线黏弹性端接布拉格光纤光栅传感器应变传递机理
下载: 1076次
1 引言
光纤光栅传感器(FBG)由于具有质量小、体积小、抗电磁干扰能力强、灵敏度高和易于复用等优点,在结构损伤监测领域中得到了广泛应用。当光纤光栅应用于基体结构的应力应变监测时,由于光纤光栅和基体结构通过中间层固定,存在应变传递损耗,因此光纤光栅所测得的应变与基体的应变不同[1]。国内外科研工作者开展了大量针对光纤应变与基体应变之间关系的研究,取得了大量成果。Ansari等[2-11]采用一定的假设条件,得出埋入式光纤光栅应变传感器的传递表达式。Qin等[12-19]推导出表面黏贴式光纤光栅传感器的应变传递方程。刘明尧等[20-21]推导了胶层黏弹性的表面黏贴式和埋入式FBG传感器的应变传递规律。但是,上述研究均是针对栅区黏贴光纤光栅传感器的应变传递。尽管栅区黏接工艺简便,但栅区黏接传感器中胶黏性和受力的不确定性易产生多峰现象,从而导致结果的错误判断。与栅区黏接相比,端接黏接的传感器仅FBG栅区两端受力,栅区不直接受力,栅区内各点受力相等。吴俊等[22]依据变形等效原理,推导了表面黏贴式非栅区封装FBG的应变传递函数,但是推导中仅考虑了黏接层弹性模量和栅区长度的影响。Sun等[23]基于对称性推导了夹持式光纤光栅传感器的应变传递公式。
本文推导了线黏弹性表面端接黏贴式光纤光栅与基体结构之间的应变传递关系,讨论分析了影响平均应变传递率的因素,通过有限元验证了理论方程的有效性。
2 线黏弹性表面黏贴式端接光纤光栅传感器应变传递机理
表面黏贴式端接FBG传感器主要由基体、FBG(光纤纤芯和包层)和黏接层组成,表面黏贴式端接FBG传感器示意图如
