具有可变真空结构的物镜优化设计及性能分析
Environmental scanning electron microscopes (ESEMs) are widely employed for high-resolution observation of water containing, oil containing, and biological samples in low vacuum environments. However, at present, the development of ESEMs in China is almost blank, and most of them need to rely on imports. Therefore, the research on ESEMs can help improve China's independent development capability in this field, and provide a theoretical and experimental basis for the development of ESEMs in the future. Compared with conventional electron microscopy, the sample chamber of ESEMs should be in a low vacuum or ambient state. The vacuum value is generally on the order of 100 Pa, while that of the electron beam channel and the electron gun needs to be less than 1
Starting from the theory of electron optics, we consider the structure of the objective lens and the vacuum differential structure in the ESEM comprehensively. Firstly, two throttle tubes are designed between the sample chamber and the electron beam channel (near the lower pole shoe of the objective lens), and a transition zone is added inside the objective lens to form a three-level vacuum differential structure of the sample chamber, the transition zone and the electron beam channel. The vacuum in the transition zone should be two orders of magnitude higher than that in the sample chamber, and that in the electron beam channel should be two to three orders of magnitude higher than that in the transition zone. Considering the processing cost and difficulty of the elongated throttle tube, we adopt the combination of multiple diaphragms, which can more conveniently change the vacuum level by adjusting the aperture and number of diaphragm sheets in the diaphragm groove to achieve the required differential pressure difference. Then, the optimized design of a high-resolution ESEM objective lens and deflector is carried out based on a double-throttle vacuum resistance structure. Finally, an experimental platform is set up, and the objective magnetic field test, vacuum differential pressure test, and resolution test are carried out for validation.
Considering the objective structure and vacuum differential structure in the ESEM, a double throttle tube vacuum resistance structure as shown in Fig. 2 is designed to form a three-stage differential test structure (Fig. 10). This design can reduce the length of the throttle tube as a whole, which lowers the requirements for the aperture and length of the throttle tube to a certain extent, and thus reduces the influence on the working distance and the deflection field. Meanwhile, it can also reduce the gas residual situation in the narrow throttle tube, and reduce the influence of the electron beam drift in the narrow gas space in the throttle tube. The results of the vacuum differential pressure test show that the vacuum in the transition vacuum zone is two orders of magnitude higher than that in the sample chamber, and the vacuum in the electron beam channel is two to three orders of magnitude higher than that in the transition vacuum zone, which can meet the design requirements. The resolution test results show that in the current experimental conditions and the low vacuum environment mode of 133 Pa, the imaging resolution corresponding to the 20 μm×20 μm scanning field is better than 50 nm, and that corresponding to the 80 μm×80 μm scanning field is better than 100 nm when the working distance is 15 mm (Fig. 13).
Starting from the electron optics theory, we consider the objective lens structure and vacuum differential structure in the ESEM comprehensively, and the two are combined for the optimal design to provide a design method for the objective lens with variable vacuum structures. Systematic analyses, calculations, and simulations are carried out. Based on the theoretical analysis and simulation results, a magnetic field test platform and an ESEM experimental test platform are built for experiments, and the results show that in the current experimental conditions and low vacuum environment mode, the imaging resolution of 20 μm×20 μm scanning field corresponds to a resolution of better than 50 nm when the working distance is 15 mm. The overall closed-loop design and test of the objective lens with variable vacuum structures provide a theoretical and experimental basis for the development of ESEM.
