激光粉末床熔化制备TiB2颗粒增强7050铝基复合材料的组织及性能研究(特邀)特邀研究论文
Laser powder bed fusion (LPBF) is a metal additive manufacturing technology that utilizes high-power lasers to melt and stack powdered materials for rapid prototyping. However, metals used in current lightweight and high-strength LPBF processes are extremely limited. In particular, in terms of aluminum alloys, LPBF-formed aluminum alloys are mainly focused on Al-Si alloys. The inherent un-weldability of 7050 aluminum alloy and the high thermal stress induced by rapid cooling during the additive manufacturing process cause cracking or deformation during LPBF, limiting the application of LPBF technology in the preparation of this type of alloys. This study investigates the LPBF formation of TiB2/7050 composite and subsequent heat treatment (solution + aging treatment) process, exploring the effects of different process parameters and heat treatment conditions on the microstructures and room temperature tensile properties of TiB2/7050 composite.
Orthogonal methods are employed to select laser process parameters, including laser power levels of 210, 225, 240, and 255 W. The scanning speed levels are set at 400, 450, 500, 550, and 600 mm/s, whereas hatch spacings are set at 75 μm and 90 μm. The scanning strategy involves a layer-by-layer rotation of 90° using the stripe scanning method. In total, there are 40 sets of process parameters. The heat treatment is conducted via solution treatment in an muffle furnace. After the samples are solubilized at 475 °C for 1 h, they are cooled in room temperature water and then aged at 120 °C for 12 h in a tubular resistance furnace before final cooling in ambient air. Tensile experiments are performed using a room temperature tensile device at a strain rate of 10-4 s-1. Tensile specimens with appropriate dimensions are prepared, and three samples in equivalent conditions are tested to obtain the average values. X-ray diffractometer is employed to analyze the phase composition of the samples. The scan angle range is set between 20° and 120° with a scan rate of 2 (°)/min. Microstructural characterization is performed using a scanning electron microscope (SEM), and the material surface elemental compositions are analyzed using an energy-dispersive spectrometer (EDS).
Under the following four sets of process parameters (laser power + scanning speed + scanning spacing), the relative densities of the printed sample are higher: 240 W+450 mm/s+75 μm (No.1), 240 W+500 mm/s+75 μm (No.2), 240 W+450 mm/s+90 μm (No.3), and 240 W+500 mm/s+90 μm (No.4). The obtained relative densities of TiB2/7050 composite samples are approximately 98.3%, 98.7%, 98.5%, and 99.1%, respectively (Fig.2). The tensile experiments under these four sets of parameters are conducted at room temperature on the as-built and heat-treated samples. In the as-built state, the TiB2/7050 composite exhibits the highest strength under the condition 240 W+450 mm/s+75 μm (No.1), with tensile strength, yield strength, and elongation being 286 MPa, 250 MPa, and 2.3%, respectively (Fig.4). After heat treatment, both the strength and plasticity of the material significantly improve for all four sets of parameters. In particular, under the as-built condition of 240 W+450 mm/s+75 μm (No.1), the material achieves tensile strength, yield strength, and elongation values of 351 MPa, 294 MPa, and 4.2%, respectively (Fig.4). Additionally, in the as-built state, the primary microstructure of the composite consists of α-Al phases and TiB2 particles. After heat treatment, a significant number of secondary phases precipitate from the matrix, and SEM results show the presence of abundant precipitates in the forms of both particles and elongated phases (Fig.6).
The addition of TiB2 particles significantly suppresses the cracking of 7050 alloys through the LPBF process and exhibits favorable formability. The optimal process parameters in the as-built state are 240 W+450 mm/s+75 μm, which result in the highest strength of the TiB2/7050 composite. The tensile strength, yield strength, and elongation are 286 MPa, 250 MPa, and 2.3%, respectively. The microstructure of the TiB2/7050 composite after heat treatment consists of fine-sized equiaxed grains. The grain size is concentrated in the range of 1?3 μm, with TiB2 particles evenly dispersed in the grain boundaries and within the equiaxed grain structure, effectively promoting the formation of heterogeneously nucleated refined grains. After heat treatment, the tensile strength, yield strength, and elongation of the specimens of TiB2/7050 composite are 351 MPa, 294 MPa, and 4.2%, respectively.