为简化分析模型,采用如下假设:光纤、黏接层和基体之间交界面结合紧密,没有脱落;光纤和基体结构均为线弹性材料,并且基体沿光纤的轴线方向均匀变形;黏接层为线黏弹性材料;只有基体结构受到轴向外力作用时,其应变通过剪应变传递,而黏贴层和光纤不直接受力;不考虑温度等环境的影响。
沿
对黏接层微段进行受力分析,可得
根据材料黏弹性积分型本构方程,可得黏接层应力/应变关系的Stieltjes卷积缩写形式,即
式中:
对(2)式和(4)式进行拉普拉斯(Laplace)变换,可得
式中:
根据积分型本构方程弹性-黏弹性对应原理,可得
式中:
中间层一般为环氧树脂胶,其蠕变柔量与光纤相差较大(一般10倍以上),故可认为
由于光纤的长度远大于其直径,因此忽略光纤径向变形,可得
式中:
对(13)式两边进行Laplace变换,可得
令
对
其通解为
式中:
经Laplace变换得
代入(17)式可得
所以黏接区域光纤应变方程为
根据
根据光纤、黏贴层和基体结构同步变形,光纤轴向变化量与基体轴向变化量相同,可得
在基体受到
式中:L-1为Laplace逆变换;
3 瞬时和准静态应变传递分析
将黏接层的黏弹性模型简化为一个Kelvin模型和一个弹簧串联而成的标准线性固体模型,如
标准线性固体模型在应力
式中:
瞬时蠕变柔量为
准静态蠕变柔量为
则可得FBG瞬时响应为
准静态的应变传递率为
4 分析与讨论
4.1 黏接层参数影响分析
光纤和黏接层的物理参数如
表 1. 光纤的物理参数
Table 1. Physical parameters of optical fiber
|
由
表 2. 黏接层的物理参数
Table 2. Physical parameters of adhesive layer
|
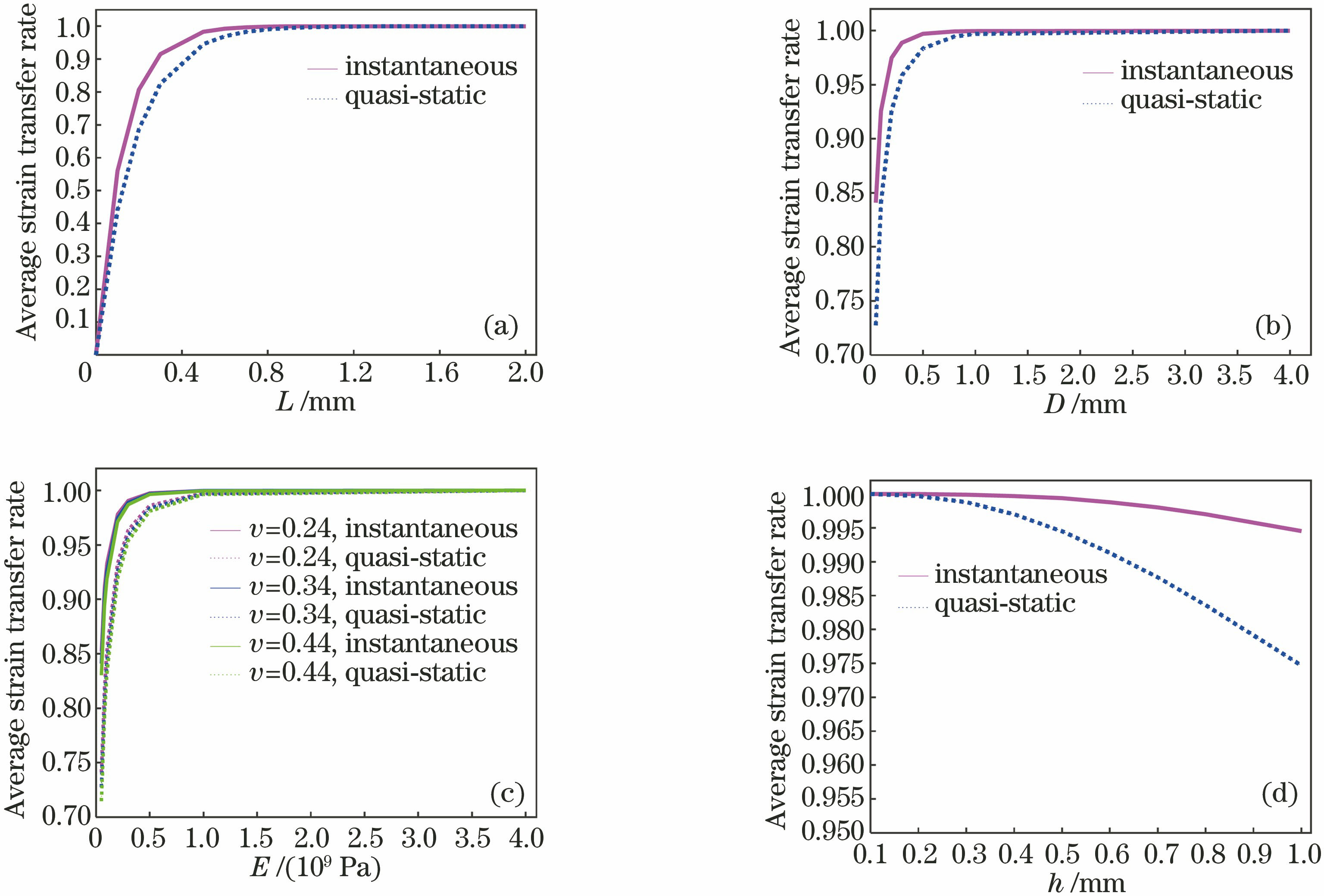