1 引言
环境扫描电子显微镜(ESEM)是扫描电子显微镜(SEM)的一个重要应用分支。ESEM除了具备传统SEM的功能外,还可在低真空模式和环境模式下(133~2660 Pa)直接观察生物样品、含水样品以及含油样品等。从理论上讲,ESEM可选择高真空、低真空(真空度介于高真空与环境之间)和环境三种不同的工作模式对样品进行观测[1-2]。在国内,ESEM的研制工作几乎空白,但利用ESEM观测含水样品、含油样品以及生物样品等已有了广泛的应用场景,例如,李成星等[3]利用ESEM观测伪俏榕小蜂雌雄成虫触角感器的形态与分布;巩雪男[4]利用ESEM对石油地质的黏土矿物和油气存储层进行了分析研究;胡明玉等[5]利用ESEM对新型硅藻土调湿材料的吸附/解吸理论、改性机制调节以及调湿机制进行了研究。因此,开展ESEM的研究有助于提升我国在这方面的自主研制能力。
目前电镜类设备常采用热场发射电子枪,真空值一般小于1
2 具有可变真空结构的高分辨率ESEM的物镜优化设计
ESEM的设计初始目标:样品室真空值为10~133 Pa;系统的最优分辨率为1 nm;扫描范围为100 μm×100 μm。
2.1 电子束通道与样品室之间真空气阻结构设计
根据ESEM的设计要求,样品室的真空值为10~133 Pa,同时电子束通道的真空值小于1
考虑到在极大压差的电子束通道与样品室之间仅设计一个节流管的常规方法会带来诸多问题,因此,本文在样品室与电子束通道之间(即物镜下极靴附近)设计了两个节流管,在物镜内增设过渡区,形成样品室、过渡区和电子束通道三级真空差分结构。其中过渡区的真空度需要高于样品室的真空度2个数量级,电子束通道的真空度需要高于过渡区的真空度2~3个数量级。双节流管的设计可以在整体上减小节流管长度,一定程度上降低对节流管孔径和长度的要求,从而降低对工作距离和偏转场的影响;也可以减少狭长节流管中的气体残存,降低电子束在节流管中狭小气体空间的漂移的影响。
基于上述提出的三级真空差分结构,根据压差公式[11]计算可得:连接电子束通道与过渡区之间的节流管长度至少为2 mm,孔直径为0.4 mm;连接过渡区与样品室之间的节流管长度至少为3 mm,孔直径为1 mm。结合物镜的设计经验,对电子束通道与样品室之间的双节流管真空气阻结构进行机械设计,最终设计结果如