1 引言
激光粉末床熔化(LPBF)属于金属增材制造技术,利用高功率激光熔化粉末材料[1-3],通过逐层堆叠打印,可以制造形状复杂且集结构与功能于一体的构件,与传统铸造技术相比,具有独特的优点[4-6]。然而,LPBF工艺能加工的金属材料种类非常有限。目前LPBF成形的铝合金主要集中在Al-Si系,而关于其他铝合金的研究报道相对较少[7-8]。所以研究其他传统铝合金材料的打印性能以及设计新的铝合金材料成分很有必要[9]。
7xxx系铝合金具有极强的力学性能及耐磨性能,被广泛应用在汽车等领域中[10]。而7050铝合金是具有代表性的7xxx系铝合金之一,它具有较高的抗拉强度及较强的抗应力腐蚀性能,被应用在飞机舱壁等长期服役的环境中[11]。相较于传统的铸造、轧制等制备方法,使用LPBF技术制备7050铝合金复杂零部件具有更大的优势。然而,7050铝合金具有本征不可焊性,而增材制造过程中的快速冷却会产生高热应力,故其在LPBF制备过程中会发生开裂或变形,这限制了LPBF技术在该合金制备中的应用[12-13]。目前关于7xxx系铝合金的研究工作主要集中在7075铝合金方面[14-18],同时也有少量关于7050铝合金的研究[19-20]。2017年,Martin等[17]使用静电组装技术制备出均匀分布的颗粒增强7075高强铝合金粉体。在这种粉体中,ZrH2纳米颗粒作为异质形核质点均匀地分布在7075高强铝合金粉体的表面上,起到了细化晶粒的作用。由于柱状晶转变为各方向上尺寸相差较小的等轴晶,7075高强铝合金的热裂敏感性降低,抗热裂性能增强,从而实现了LPBF成形无裂纹的目标。此外,詹强坤等[21]使用低能球磨法制备了ZrH2/7075复合粉末,并研究了ZrH2纳米颗粒对试样的显微组织和力学性能的影响。同样,Mertens等[19]在7050铝合金中添加了质量分数为1%的Zr,经过固溶处理和时效处理后,该材料的抗拉强度超过400 MPa。Zr元素的添加可以细化铝合金的晶粒并且降低材料的热裂倾向,从而提高LPBF的成形质量,达到增强原有合金力学性能的目的。但是,此方法产生的细化晶粒有限,且初生的Al3Zr相在激光成形过程中会发生熔解,无法起到形核质点的作用[22]。此外,Li等[20]将Ti/TiN混合晶粒细化剂添加到LPBF成形的7050铝合金中,获得了平均尺寸为775 nm的超细晶粒。相较于7075铝合金,7050铝合金中的Zn和Cu含量发生变化,而这两种元素含量的变化都会使7050铝合金的熔点发生改变,最终影响LPBF过程中该合金的裂纹量,增加了打印难度。因此,需要采用适当的激光工艺参数,确保7050铝合金能够完全熔化,并尽可能减少热影响区域中产生的裂纹和变形。
本文采用LPBF成形TiB2/7050复合材料,后续采用热处理(固溶处理+时效处理)工艺处理材料,研究了不同工艺参数及热处理状态对TiB2/7050复合材料的微观组织和室温拉伸性能的影响,并得到了无裂纹且力学性能良好的LPBF成形TiB2/7050复合材料。
2 材料与实验设备
采用原位自生混合盐法制备含有7%(质量分数)TiB2的7050铝合金铸锭。采用气雾化制粉工艺,得到粒径为15~53 μm的TiB2/7050复合材料粉末[23]。电感耦合等离子体(ICP)检测结果如
表 1. TiB2/7050复合材料粉末及LPBF成形试样的化学成分
Table 1. Chemical compositions of TiB2/7050 composite powder and LPBF formed sample
|

图 1. TiB2/7050复合材料粉末形貌、打印试样块及拉伸试样尺寸。(a)粉末形貌;(b)不同的打印样块;(c)LPBF成形的尺寸为60 mm×20 mm×10 mm的样块;(d)拉伸试样尺寸示意图
Fig. 1. TiB2/7050 composite powder morphology, print sample blocks, and tensile sample size. (a) Powder morphology; (b) different print sample blocks; (c) LPBF shaped sample block with size of 60 mm×20 mm×10 mm; (d) schematic of tensile specimen size
