密机械研究所中国科学院大气光学重点实验室研制了一套户外型全天时大气气溶胶-水汽激光雷达产品,用于气溶胶及水汽的长期探测。
1 探测原理
大气气溶胶-水汽激光雷达中三波长米散射激光雷达方程为:
式中:
反演大气气溶胶消光系数采用Fernald方法,该方法在反演过程中需要假设激光雷达比Sa(气溶胶消光后向散射比),受到气溶胶特性和波长的影响。文中假定激光雷达比Sa为常数,取532 nm和355 nm的Sa为50 sr。空气分子激光雷达比Sm(空气分子消光后向散射比)由大气模式获得,为
在利用Fernald方法反演的过程中,需要选取不含气溶胶粒子的洁净大气的高度作为标定高度rc,取
其中,
式中:
混合层高度是指大气气溶胶浓度梯度变化最大的高度,记为
Angstrom指数A是度量气溶胶粒子尺度的一个重要参数,其表达式为:
当细粒子模态(50~500 nm)和粗粒子模态(大于500 nm)共同影响光散射时,利用两个波长指数可以更深入地研究气溶胶粒子的半径[10]。文中研制的雷达系统有355 nm和532 nm两种波长,利用A532/355分析不同天气下的气溶胶粒子特性。
偏振激光雷达的偏振特性可用于研究气溶胶粒子的形态特征,是探测冰晶云和沙尘气溶胶等非球形粒子的最有效的方法,文中系统设计的波长532 nm的退偏振比可以表示为:
式中:
水汽拉曼激光雷达探测的水汽混合比(g/kg)可表达为:
式中:
2 激光雷达系统
为了满足在户外长期探测大气气溶胶-水汽的时空变化,设计及构建了一台户外型全天时激光雷达系统,该系统示意图及外观如图1所示,其外形尺寸为1.8 m×1.2 m×2 m (长×宽×高);表1为该系统主要性能参数,其主要由发射单元、接收单元、后继单元、数据采集单元及辅助控制单元组成。
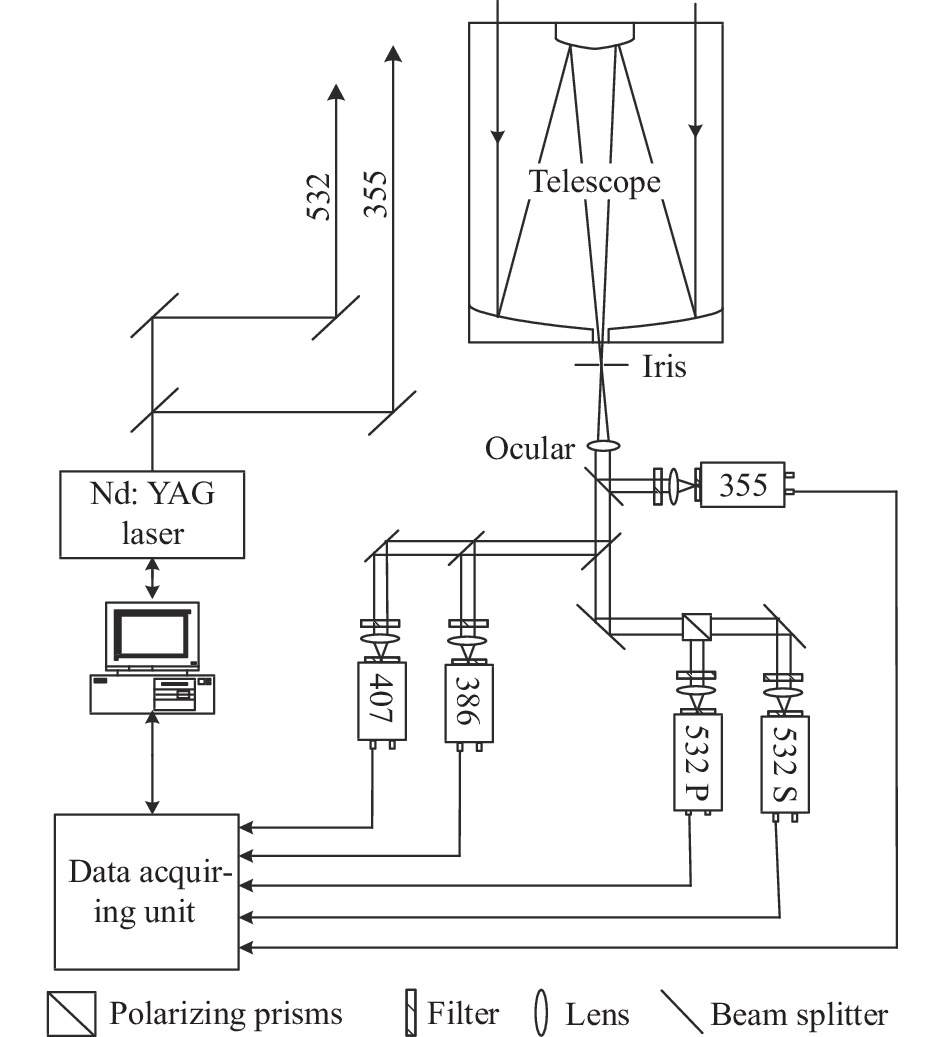
图 1. 大气气溶胶-水汽激光雷达产品结构示意图
Fig. 1. Structural schematic of lidar system for atmospheric aerosol-water vapor
表 1. 大气气溶胶-水汽激光雷达系统的主要参数、性能指标
Table 1. Main specifications, performance parameters of lidar system for atmospheric aerosol-water vapor
|
发射单元中,激光器采用Nd:YAG激光器,输出波长为二倍频532 nm和三倍频355 nm,其能量分别为88 mJ和50 mJ,重复频率均为10 Hz。由于两波长从一个出光口射出,故采用高稳定的光学调整架及分束镜将两波长分离开,再利用反射镜和高稳定光学调整架将两波长反射到大气中,来调节发射光轴与接收光轴并保持高度的平行。由于出射光束小于13 mm,故所有的发射单元镜片采用1 in (1 in=2.54 cm)的圆形镜片。
