微柱镜面周期性纹理结构衍射杂散光研究
In the field of optics, micro-cylindrical lenses and cylindrical lens arrays are commonly used optical devices usually processed by traditional mechanical grinding, ultraprecision turning, and die forming. In the process of diamond lathe machining, the tool machining step often has a constant period, resulting in the formation of a periodic texture structure corresponding to the knife pattern on the lens surface. This structure causes an obvious diffraction phenomenon under strong laser irradiation and produces stray light that interferes with the optical path in the optical system, seriously affecting the function of the optical system. However, there are few reports on this phenomenon at present, resulting in a lack of theoretical guidance for solving this problem.
To analyze the influence of stray light on the surface of a 100-μm-diameter micro-cylindrical lens caused by lathe machining, a model of the periodic texture structure is constructed, and the influence of the surface periodic texture structure on diffractive stray light is analyzed. The theoretical diffraction model of the structure is established by using Fourier optical theory, and the intensity distribution rules of the diffracted light field at different depths are calculated. The theoretical rules obtained are basically consistent with the physical optics simulation and experimental test results of the commercial optical software VirtualLab, and the theoretical model is verified theoretically and experimentally.
Based on the model of the periodic texture structure, the light spots of the ideal lens are compared with those of the lens with the periodic texture structure. As shown in Fig.5, compared with those of the ideal lens, the light spots of the lens with the periodic texture structure produce obvious diffractive stray light along the fast axis direction. In view of this phenomenon, the beam intensity distributions are calculated and simulated at different depths of the periodic texture structure on the surface of the micro-cylindrical lens, as shown in Fig.6. It can be seen that, the deeper the periodic structure, the stronger the diffractive stray light generated. When the surface texture depth of the cylindrical lens is greater than 16.2 nm, the diffracted stray light is more significant, which has a significant impact on the optical performance of the device. This is basically consistent with the experimental result. Finally, according to the research results, a method is proposed to control the depth of the periodic structure and destroy the periodic characteristics of the surface to weaken the diffractive stray light.