本文采用的热处理为单级固溶处理+时效处理,首先将样品在475 ℃固熔炉中放置1 h,然后放到室温水中进行冷却,再在120 ℃的时效炉中放置12 h,最终在空气中冷却。室温拉伸实验在拉伸设备中进行,其中应变速率采用10-4 s-1。对三个同等状态的拉伸试样进行测试,结果取平均值。采用X射线衍射仪分析样品的物相组成,衍射角度在20°~120°范围内,扫描速率为2(°)/min。微观组织表征采用扫描电子显微镜(SEM),并利用能谱仪(EDS)分析材料表面的元素组成。采用三离子束切割仪,通过机械研磨和离子抛光,制备电子背散射衍射(EBSD)样品。使用配有EBSD检测器的SEM对样品进行显微结构分析,其中EBSD分析采用1.8 μm的步长,EBSD菊池花样使用Aztec晶体软件包进行处理。
3 分析与讨论
3.1 试样块的微观组织
采用阿基米德排水法测量40组不同打印参数下的TiB2/7050复合材料试样的相对密度,结果如
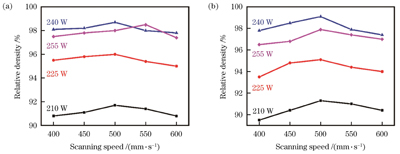
图 2. 不同扫描间距下样块的相对密度。(a)扫描间距为75 μm;(b)扫描间距为90 μm
Fig. 2. Relative density values of samples measured under different scanning spacings. (a) Scanning spacing of 75 μm; (b) scanning spacing of 90 μm
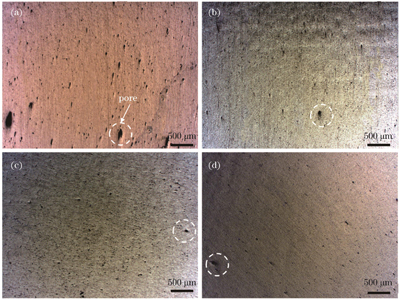
图 3. 不同工艺参数下的TiB2/7050截面金相组织。(a)No.1;(b)No.2;(c)No.3;(d)No.4
Fig. 3. Metallographic structures of TiB2/7050 sections under different process parameters. (a) No.1; (b) No.2; (c) No.3; (d) No.4
3.2 试样块的力学性能及分析
在上述四组参数下,利用LPBF成形尺寸为60 mm×20 mm×12 mm的样块,并对沉积态和热处理态试样进行室温拉伸实验,试样的抗拉强度、屈服强度及延伸率如
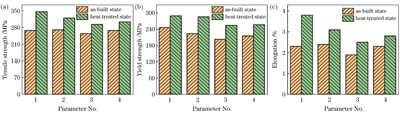
图 4. 沉积态和热处理态LPBF成形TiB2/7050复合材料的力学性能。(a)抗拉强度;(b)屈服强度;(c)延伸率
Fig. 4. Mechanical properties of as-built and heat-treated TiB2/7050composites formed by LPBF. (a) Tensile strength; (b) yield strength; (c) elongation
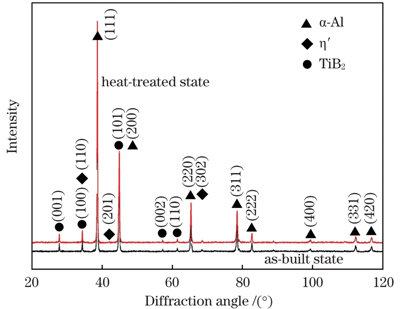
图 5. 沉积态和热处理态TiB2/7050复合材料的XRD谱
Fig. 5. XRD patterns of as-built and heat-treated TiB2/7050 composites
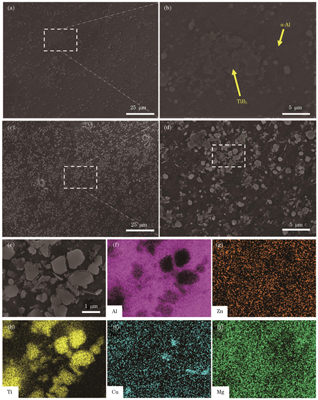
图 6. 沉积态和热处理态TiB2/7050复合材料的SEM图像和EDS结果。(a)(b)沉积态和(c)~(e)热处理态TiB2/7050复合材料的SEM图像;沉积态及热处理态TiB2/7050复合材料中的(f)Al、(g)Zn、(h)Ti、(i)Cu、(j)Mg的EDS结果
Fig. 6. SEM images and EDS results of as-built and heat-treated TiB2/7050 composites. SEM images of (a)(b) as-built and (c)‒(e) heat-treated TiB2/7050 composites; EDS results of (f) Al, (g) Zn, (h) Ti, (i) Cu, and (j) Mg in as-built and heat-treated TiB2/7050 composites
TiB2/7050复合材料(TiB2颗粒的质量分数为7%)采用LPBF成形工艺,由于颗粒的存在以及较高的冷却速率,在LPBF过程中能够诱发大量位错的生成,在拉伸过程中发生位错的塞积,从而提高材料的强度。位错强化(
式中:
式中:
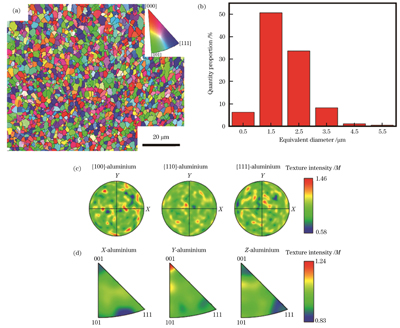