图 5. 黏接层参数对应变传递率的影响。(a)长度;(b)宽度;(c)杨氏模量;(d)下部厚度
Fig. 5. Influences of adhesive layer parameters on strain transfer rate. (a) Length; (b) width; (c) Young's modulus; (d) thickness of lower part
4.2 数值验证
为验证理论预测模型的有效性,利用ANSYS Workbench 17.0进行有限元(FEM)分析。黏接层参数:
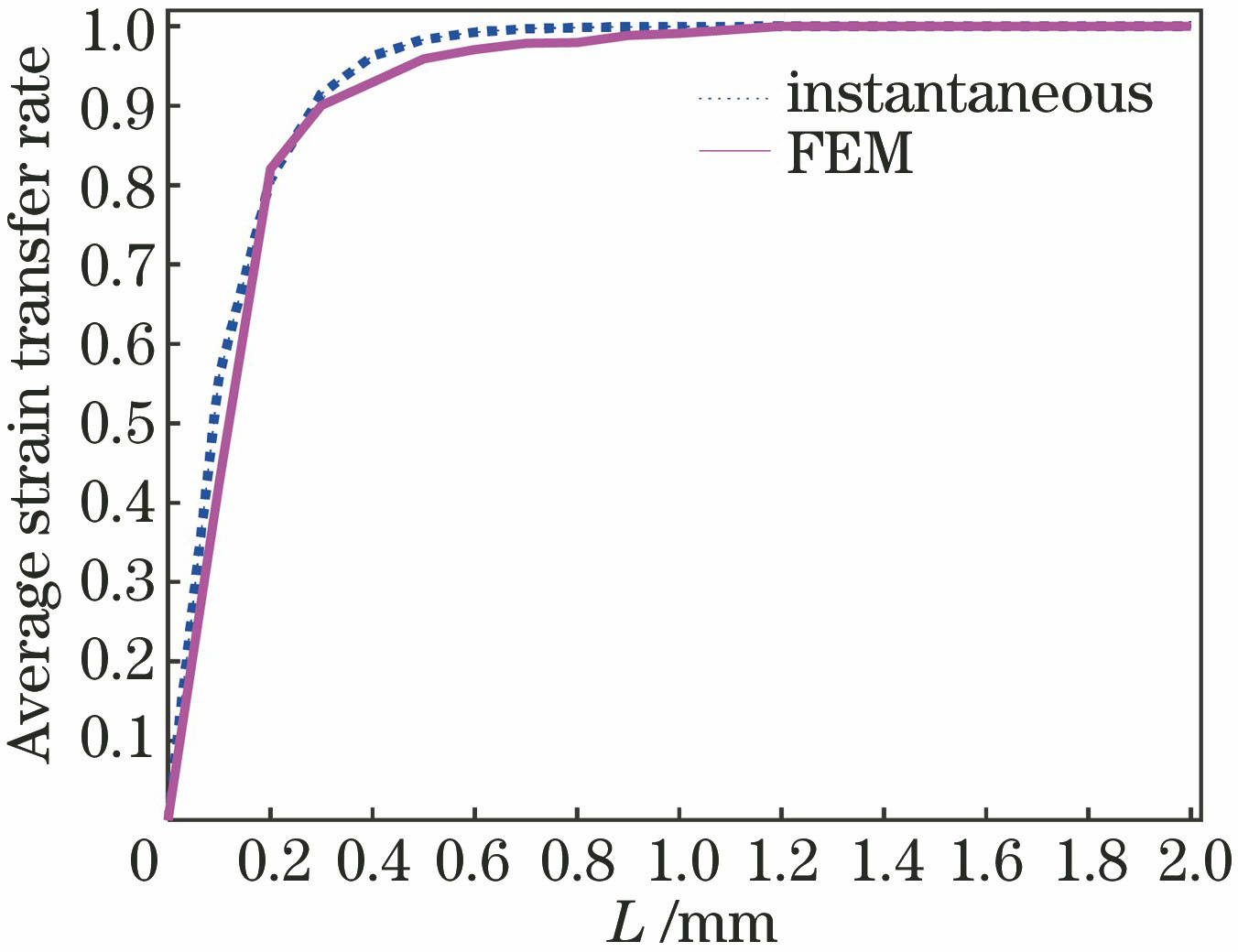
图 7. 理论解和有限元结果对比
Fig. 7. Comparison between theoretical solutions and results obtained by FEM
5 结论
端接光纤光栅应变传感器已经得到越来越广泛的应用。以表面端接黏贴式光纤光栅为研究对象,基于剪滞理论,研究了表面黏贴式端接光纤光栅应变传递机理,建立了光纤光栅和基体之间的平均应变传递模型,给出表面黏接式端接光纤光栅传感器的参数优化规律。与现有的方法相比,本方法提高了测量精度,简化了封装工艺。为了验证理论预测的正确性,利用有限元法与提出的模型进行对比,结果表明两者具有很好的一致性。提出的模型为端接光纤光栅应变传感器的设计和应用提供了理论依据。
[2] Ansari F, Yuan L B. Mechanics of bond and interface shear transfer in optical fiber sensors[J]. Journal of Engineering Mechanics, 1998, 124(4): 385-394.