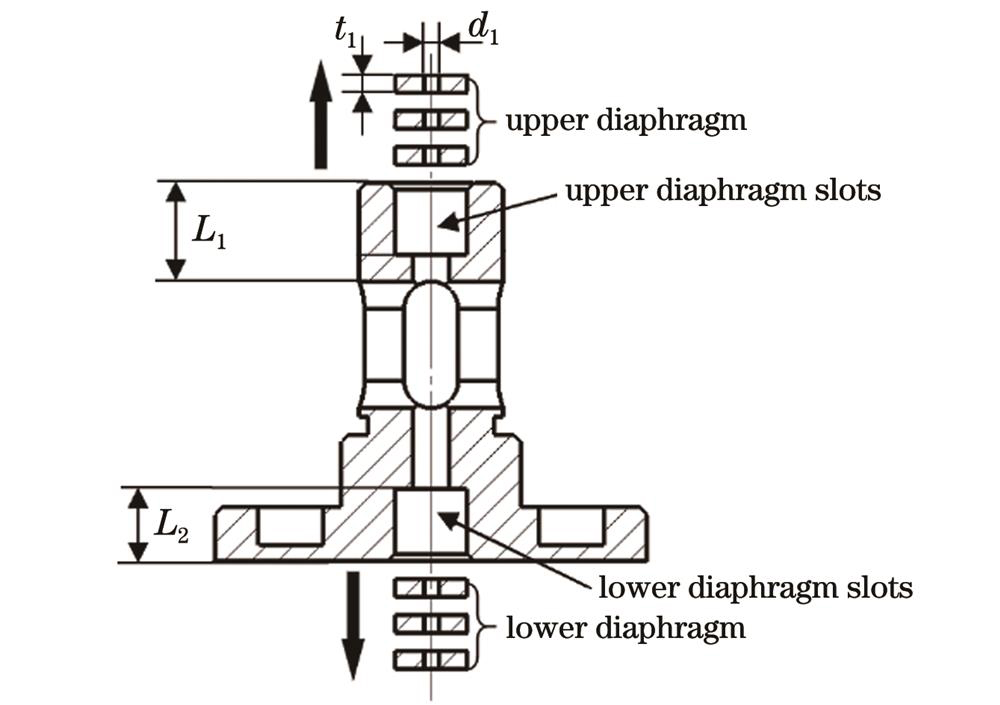
图 2. 双节流管真空气阻结构示意图
Fig. 2. Schematic diagram of vacuum resistance structure of double throttle tube
2.2 高分辨率ESEM的物镜优化设计
根据上述双节流管真空气阻结构的设计,进行高分辨率ESEM物镜的优化设计。在物镜优化设计中,主要选取束斑直径作为优化目标。束斑直径[12-13]可以近似表示为
式中:
加速电压30 kV对应的电子波长
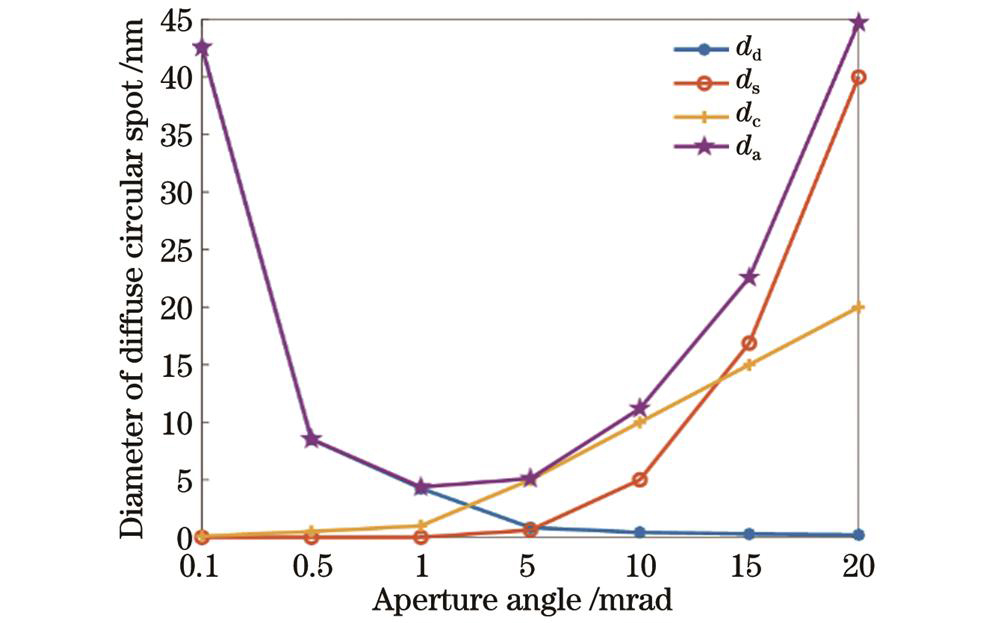
图 3. 各像差的弥散圆斑直径与束半角之间的关系图
Fig. 3. Relationship between diameter of diffuse circular spot of each aberration and half angle of beam
物镜一般采用
结合真空气阻结构的设计与上述理论分析,利用Murno’s Electron Beam Software(MEBS)电子光学计算软件对物镜和偏转器进行优化设计,最终采用如
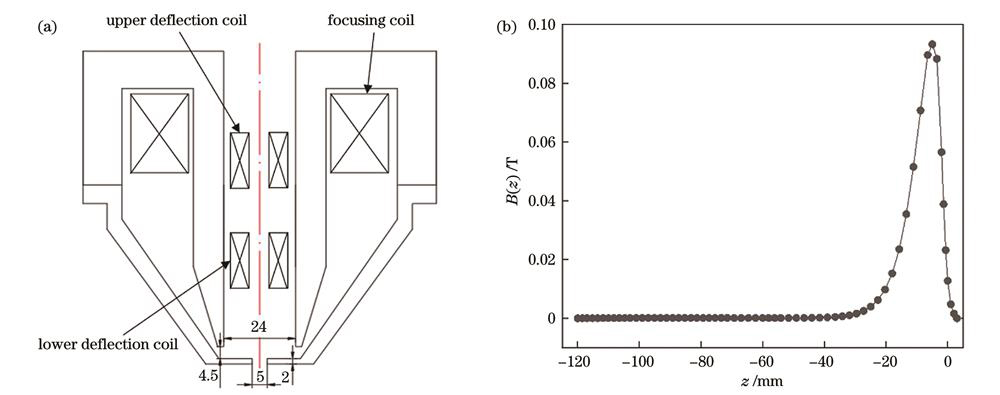
图 4. 优化后的物镜结构与轴上磁场的仿真结果。(a)优化后的物镜结构示意图;(b)激励为936安培匝数(1 A)对应的物镜轴上场分布
Fig. 4. Optimized objective structure and simulation results of magnetic field on axis. (a) Diagram of optimized objective structure; (b) field distribution of objective lens axis corresponding to excitation of 936 ampere-turns (1 A)
设置扫描场为100 μm×100 μm,上偏转线圈绕制6匝,根据几何关系计算,下偏转线圈绕制12匝,如图
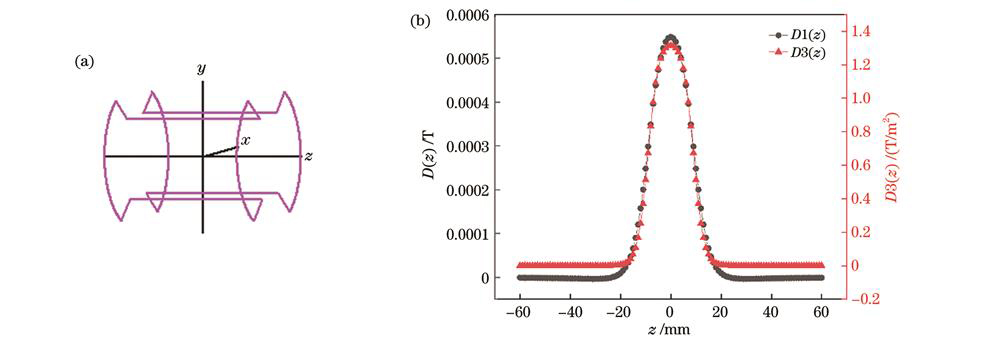
图 5. 上偏转中单个偏转线圈的仿真示意图。(a)仿真模型;(b)轴上磁场分布