图 7. LPBF成形 TiB2/7050复合材料热处理态的EBSD图像、晶粒尺寸分布、极图和反极图。(a)EBSD图像;(b)晶粒尺寸分布图;(c)极图;(d)反极图
Fig. 7. EBSD image, grain size distribution, and pole and inverse pole images of heat-treated TiB2/7050 composites formed by LPBF. (a) EBSD image; (b) grain size distribution; (c) pole image; (d) inverse pole image
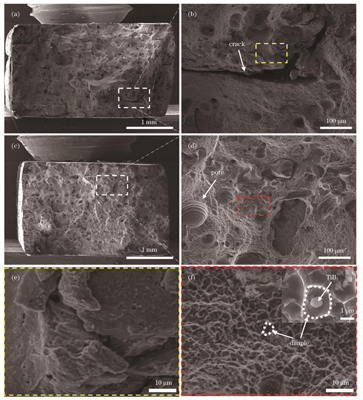
图 8. 沉积态和热处理态TiB2/7050复合材料的拉伸断口形貌。(a)(b)沉积态;(c)(d)热处理态;(e)图8(b)虚线框处的放大结果;(f)图8(d)虚线框处的放大结果
Fig. 8. Tensile fracture morphologies of as-built and heat-treated TiB2/7050 composites. (a)(b) As-built state; (c)(d) heat-treated state; (e) enlarged result at dotted box in Fig. 8 (b); (f) enlarged result at dotted box in Fig. 8 (d)
4 结论
通过在7050合金中引入原位自生纳米TiB2颗粒,显著抑制了LPBF成形7050铝合金的裂纹产生,材料表现出较好的成形性。在240 W+450 mm/s+75 μm(No.1)打印参数下TiB2/7050复合材料的强度最高,其抗拉强度、屈服强度及延伸率分别为286 MPa、250 MPa和2.3%。进一步对TiB2/Al-Cu-Mg复合材料(TiB2的质量分数为4%)进行后热处理研究,通过调控析出相的尺寸和数量,获得了具有较高强度和伸长率的增材制造复合材料。LPBF成形的TiB2/7050复合材料经热处理后,其抗拉强度、屈服强度和伸长率分别为351 MPa、294 MPa和4.2%。研究结果为增材制造纳米颗粒增强铝基复合材料及其后处理提供了理论参考。
[1] Wang A, Wang H Z, Wu Y, et al. 3D printing of aluminum alloys using laser powder deposition: a review[J]. The International Journal of Advanced Manufacturing Technology, 2021, 116(1/2): 1-37.
[2] Wei M W, Chen S Y, Xi L Y, et al. Selective laser melting of 24CrNiMo steel for brake disc: fabrication efficiency, microstructure evolution, and properties[J]. Optics & Laser Technology, 2018, 107: 99-109.
[3] Mu J R, Sun T T, Leung C L A, et al. Application of electrochemical polishing in surface treatment of additively manufactured structures: a review[J]. Progress in Materials Science, 2023, 136: 101109.
[4] 孙腾腾, 王洪泽, 吴一, 等. 原位自生2%TiB2颗粒对2024Al增材制造合金组织和力学性能的影响[J]. 金属学报, 2023, 59(1): 169-179.
Sun T T, Wang H Z, Wu Y, et al. Effect of in situ 2%TiB2 particles on microstructure and mechanical properties of 2024Al additive manufacturing alloy[J]. Acta Metallurgica Sinica, 2023, 59(1): 169-179.
