光纤中无波分裂脉冲的平坦超连续谱产生
0 引 言
被动锁模光纤激光器因其高稳定性和紧凑的设计而受到广泛关注[1],其中,如何提高脉冲能量和峰值功率一直是研究热点。因为许多应用需要具有高脉冲能量和高峰值功率的孤子脉冲,例如超连续谱产生、材料处理和手术医疗等。然而,过度非线性相移的积累会导致脉冲分裂,这是限制脉冲能量的主要因素[2]。通常,传统孤子的脉冲啁啾很小,脉冲分裂阈值较低,在极大的泵浦功率下容易产生波分裂[3]。近年来,耗散孤子共振脉冲引起了人们的广泛关注。它的脉冲啁啾随着泵浦强度线性增加,这可以有效地避免波分裂[4]。然而,它的脉冲宽度通常在纳秒量级,导致低峰值功率。耗散孤子可以积累大量啁啾提高脉冲分裂阈值,同时脉冲宽度可以压缩到飞秒量级[5]。为此,人们提出了各种结构和方案来提高耗散孤子的脉冲能量。
最近,Wang等人使用单壁碳纳米管作为锁模结构来获得高能量的耗散孤子脉冲[6]。然而,单壁碳纳米管的低损耗阈值、窄波长工作范围和复杂的制备工艺限制了其应用。Szczepanek等人报告了使用非线性极化演化(Nonlinear Polarization Evolution, NPE)锁模方法获得高能量脉冲[7]。虽然,NPE可以抵抗光纤腔内的高功率,但其缺点是成本高,光纤腔拼接困难。相比于其他锁模结构,非线性偏振旋转(Nonlinear Polarization Rotation, NPR)结构简单,成本低,波长可调谐范围大,输出光谱宽,输出功率高[8]。笔者采用 NPR作为锁模结构实现了耗散孤子和无波分裂脉冲的切换输出。与耗散孤子脉冲相比,无波分裂脉冲的脉冲能量可以提高到3.89 nJ。
当光脉冲被注入到高非线性光纤中,其频谱受到非线性效应诸如自相位调制、交叉相位调制、四波混频和受激拉曼散射的影响,使脉冲频谱内产生新的频率分量[9]。频谱会被极大地被展宽,这种被展宽的频谱被称为超连续谱。超连续谱在实际应用中可以作为宽带光源测量光纤光栅的反射率和透射率,也被应用于医学、计量学等领域[10-11]。经报道传统孤子、类噪声脉冲、耗散孤子脉冲、束缚态脉冲均可以在高非线性光纤中产生超连续谱[12-14],然而针对于可切换的高能量脉冲在微纳光纤中产生超连续谱的报道较少。笔者注入耗散孤子和无波分裂脉冲分别进入拉锥后的高非线性光纤获得超连续谱,观察到无波分裂脉冲产生的超连续谱宽度和平坦度都优于耗散孤子脉冲。文中利用无波分裂脉冲产生的平坦超连续谱对多信道波分复用光源应用领域的研究有重要意义。
1 实验结构
被动锁模激光器的示意图如图1所示。两个976 nm泵浦源通过两个980/1550 nm波分复用器(Wavelength Division Multiplexer, WDM)泵浦一段4 m长的掺铒光纤(Erbium-Doped Fiber, EDF)产生1550 nm激光。两个偏振控制器(Polarization Controller, PC)和一个偏振相关隔离器(Polarization Dependent Isolator, PD-ISO)一起构成NPR结构周期地调整腔内损耗产生锁模脉冲。采用10∶90耦合器(Optical Coupler, OC)输出激光。输出后的脉冲先经过单模光纤压缩,再经过掺铒光纤放大器(Erbium-Doped Fiber Amplifier, ED-FA)放大,最后注入拉锥后的高非线性光纤(High Non-linear Fiber, HNLF)产生超连续谱。