Fig. 5. Simulation diagram of a single deflection coil in upper deflection. (a) Simulation model; (b) magnetic field distribution on axis
设置加速电压为30 kV,虚源直径为15 nm,虚源位置为0 mm,物镜位置为351.85 mm,上下偏转分别位于290.85 mm和326.85 mm处。将下极靴的下端面到样品表面的距离定义为工作距离,单物镜模式下,仅考虑聚焦场,不同工作距离下的束斑性能如
表 1. 仅考虑聚焦场时不同工作距离下的束斑性能参数
Table 1. Performance parameters of beam spot at different working distances when only considering focusing field
|
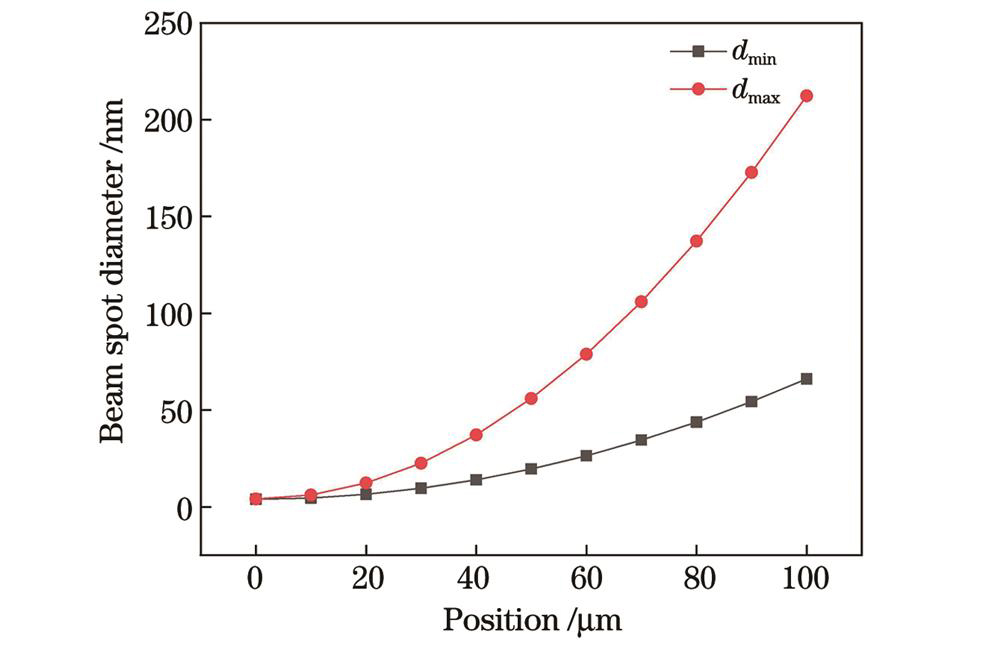
图 6. 同时考虑聚焦场和偏转场时不同扫描场对应的束斑尺寸(工作距离为15 mm)
Fig. 6. Beam spot sizes corresponding to different scanning fields when focusing field and deflection field are considered simultaneously (Working distance is 15 mm)
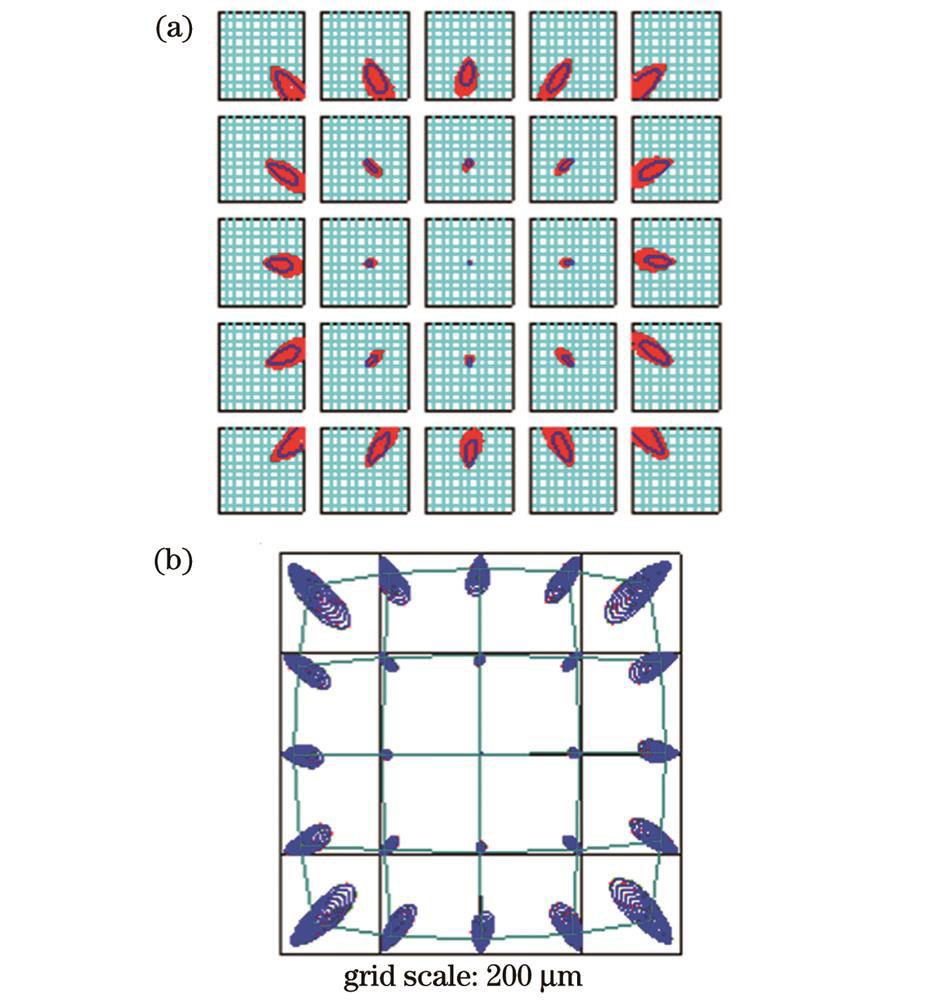
图 7. 电子束斑和像差斑仿真结果。(a)偏转场各位置的电子束斑;(b)偏转场各位置的像差斑
Fig. 7. Simulation results of electron beam spot and aberration spot. (a) Electron beam spots at various locations of deflection field; (b) aberration spots at various locations of deflection field