[5] Gao Z Y, Wang H Z, Letov N, et al. Data-driven design of biometric composite metamaterials with extremely recoverable and ultrahigh specific energy absorption[J]. Composites Part B: Engineering, 2023, 251: 110468.
[6] 郭朦, 戴延丰, 黄斌达. 典型航空机电产品激光粉末床熔融技术应用现状与发展[J]. 中国激光, 2023, 50(16): 1602304.
[7] Karolus M, Maszybrocka J, Stwora A, et al. Residual stresses of AlSi10Mg fabricated by selective laser melting (SLM)[J]. Archives of Metallurgy and Materials, 2029, 64: 1011-1016.
[8] 赵钰涣, 龙芋宏, 徐榕蔚, 等. 7075铝合金选区激光熔化研究现状[J]. 现代制造工程, 2021(9): 146-151.
Zhao Y H, Long Y H, Xu R W, et al. Research status of selective laser melting in 7075 aluminum alloy[J]. Modern Manufacturing Engineering, 2021(9): 146-151.
[9] DebRoy T, Wei H L, Zuback J S, et al. Additive manufacturing of metallic components-process, structure and properties[J]. Progress in Materials Science, 2018, 92: 112-224.
[10] Liu P, Hu J Y, Li H X, et al. Effect of heat treatment on microstructure, hardness and corrosion resistance of 7075 Al alloys fabricated by SLM[J]. Journal of Manufacturing Processes, 2020, 60: 578-585.
[11] Otani Y, Sasaki S. Effects of the addition of silicon to 7075 aluminum alloy on microstructure, mechanical properties, and selective laser melting processability[J]. Materials Science and Engineering: A, 2020, 777: 139079.
[12] Zhou S Y, Su Y, Wang H, et al. Selective laser melting additive manufacturing of 7xxx series Al-Zn-Mg-Cu alloy: cracking elimination by co-incorporation of Si and TiB2[J]. Additive Manufacturing, 2020, 36: 101458.
[13] 沈君剑, 刘允中, 欧阳盛, 等. 激光选区熔化成形TiB2与SiC颗粒混杂增强铝基复合材料的显微组织与力学性能[J]. 粉末冶金材料科学与工程, 2020, 25(3): 251-259.
Shen J J, Liu Y Z, Ouyang S, et al. Microstructures and mechanical properties of aluminum matrix composites reinforced with hybrid TiB2 and SiC prepared by selective laser melting[J]. Materials Science and Engineering of Powder Metallurgy, 2020, 25(3): 251-259.
[14] Tan Q Y, Fan Z Q, Tang X Q, et al. A novel strategy to additively manufacture 7075 aluminium alloy with selective laser melting[J]. Materials Science and Engineering: A, 2021, 821: 141638.
[15] Yi J L, Chang C, Yan X C, et al. A novel hierarchical manufacturing method of the selective laser melted Al 7075 alloy[J]. Materials Characterization, 2022, 191: 112124.
[16] Hu J Y, Liu P, Sun S Y, et al. Relation between heat treatment processes and microstructural characteristics of 7075 Al alloy fabricated by SLM[J]. Vacuum, 2020, 177: 109404.
[17] Martin J H, Yahata B D, Hundley J M, et al. 3D printing of high-strength aluminium alloys[J]. Nature, 2017, 549(7672): 365-369.
[18] 吕新蕊, 刘婷婷, 廖文和, 等. 高强铝合金7075激光粉末床熔融凝固裂纹的消除与质量控制[J]. 中国激光, 2022, 49(14): 1402209.
[19] Mertens R, Baert L, Vanmeensel K, et al. Laser Powder bed fusion of high strength aluminum[J]. Material Design & Processing Communications, 2021, 3(5): 161.
[20] Li X W, Li G, Zhang M X, et al. Novel approach to additively manufacture high-strength Al alloys by laser powder bed fusion through addition of hybrid grain refiners[J]. Additive Manufacturing, 2021, 48: 102400.
[21] 詹强坤, 刘允中, 刘小辉, 等. 激光选区熔化成形含锆7xxx系铝合金的显微组织与力学性能[J]. 材料工程, 2021, 49(6): 85-93.
Zhan Q K, Liu Y Z, Liu X H, et al. Microstructures and mechanical properties of zirconium-containing 7xxx aluminum alloy prepared by selective laser melting[J]. Journal of Materials Engineering, 2021, 49(6): 85-93.