In this study, the relationship between the periodic texture of the micro-cylindrical lens and the diffracted stray light of the beam is studied, an analysis model of the periodic texture structure of the micro-cylindrical lens is established, and theoretical calculation and simulation analysis methods for analyzing the relationship between the periodic structure and the intensity of the diffracted stray light are given. This research provides a theoretical analysis method for solving the problem of diffractive stray light caused by periodic knife grain in the machining process of micro-cylindrical lens.
1 引言
微柱透镜及柱透镜阵列在激光照明、激光切割、激光显示及光刻等领域具有广泛应用[1-3]。如在工业领域,作为基础光学器件,微柱透镜因准直效果好、结构紧凑、能量损耗低等优点[4],常用于体积较小的半导体激光器的光束整形[5]。随着应用场景的不断拓展,市场对微柱透镜及其阵列透镜的要求不断上升,对该类器件的快速高精度加工需求更加迫切。
微柱透镜通常采用传统机械磨削、超精密车削及模压成形等方法加工而成[6-7]。在车床加工过程中,车床刀具的步进常为恒定周期,这决定了透镜表面必然存在与刀纹对应的周期性纹理结构。这一周期性纹理结构在强激光照射下将激发明显的衍射现象[8],并在光路中形成干扰系统的杂散光,严重影响光学系统功能。然而,目前关于这一现象的研究报道较少,导致该问题的解决缺乏理论指导依据。
基于这一现状,本文开展了柱透镜加工表面的周期性纹理对光束质量影响的研究,在傅里叶光学的基础上建立了具有周期性纹理特征的柱透镜的衍射杂散光分析模型,并结合VirtualLab物理光学仿真和快轴准直微柱透镜实验,基于该模型对衍射杂散光的分布进行了理论计算、模拟仿真和实验验证,得到了一致的规律,为该类光学器件中周期纹理引起的衍射杂散光问题的解决提供了理论参考。
2 现象
为了研究表面周期性纹理对光束质量的影响,以半导体激光快轴准直微柱透镜为例,搭建了
表 1. 快轴准直微柱透镜设计参数
Table 1. Design parameters of fast axis collimating micro-cylindrical lens
|
经过实验测试,光电探测器阵列所获得的准直后的光斑分布如

图 2. 微柱透镜调试光斑和对应的截面轮廓特征图。(a)测试得到的光斑图;(b)快轴微柱透镜表面轮廓及局部误差测试结果
Fig. 2. Debugging spot of micro-cylindrical lens and corresponding cross-section profile feature. (a) Spot pattern obtained by measurement; (b) surface profile and local error test result of fast axis micro-cylindrical lens
3 结构与模型
为了对上述微柱透镜表面的周期性纹理结构及其产生的衍射杂散光进行理论分析,基于车床加工时残留刀纹的特征,建立了
根据
式中:
对于微柱透镜,其理想面形部分所对应的厚度函数h1(x)满足
式中:a为理想微柱透镜的中心厚度;R为理想微柱透镜的曲率半径。
对于周期性纹理结构,根据刀具及其步进工作方式,得到其厚度函数h2(x)为
式中:
透镜的相位变换函数,可以由光程给出,透镜的光程
式中:
然而,透镜子口径大小是有限的,相当于存在一个有限大小的孔径,为了表示透镜的有限孔径效应,加入光瞳函数[
式中:k为空间角频率;在透镜孔径内
探测面上光场的复振幅分布为入射光复振幅乘以透镜透射系数的傅里叶变换结果,对振幅进行平方运算就可以得到光强的分布。入射球面波复振幅分布的一般形式[11]表示为
式中:
因此探测面复振幅分布可以表示为
式中:
探测面光强分布为
4 讨论分析
由上述模型可知,实际加工柱透镜的面形由理想面和周期纹理结构叠加而成。为了对上述结构及其产生的衍射杂散光理论模型进行验证,首先利用软件建立
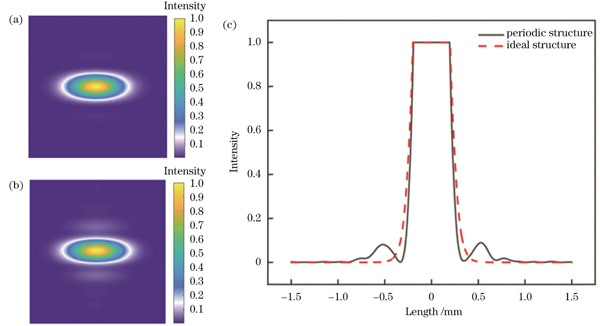
图 4. 仿真光斑和快轴方向的光强分布图。(a)H=0 nm时的光斑;(b)H=26.8 nm时的光斑;(c)光强截面分布图
Fig. 4. Simulated spots and intensity distribution along fast axis direction. (a) Spot when H=0 nm; (b) spot when H=26.8 nm; (c) cross-sectional distribution of light intensity
通过对该光分布中主光斑的分析可知,柱透镜表面周期纹理特征并不会影响光束准直性,不同深度H下主光斑的快轴发散角和直径基本一致,发散角约为0.4°,直径为0.48 mm。然而,与
为了进一步量化衍射杂散光,沿光分布图中白色虚线箭头方向(快轴方向)对光斑能量进行截取,并采用与
为了进一步验证所建立的理论模型的准确性,选取6组实际加工得到的微柱透镜,H分别为13.1、15.3、16.2、18.2、22.8、26.8 nm,采用
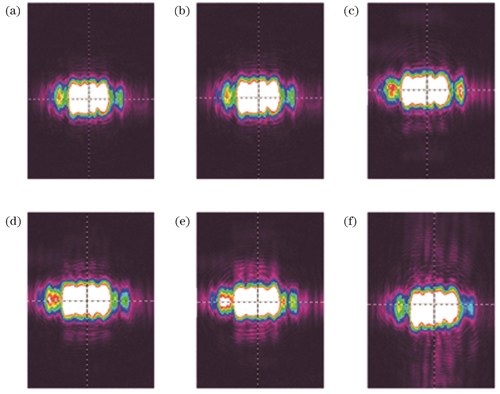