[5] Li Q B, Li G, Wang G L, et al. Elasto-plastic bonding of embedded optical fiber sensors in concrete[J]. Journal of Engineering Mechanics, 2002, 128(4): 471-478.
[6] Zhou J, Zhou Z, Zhang D. Study on strain transfer characteristics of fiber Bragg grating sensors[J]. Journal of Intelligent Material Systems and Structures, 2010, 21(11): 1117-1122.
[8] Li H N, Zhou G D, Ren L, et al. Strain transfer coefficient analyses for embedded fiber Bragg grating sensors in different host materials[J]. Journal of Engineering Mechanics, 2009, 135(12): 1343-1353.
[10] Wan K T. Leung C K Y, Olson N G. Investigation of the strain transfer for surface-attached optical fiber strain sensors[J]. Smart Materials and Structures, 2008, 17(3): 035037.
[11] 覃荷瑛, 朱万旭, 张贺丽, 等. 内嵌预压式大量程光纤光栅传感器的智能钢绞线的研制与性能分析[J]. 中国激光, 2017, 44(4): 0410001.
[12] 覃荷瑛, 霍婷婷, 朱万旭. 螺旋倾斜复合技术对光纤布拉格光栅传感器的减敏作用[J]. 激光与光电子学进展, 2017, 54(3): 030601.
[17] 田石柱, 张国庆, 王大鹏. 表面式光纤布拉格光栅传感器应变传递机理的研究[J]. 中国激光, 2014, 41(8): 0805005.
[18] 张桂花, 柴敬, 李旭娟, 等. 基片式光纤光栅应变传感器的应变传递研究[J]. 激光与光电子学进展, 2014, 51(1): 010601.
[19] 由泽伟, 王源, 孙阳阳, 等. 预张拉光纤布拉格光栅应变传递规律实验研究[J]. 激光与光电子学进展, 2016, 53(11): 110501.
[20] 刘明尧, 季冬亮, 肖爽, 等. 胶黏剂黏弹性对黏贴式FBG应变传递的影响[J]. 光学精密工程, 2016, 24(6): 1307-1318.
[21] 常新龙, 李明, 谷小飞. 基于线黏弹性的埋入式聚合物光纤传感器应变传递研究[J]. 固体火箭技术, 2010, 33(3): 353-359.
[22] 吴俊, 陈伟民, 余葵, 等. 非栅区封装光纤布喇格光栅应变传感特性研究[J]. 光子学报, 2016, 45(2): 0206004.
[23] Sun L, Li C, Li J, et al. Strain transfer analysis of a clamped fiber Bragg grating sensor[J]. Applied Sciences, 2017, 7(2): 188.
陈光, 丁克勤, 冯其波, 高瞻. 线黏弹性端接布拉格光纤光栅传感器应变传递机理[J]. 激光与光电子学进展, 2018, 55(11): 110604. Guang Chen, Keqin Ding, Qibo Feng, Zhan Gao. Strain Transfer Mechanism of End-Bonding Fibre Bragg Grating Sensors Based on Linear Viscoelasticity[J]. Laser & Optoelectronics Progress, 2018, 55(11): 110604.