[22] Zhou L, Pan H, Hyer H, et al. Microstructure and tensile property of a novel AlZnMgScZr alloy additively manufactured by gas atomization and laser powder bed fusion[J]. Scripta Materialia, 2019, 158: 24-28.
[23] Sun T T, Chen J, Wu Y, et al. Achieving excellent strength of the LPBF additively manufactured Al-Cu-Mg composite viain situ mixing TiB2 and solution treatment[J]. Materials Science and Engineering: A, 2022, 850: 143531.
[24] Zheng T Q, Pan S H, Murali N, et al. Selective laser melting of novel 7075 aluminum powders with internally dispersed TiC nanoparticles[J]. Materials Letters, 2022, 319: 132268.
[25] Liu Y X, Wang R C, Peng C Q, et al. Microstructural evolution and mechanical performance of in situ TiB2/AlSi10Mg composite manufactured by selective laser melting[J]. Journal of Alloys and Compounds, 2021, 853: 157287.
[26] Mason C J T, Rodriguez R I, Avery D Z, et al. Process-structure-property relations for as-deposited solid-state additively manufactured high-strength aluminum alloy[J]. Additive Manufacturing, 2021, 40: 101879.
[27] 欧阳盛, 刘允中, 沈君剑, 等. (TiH2+TiB2)/AA7075复合粉末激光选区熔化成形的显微组织与力学性能[J]. 粉末冶金材料科学与工程, 2020, 25(3): 197-205.
Ouyang S, Liu Y Z, Shen J J, et al. Microstructure and mechanical properties of selective laser melting of (TiH2+TiB2)/AA7075 composite powders[J]. Materials Science and Engineering of Powder Metallurgy, 2020, 25(3): 197-205.
[28] Yan S J, Wang R C, Peng C Q, et al. Enhancing mechanical properties of 7055 Al alloy by adding 1 wt.% Mg and tailoring the precipitations[J]. Journal of Alloys and Compounds, 2023, 947: 169536.
[29] Ma K K, Wen H M, Hu T, et al. Mechanical behavior and strengthening mechanisms in ultrafine grain precipitation-strengthened aluminum alloy[J]. Acta Materialia, 2014, 62: 141-155.
[30] Zhang J L, Song B, Yang L, et al. Microstructure evolution and mechanical properties of TiB/Ti6Al4V gradient-material lattice structure fabricated by laser powder bed fusion[J]. Composites Part B: Engineering, 2020, 202: 108417.
[31] Hooper P A. Melt pool temperature and cooling rates in laser powder bed fusion[J]. Additive Manufacturing, 2018, 22: 548-559.
[32] Liu G, Geng J W, Li Y G, et al. Microstructures evolution of nano TiB2/7050 Al composite during homogenization[J]. Materials Characterization, 2020, 159: 110019.
[33] Chai H W, Fan D, Yuan J C, et al. Deformation dynamics of a neutron-irradiated aluminum alloy: an in situ synchrotron tomography study[J]. Acta Materialia, 2023, 243: 118493.
[34] 石岩, 魏登松. 激光粉末床熔融增材制造未熔合气孔缺陷形成机理研究[J]. 中国激光, 2023, 50(20): 2002303.
[35] Wang Y F, Toda H, Xu Y T, et al. In-situ 3D observation of hydrogen-assisted particle damage behavior in 7075 Al alloy by synchrotron X-ray tomography[J]. Acta Materialia, 2022, 227: 117658.
[36] Croom B P, Jin H, Noell P J, et al. Collaborative ductile rupture mechanisms of high-purity copper identified by in situ X-ray computed tomography[J]. Acta Materialia, 2019, 181: 377-384.
[37] 宋长辉, 付厚雄, 严仲伟, 等. 激光粉末床熔融成形内部质量缺陷及其调控方法[J]. 中国激光, 2022, 49(14): 1402801.
Article Outline
王安, 唐梓珏, 吴一, 王浩伟, 王洪泽. 激光粉末床熔化制备TiB2颗粒增强7050铝基复合材料的组织及性能研究(特邀)[J]. 中国激光, 2024, 51(4): 0402304. An Wang, Zijue Tang, Yi Wu, Haowei Wang, Hongze Wang. Microstructure and Properties of 7050 Aluminum Matrix Composites Reinforced by TiB2 Particles Fabricated Through Laser Powder Bed Fusion (Invited)[J]. Chinese Journal of Lasers, 2024, 51(4): 0402304.