接收单元主要由望远镜、小孔光阑及目镜组成。望远镜采用卡塞格林望远镜,有效口径为400 mm,并在其系统焦点处安装具有可调节的小孔光阑,来实现在0.5~2 mrad范围内调节,适用于不同环境和弱信号探测的要求;通过小孔光阑的汇聚光经过焦距为100 mm的目镜将其准直为准平行光用于后继光学单元探测。
后继光学单元光路设计优化是提高探测灵敏度的关键技术之一,其稳定性直接关系到探测信号是否完全被探测器所接收。首先对后继单元设计进行优化的原则:弱信号光程要短;分束片使用高性能的镀膜,增加反射和透射效率;通过分束片尽可能采用反射方式等,设计出的后继光路如图1所示;然后对后继单元进行结构设计,将所有分束片采用矩形,安装结构采用三角结构,基频远大于200 Hz,结构稳定性高,如图2(a)所示,并通过高精度的插槽方式固定于一体化的后继光学结构中,如图2(b)所示,其基频远大于200 Hz,稳定性高。
后继探测单元由5个探测通道组成,其中3个米散射探测通道分别用于探测355 nm出射波长与气溶胶粒子相互作用产生的米散射信号及532 nm的米散射型号垂直分量和水平分量,2个拉曼探测通道分别用于355 nm出射波长与氮气分子相互作用产生的386 nm拉曼散射信号和与水汽分子相互作用产生的407 nm拉曼信号。均采用探测器PMT,在每个探测器前安装1 nm的干涉滤光片来滤除背景光;以及安装会聚透镜,安装完成如图3所示。
数据采集单元主要用于采集探测器转换的电信号,其采集系统采用模数转换A/D (采样精度为12 bits)和光子技术PC (最高计数率为250 MHz)相结合的数据采集器,空间分辨率为7.5 m。
系统的辅助控制单元主要有以下几种功能:
(1)利用工控机来确保发射信号与数据采集同步进行;
(2)利用全自动反演软件,无需人为干预便可获得反演后的数据,如不同波长的距离订正回波信号、退偏振比以及水汽等数据获取,可快速直观地描述大气垂直分布的时空变化特征,同时,与自动测量程序相结合,可实现无人干预条件下的自动测量和数据反演;
(3)利用设计控制软件可实现对雷达系统方舱进行温度、湿度的自动控制、一键启动、远程数据传输及应急保护等功能。
3 探测结果与数据分析
3.1 大气气溶胶探测结果分析
2019年年初,合肥出现了严重的污染天,运用拉曼-米散射激光雷达对2019年1月份合肥地区上空大气进行了探测,一次探测累积时间为15 min。
3.1.1 污染天探测结果与分析
测量时间为2019年1月19日23:00,根据气象数据可知,此时AQI为237,PM2.5为187 μg/m3,为重度污染天。图4为实际探测的355 nm和532 nm (P和S)波长的距离平方信号(RSCS)(实线),及其导数(虚线),两波长回波信号随高度变化趋势相同。图5为根据图4的数据反演出的消光系数、Angstrom指数特性及532 nm的退偏振比。由于当时天气为重度污染,且出现低层薄云,影响了系统探测距离。由图4、图5可以明显看出,分别在高度1.75 km、3 km以及3.6 km处出现薄云。
由图4可知,重度污染天的混合层高度很低,对两波长回波信号进行一阶求导,计算出混合层高度分别为0.47 km (355 nm),0.36 km (532 nm),这是由于两波长所受气溶胶分布特征影响不同,从而导致两波长计算处理的混合层高度不同。