仿真结果显示,随着扫描场增加,束斑形状逐渐椭圆化,束斑直径会增加,且椭圆程度和束斑直径增加的速率变快。当扫描场小于20 μm×20 μm时,对应的束斑短轴直径为6.51 nm,长轴直径为12.32 nm。当扫描场大于20 μm×20 μm时,扫描场的增加对束斑直径影响变大,最终100 μm×100 μm的扫描场对应的束斑短轴直径为66.15 nm,长轴直径为212.30 nm。系统的成像分辨率与电子束的束斑直径密切相关,一般认为入射到样品表面的电子束束斑直径是扫描电镜分辨率的极限,因此可以将上述仿真结果作为实验结果的预测依据。
基于仿真结果进行机械设计,同时将双节流管真空气阻结构与物镜进行装配[18],最终形成如
3 性能测试
3.1 磁场测试
通过磁场测试平台对物镜和偏转线圈进行磁场分布测试。物镜线圈的实际匝数为936匝,
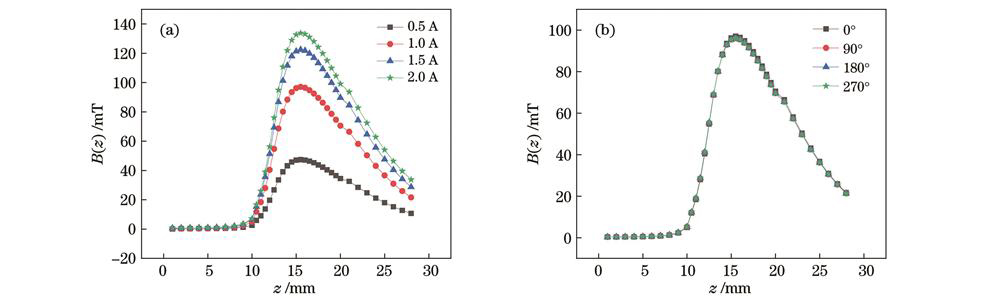
图 10. 物镜的磁场测试结果。(a)不同电流下对应的轴上磁场分布情况;(b)线圈通入1 A电流时,转台旋转不同角度的轴上磁场分布情况
Fig. 10. Magnetic field test results of objective lens. (a) Corresponding on-axis magnetic field distributions at different currents; (b) on-axis magnetic field distributions at different angles of rotation of rotary table when coil is energized with 1 A current
表 2. 物镜在不同电流下对应的轴上磁场最大值
Table 2. Maximum magnetic field values on axis corresponding to object lens at different currents
|
表 3. 上偏转线圈在不同电流下对应的轴上磁场最大值
Table 3. Maximum magnetic field values on axis corresponding to upper deflection coil at different currents
|
表 4. 下偏转线圈在不同电流下对应的轴上磁场最大值