图 5. 实验测得的光斑图。(a)H=13.1 nm;(b)H=15.3 nm;(c)H=16.2 nm;(d)H=18.2 nm;(e)H=22.8 nm;(f)H=26.8 nm
Fig. 5. Spot patterns obtained by experiment. (a) H=13.1 nm; (b) H=15.3 nm; (c) H=16.2 nm; (d) H=18.2 nm; (e) H=22.8 nm; (f) H=26.8 nm
针对上述6组器件,采用所建立的理论分析模型以及商业软件进行计算,获得6组H不同的柱透镜的衍射峰值强度,如
此外,通过上述模型的分析和实验可知,透镜表面周期纹理结构能够产生固定级次和角度的衍射现象。因此可尝试破坏其表面周期特征,以达到削弱杂散光的目的。在H同为26.8 nm的情况下,根据理论模型,在理想微柱透镜面形上构建了
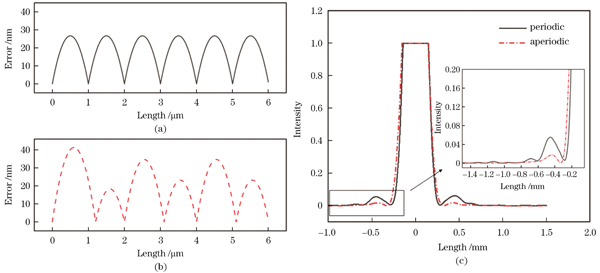
图 7. 微柱透镜截面误差和对应的快轴方向的光强分布。(a)周期结构刀纹细节图;(b)非周期结构刀纹细节图;(c)微柱透镜的光强截面分布图
Fig. 7. Cross-sectional error of micro-cylindrical lens and corresponding light intensity distribution along fast axis direction. (a) Detail view of knife pattern with periodic structure; (b) detail view of knife pattern with aperiodic structure; (c) cross-sectional distribution of light intensity of micro-cylindrical lens
5 结论
研究了微柱透镜表面周期性纹理与光束衍射杂散光之间的关系,建立了微柱透镜周期纹理结构的分析模型,给出了周期结构与衍射杂散光强度关系的理论计算和仿真分析方法,该方法得到的计算结果与实验结果基本一致。研究结论为解决微柱透镜加工过程中周期结构刀纹产生的衍射杂散光问题提供了理论分析方法。
[1] 赵旺, 董理治, 杨平, 等. 目标粗糙特性对主动照明信标波前探测的影响[J]. 中国激光, 2019, 46(7): 0705002.
[2] Dubey A K, Yadava V. Laser beam machining—a review[J]. International Journal of Machine Tools and Manufacture, 2008, 48(6): 609-628.
[3] 甘雨, 张方, 朱思羽, 等. 光刻机照明系统光瞳特性参数的评估算法[J]. 中国激光, 2019, 46(3): 0304007.
[4] Guo C W, Xiong B X, Zhang G J, et al. LD beam collimation based on aspheric cylindrical lens[J]. Proceedings of SPIE, 2019, 10838: 1083817.
[5] 许昌, 彭钦军, 王志敏, 等. 一种光束整形的光学系统: CN218767618U[P]. 2023-03-28.
XuC, PengQ, WangZ, et al. Optical system for beam shaping: CN218767618U[P]. 2023-03-28.
[6] 郑鑫宇. 柱阵列玻璃透镜模压成形仿真及实验研究[D]. 长沙: 湖南大学, 2021.
ZhengX Y. Simulation and experimental study on compression molding of cylindrical array glass lens[D]. Changsha: Hunan University, 2021.
[7] 青建宏. 基于紫外压印技术的柱面微透镜阵列制备研究[D]. 成都: 中国科学院光电技术研究所, 2022.
QingJ H. Research on the fabrication of cylindrical microlens array based on UV imprinting technology[D]. Chengdu: Institute of Optics and Electronics, Chinese Academy of Sciences, 2022.
[8] 郭福源, 李连煌, 郑华. 小平坦波面光场的衍射与准直特性[J]. 光学学报, 2021, 41(4): 0426001.
[9] 蒋全伟, 张兰平, 郭林辉, 等. 高亮度半导体激光尾纤模块中光学补偿方法[J]. 中国激光, 2021, 48(11): 1101003.
[10] 赵凯华, 钟锡华. 光学[M]. 北京: 北京大学出版社, 1984.
ZhaoK H, ZhongX H. Optics[M]. Beijing: Peking University Press, 1984.
[11] 郁道银, 谈恒英. 工程光学[M]. 2版. 北京: 机械工业出版社, 2006.
YuD Y, TanH Y. Engineering optics[M]. 2nd ed. Beijing: China Machine Press, 2006.
孙昊, 唐乐, 王春艳, 张为国, 杜春雷, 夏良平. 微柱镜面周期性纹理结构衍射杂散光研究[J]. 中国激光, 2024, 51(5): 0513001. Hao Sun, Le Tang, Chunyan Wang, Weiguo Zhang, Chunlei Du, Liangping Xia. Diffractive Stray Light of Periodic Texture of Micro‑cylindrical Lens[J]. Chinese Journal of Lasers, 2024, 51(5): 0513001.