由图5可知,重度污染天消光系数较大,两波长的消光曲线具有良好的一致性。利用消光系数计算出的Angstrom指数在−2.5~3.8之间变化,退偏振比在0~0.11之间变化。1.25 km以下的污染层:Angstrom指数在0.8~1.8之间变化,说明在底层气溶胶存在较多的直径为1 μm的粗粒子,且在0.5~1.25 km基本保持不变,说明该区域气溶胶粒子均匀混合。退偏振比在0.03~0.11之间变化,随着高度不断增加而减小,但在0.7~1.25 km发生突变,峰值达到0.07,整个污染层主要以球形粒子污染物为主。在高度1.75 km、3 km以及3.6 km处云层中,两波长消光系数进入云层后数值大小发生反转,对应计算出的Angstrom指数出现较大的波动,并出现负值,说明在云层中粒子半径较大;对应的退偏振比都在0.05以下,属于水云。

图 5. 重度污染天的消光系数(a)、Angstrom指数(b)及退偏振比(c)
Fig. 5. Extinction coefficient (a), Angstrom index (b) and depolarization ratio (c) in the heavy pollution conditions
3.1.2 干净天探测结果与分析
测量时间为2019年1月26日13:00,根据气象数据可知,此时AQI为24,PM2.5为14 μg/m3,为一级优天。利用激光雷达系统探测到的RSCS及其导数,如图6所示。根据探测数据反演得到消光系数、Angstrom指数特性及532 nm的退偏振比,如图7所示。由图6、图7可知,在8 km处出现卷云。
由图6可知,355 nm和532 nm信号得到的混合层高度为1.1 km,这是由于在一级优天条件下,在混合层内气溶胶分布特征对两波长影响近似。
由图7可知,在一级优天的条件下,两波长在底层反演得到的消光系数远小于重度污染天的消光系数。根据Angstrom指数曲线可知,底层气溶胶的Angstrom指数比重度污染天要大,说明在一级优天条件下,底层大气中气溶胶粒子半径比重度污染天的小。根据退偏振比曲线可知,底层气溶胶在这两种天气下的退偏振比都在同一范围内(0~0.11),说明两种天气底层气溶胶以球形粒子为主。在1.7 km以下,Angstrom指数随着高度增加而呈现增加的趋势,说明气溶胶粒子半径随高度的增加而呈现减小的趋势。在1.7~3.9 km高度下,Angstrom指数随着高度增加而不断减小,最小值到达0.88,且退偏振比不断增加,最大值达到0.047,说明气溶胶粒子半径随高度的增加而增加,且在3.9 km处气溶胶层以直径为1 μm的粗粒子的球形粗粒子为主。在8 km处,卷云的两波长消光系数数值发生反转;对应的Angstrom指数波动较大,且出现负值,说明在云层中粒子半径较大;对应的退偏振比峰值到达0.2,介于水云和冰晶云之间,属于混合云。