Table 4. Maximum magnetic field values on axis corresponding to lower deflection coil at different currents
|
3.2 VP模式下分辨率测试
实验采取如下方案:上光阑槽放置5片厚度为0.5 mm且内孔直径为0.4 mm的光阑片;下光阑槽放置6片厚度为0.5 mm且内孔直径为1 mm的光阑片。
表 5. VP模式下真空度的理论值和实验测试值
Table 5. Theoretical and experimental test values of vacuum level under VP mode
|
搭建了如
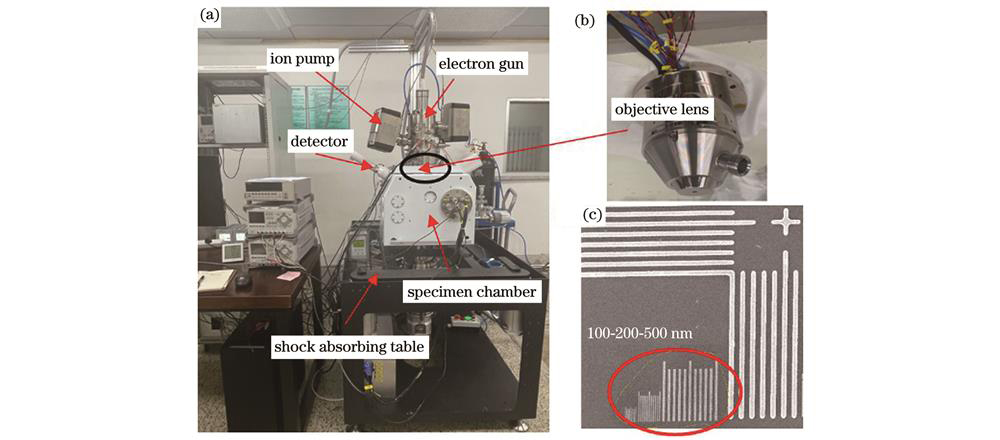
图 11. 实验平台与测试样品。(a)ESEM实验平台;(b)具有可变真空结构的物镜实物;(c)ZEISS Gemini SEM在高真空状态下的目标栅条样品成像结果
Fig. 11. Experimental platform and test sample. (a) ESEM experimental platform; (b) physical object of objective lens with a variable vacuum structure; (c) imaging results of target grid samples under high vacuum by ZEISS Gemini SEM
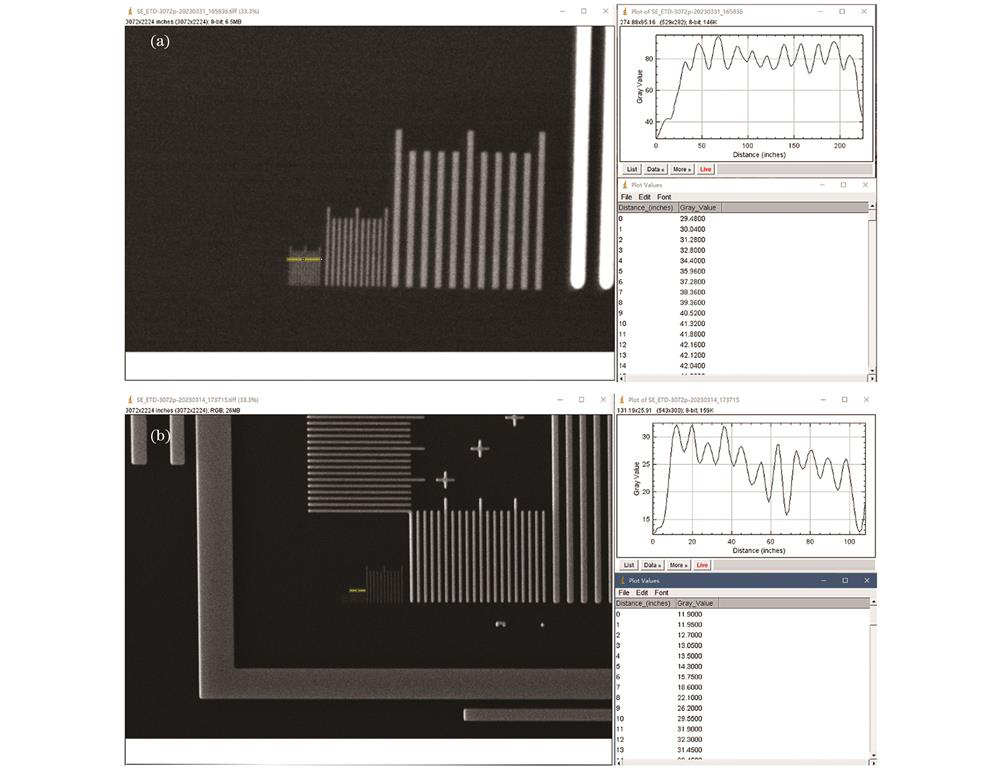
图 12. 成像结果与imageJ分析结果。(a)20 μm×20 μm的扫描场;(b)80 μm×80 μm的扫描场
Fig. 12. Imaging results and imageJ analysis results. (a) Scanning field of 20 μm×20 μm; (b) scanning field of 80 μm×80 μm
在实际实验中,由于二次电子探测器的安装位置导致工作距离不能太小,否则采集到的信号会很弱,而且受实验平台稳定性等条件的限制,
1)目前搭建的ESEM实验平台对中精度不高,导致像散大,超出了现有消像散器的调节范围,从而使得成像分辨率与仿真分辨率有较大差异;