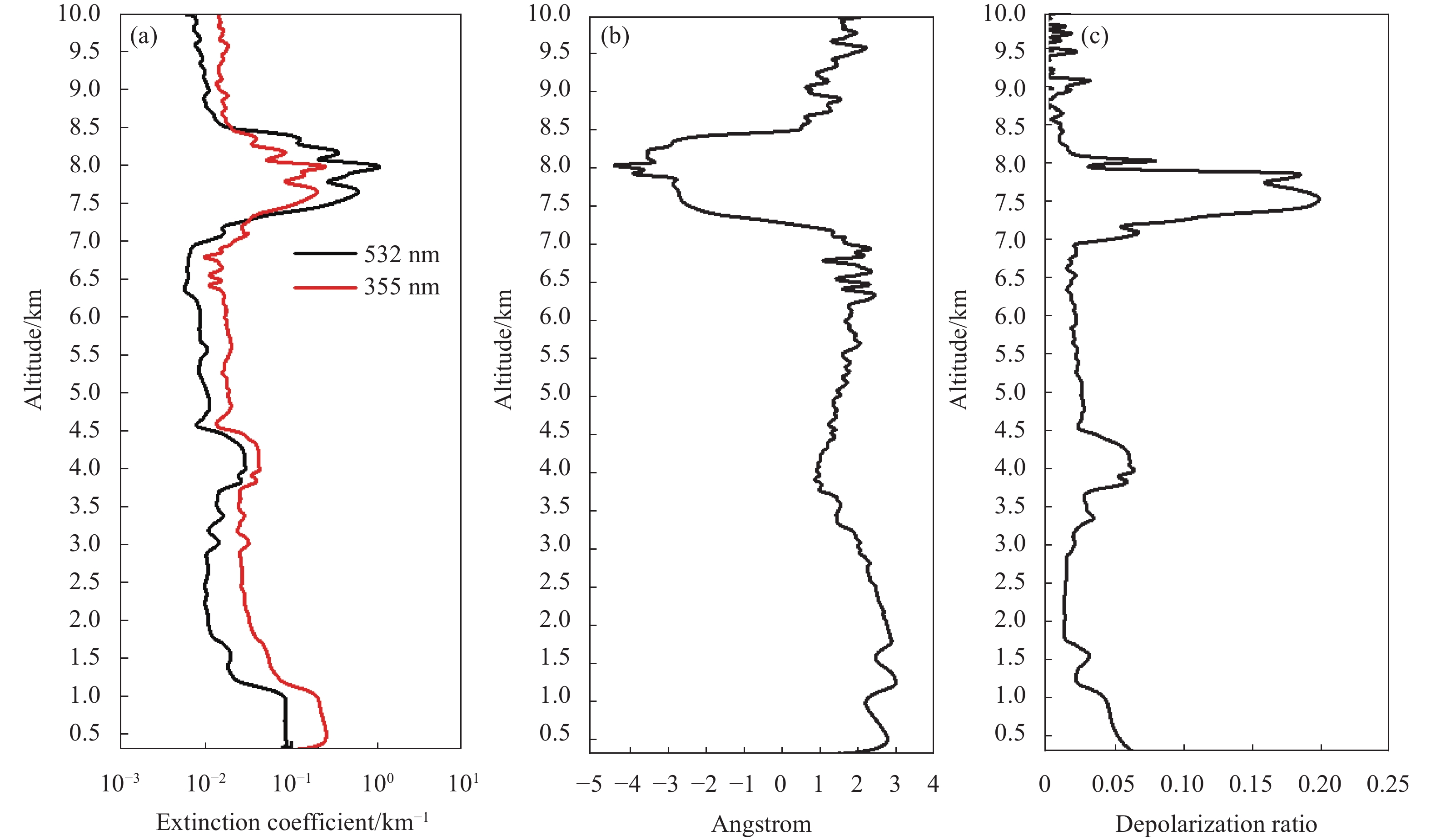
图 7. 干净天的消光系数(a)、Angstrom指数(b)及退偏振比(c)
Fig. 7. Extinction coefficient (a), Angstrom index (b) and depolarization ratio (c) in the clean weather conditions
3.2 系统常数标定及水汽探测结果分析
3.2.1 系统常数标定
采用文献[15]研制的全固态紫外拉曼激光雷达系统探测的水汽混合比对文中研制的激光雷达进行标定,获得标定常数
式中:
利用全固态紫外拉曼激光雷达系统对文中所设计的拉曼-米激光雷达进行系统标定,标定时间为2020年11月20日01:45,根据气象数据可知,此时AQI为64,PM2.5为49 μg/m3,该天空气质量良好。由文献[15]提供的水汽混合比垂直分布如图8(a)(实线)所示,在5 km左右出现比较厚的云,使得5 km以上的数据迅速减小,甚至出现负值,这是由于信号没有穿透云层所致。综合考虑拉曼-米激光雷达的几何重叠因子的影响,文中选择0.5~3.5 km的水汽混合比对拉曼-米激光雷达系统进行标定,获得系统标定常数为121。

图 8. 水汽混合比垂直分布特征及绝对误差廓线图
Fig. 8. Profile of vertical distribution feature of water vapor mixing ratio and absolute error
拉曼-米激光雷达通过标定常数反演的水汽混合比,如图8(a)(虚线)所示,与全固态紫外拉曼激光雷达反演的水汽混合比,如图8(a)(实线)进行对比,两者探测结果大体一致,水汽结果能较好地吻合。对比两者的绝对误差,如图8(b)所示,绝大部分的绝对误差在±0.3 g/kg以内,但由于几何重叠因子的影响导致在0.5 km 以下误差较大。
3.2.2 水汽探测结果分析
测量时间为2018年11月01日18:00到2018年11月02日16:00,根据气象数据可知,此时AQI平均为60,PM2.5平均为40.5 μg/m3,该天空气质量良好。图9给出了探测期间水汽混合比的连续时空分布,垂直分辨率为7.5 m,时间分辨率为15 min。
由图9可知,夜晚测量探测高度可达5 km,白天由于背景太强,导致水汽混合比探测高度只有1.4~2 km。在夜晚探测期间,在2.5 km左右有明显的水汽层,随着时间的变化,峰值从1.5 g/kg增加到3 g/kg,由于信噪比低,该水汽层在白天探测不到。水汽主要分布在1.5 km以内,夜晚底层水汽混合比大部分在4~5 g/kg之间变化,白天水汽混合比大部分在3~4 g/kg之间变化,且夜晚底层水汽混合比要比白天高。由此表明,拉曼-米激光雷达系统可以实时、连续、精确探测大气中的水汽含量。
4 结 论