2)为了提高气阻比,使用了多片0.5 mm厚的光阑片,这可能导致电子束以一定发散角在经过多个较厚光阑片时,碰撞光阑内壁产生杂散电子,进而导致成像分辨率下降;
3)样品的限制,用于实验测试的标准样品S1000 nm可分辨的最小尺寸为50 nm。
此外,实验平台的稳定性以及探测器的成像效果也会影响分辨率,因此,认为上述实验结果可初步验证仿真结果。
4 结论与展望
本文从电子光学理论出发,综合考虑了ESEM中物镜结构和真空差分结构,将两者结合在一起进行了优化设计,提出了一种具有可变真空结构的物镜设计方法,并进行了系统的分析、计算和仿真。在理论分析和仿真结果的基础上,搭建了磁场测试平台以及ESEM实验测试平台进行实验,结果表明,在目前实验条件和低真空环境模式下,工作距离为15 mm时,20 μm×20 μm的扫描场对应的成像分辨率优于50 nm。具有可变真空结构物镜的整体闭环设计与测试,为ESEM的研制提供了理论与实验基础。在今后的工作中:
1)将结合实验结果对现有的计算模型作进一步优化,调节节流管的位置,使电子束经过节流管时的发散角较小,从而减少碰撞内壁产生的杂散电子,进而提高分辨率;
2)进一步提高标准样品的图形最小可分辨尺寸,但这也将导致加工难度提升;
3)对实验平台进行改进优化,同时扩大消像散的调节范围,进一步提高其稳定性、对中性能和分辨率使其应用于国产ESEM中。
[1] 袁莉民, 蒋蔚霞. 对环境扫描电子显微镜(ESEM)的认识[J]. 现代科学仪器, 2001(2): 53-56.
Yuan L M, Jiang W X. About environmental scanning electron microscope[J]. Modern Scientific Instruments, 2001(2): 53-56.
[2] Danilatos G D. Introduction to the ESEM instrument[J]. Microscopy Research and Technique, 1993, 25(5/6): 354-361.
[3] 李成星, 刘志祥, 杨培, 等. 伪鞘榕小蜂雌雄成虫触角感器的超微形态、分布及适生意义[J]. 环境昆虫学报, 2020, 42(2): 400-409.