研制了一款用于连续探测大气气溶胶和水汽的激光雷达系统。不同于传统的大气气溶胶和水汽激光雷达,该系统同时具备355 nm/532 nm波长米散射探测、532 nm退偏振探测、氮气和水汽分子拉曼探测的功能。两波长米散射探测功能可以精细探测大气边界层结构、大气气溶胶和云的消光特性及粗细粒子分布;532 nm退偏振探测功能可反映出大气气溶胶粒子和云粒子的形状特征,可识别出球形粒子(水云、污染型气溶胶和雾霾)和非球形粒子(沙尘与冰晶云等);氮气和水汽分子拉曼探测功能可获得水汽混合比的时空分布特征。该系统后继探测单元采用高稳定性的一体化结构,配置方舱具备防尘防水的恒温功能,可以直接在户外长期探测,有利于对局部气溶胶粒子和云粒子、水汽等物理参数间进行统计分析,已用于大气环境监测和科学等研究领域。
[8] 谭敏. 污染条件下气溶胶和水汽时空分布探测和数据分析研究[D]. 中国科学技术大学, 2018.
Tan Min. Study on spatial tempal distribution data analysis of aerosol water vap under pollution conditions[D]. Hefei: University of Science Technology of China, 2018. (in Chinese)
[14] Wu D, Wang Z, Liu D, et al. Independent calibration of water vap Raman lidar by using additional elastic measurements at water vap Raman wavelength [C]EPJ Web of Conferences EDP Sciences, 2016, 119: 25007.
[15] Deng Qian. Research on selfcalibration method of Raman Lidar water vap detection development of allsolidstate system[D]. Hefei: University of Science Technology of China(Anhui Institute of Optics Fine Mechanics, Chinese Academy of Sciences), 2019. (in Chinese)
Article Outline
李路, 邢昆明, 赵明, 邓迁, 王邦新, 庄鹏, 施云. 探测气溶胶-水汽的拉曼-米散射激光雷达系统[J]. 红外与激光工程, 2023, 52(4): 20220484. Lu Li, Kunming Xing, Ming Zhao, Qian Deng, Bangxin Wang, Peng Zhuang, Yun Shi. Raman-Mie scattering lidar system for detection of aerosol and water vapor in the atmosphere[J]. Infrared and Laser Engineering, 2023, 52(4): 20220484.