Li C X, Liu Z X, Yang P, et al. Ultramorphology, distribution and ecological significance of antennal sensilla of female and male Sycoscapter trifemmensis (Hymenoptera: Pteromalidae)[J]. Journal of Environmental Entomology, 2020, 42(2): 400-409.
[4] 巩雪男. 环境扫描电镜在地质中的应用与研究[J]. 西部探矿工程, 2022, 34(4): 61-64.
Gong X N. Application and research of environmental scanning electron microscope in geology[J]. West-China Exploration Engineering, 2022, 34(4): 61-64.
[5] 胡明玉, 刘章君, 樊财进, 等. 新型硅藻土调湿材料温湿度调节效果及机制[J]. 功能材料, 2019, 50(3): 3014-3019.
Hu M Y, Liu Z J, Fan C J, et al. Effect of temperature and humidity control and the mechanism of new diatomite humidity-controlling materials[J]. Journal of Functional Materials, 2019, 50(3): 3014-3019.
[6] Myers B D, Dravid V P. Variable pressure electron beam lithography (VP-eBL): a new tool for direct patterning of nanometer-scale features on substrates with low electrical conductivity[J]. Nano Letters, 2006, 6(5): 963-968.
[7] Postek M T, Jr, Vladár A E. New application of variable-pressure/environmental microscopy to semiconductor inspection and metrology[J]. Scanning, 2004, 26(1): 11-17.
[8] Slówko W, Wiatrowski A, Krysztof M. Detection of secondary and backscattered electrons for 3D imaging with multi-detector method in VP/ESEM[J]. Micron, 2018, 104: 45-60.
[9] Slówko W, Wiatrowski A. Coaxial ion micro-source for VP/ESEM-E-beam impact mode[J]. Vacuum, 2016, 132: 53-61.
[10] Danilatos G D. Review and outline of environmental SEM at present[J]. Journal of Microscopy, 1991, 162(3): 391-402.
[11] 达道安. 真空设计手册[M]. 2版. 北京: 国防工业出版社, 1991: 98-132.
DaD A. Vacuum design manual[M]. 2nd ed. Beijing: National Defense Industry Press, 1991: 98-132.
[12] 杜秉初, 汪健如. 电子光学[M]. 北京: 清华大学出版社, 2002: 216-251.
DuB C, WangJ R. Electron optics[M]. Beijing: Tsinghua University Press, 2002: 216-251.
[13] 华中一, 顾昌鑫. 电子光学[M]. 上海: 复旦大学出版社, 1992.
HuaZ Y, GuC X. Electron optics[M]. Shanghai: Fudan University Press, 1992.
[14] 沈一骑. 磁透镜极靴形状对其电子光学参量的影响[J]. 电子显微学报, 1996, 15(1): 59-65.
Shen Y Q. Influences of polepiece optical parameters shapes on the electron of megnatic lenses[J]. Journal of Chinese Electron Microscopy Society, 1996, 15(1): 59-65.
[15] 陈文雄. 极靴磁饱和如何影响磁透镜的球差系数和色差系数[J]. 电子显微学报, 1988, 7(1): 55-66.
Chen W X. How does polepiece saturation affect the spherical and chromatic aberration coefficient of the magnetic lenses[J]. Journal of Chinese Electron Microscopy Society, 1988, 7(1): 55-66.
[16] 西门纪业,葛肇生. 电子显微镜的原理和设计[M]. 北京: 科学出版社, 1979:27-35.
XimenJ Y, GeZ S. Principle and design of electron microscope[M]. Beijing: Science Press, 1979: 27-35.
[17] 史丽娜, 刘俊标, 牛耕, 等. 棒阳极X射线源聚焦系统的电子光学设计[J]. 光学学报, 2022, 42(22): 2234001.
[18] 王鹏飞, 史丽娜, 赵伟霞, 等. 一种物镜及环境扫描电镜: CN115527823A[P]. 2022-12-27.
WangP F, ShiL N, ZhaoW X, et al. An objective lens and environmental scanning electron microscope: CN115527823A[P]. 2022-12-27.
Article Outline
史丽娜, 王鹏飞, 刘俊标, 王岩, 殷伯华, 韩立. 具有可变真空结构的物镜优化设计及性能分析[J]. 光学学报, 2024, 44(5): 0522002. Lina Shi, Pengfei Wang, Junbiao Liu, Yan Wang, Bohua Yin, Li Han. Optimized Design and Performance Analysis of Objective Lenses with Variable Vacuum Structures[J]. Acta Optica Sinica, 2024, 44(5): 0522002.