通过合理安排谐振腔内色散补偿光纤(Dispersion Compensation Fiber, DCF)和单模光纤(Single-Mode Fiber,SMF)的长度,可以控制腔的净色散。其中,掺铒光纤、单模光纤和色散补偿光纤的群速度色散为+0.0535、−0.0229、+0.1848 ps2/m。所有器件的尾纤均为标准单模光纤长度为1 m。色散补偿光纤和单模光纤的长度分别为1 m和5.05 m。因此,总腔长约为11.05 m。计算出腔内的净色散为+0.260255 ps2。最后,输出激光通过最小分辨率为0.05 nm的光谱分析仪(OSA, Yokogawa, AQ6375)测量光谱,输出光脉冲通过带宽为10 GHz的1.55 μm光电探测器进行光电转换,然后进入带宽为2.5 GHz的示波器(OSC, Agilent, DSO-X93204A)采集脉冲序列,其中射频频谱由频率范围为3 Hz~44 GHz的射频信号分析仪(Agilent, DSO-X93204A)探测,由于示波器的探测极限不足以精确地分辨小于50 ps的窄脉冲宽度,因此,脉冲的真实宽度由自相关仪(FR-103XL)记录。
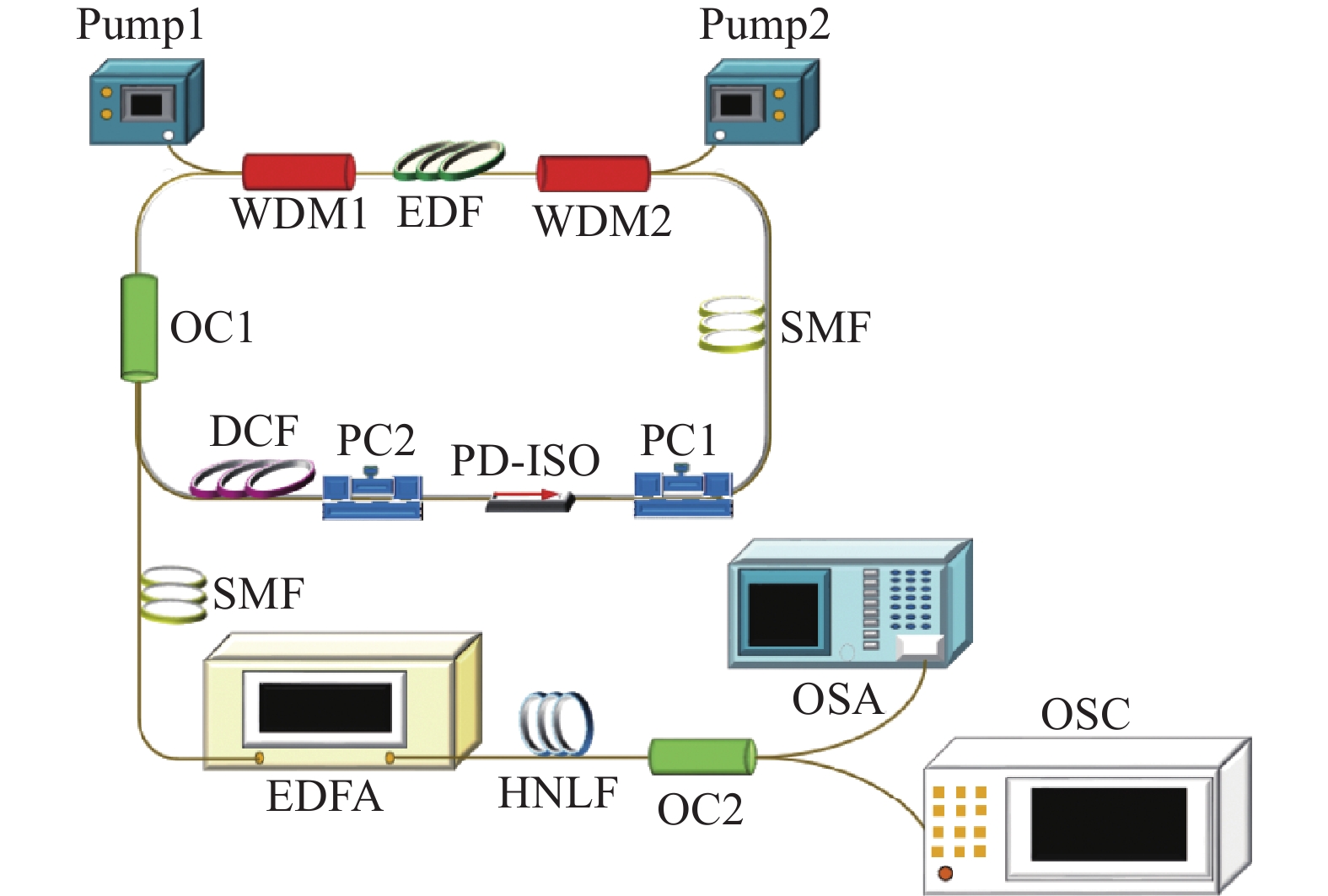
图 1. 被动锁模激光器原理图。WDM:波分复用器;EDF:掺铒光纤;OC:10∶90光耦和器;PC1和PC2:偏振控制器;PD-ISO:偏振相关隔离器;SMF:单模光纤; DCF:色散补偿光纤;EDFA:掺铒光纤放大器;HNLF:高非线性光纤;OSA:光谱分析仪;OSC:示波器
Fig. 1. Schematic diagram of passive mode-locked laser. WDM: wavelength division multiplexer; EDF: erbium-doped fiber; OC: 10∶90 optical coupler; PC1 and PC2: polarization controller; PD-ISO: polarization dependent isolator; SMF: single-mode fiber; DCF: dispersion compensation fiber; EDFA: erbium-doped fiber amplifier; HNLF: high nonlinear fiber; OSA: optical spectrum analyzer; OSC: oscilloscope
锥形光纤的结构如图2(a)所示,将一根标准的HNLF熔融拉锥后形成的锥形光纤拥有锥形过渡区、腰区两部分,这两个部分均用红色线条在图中标记出来了。可以看见除了光纤的包层,光纤的纤芯部分也会随着拉锥的长度增加而变细。与普通HNLF相比,锥形HNLF的纤芯直径约为6 μm。因此,锥形HNLF的非线性更强,有利于超连续谱的产生。实验中HNLF的数值孔径为0.35,传输损耗小于1.5 dB/km,长度为4 m,腰区为1 m,锥区为3 m。在锥形光纤的制作过程中利用氢氧火焰拉锥机制备,拉锥后的HNLF实物图如图2(b)所示。

图 2. (a) 锥形光纤结构图;(b) HNLF熔融拉锥实物图
Fig. 2. (a) Structure diagram of tapered optical fiber; (b) Physical drawing of tapered HNLF
2 结果与讨论
打开泵浦2,逐渐将功率增加到0.3 W,光谱从连续光变为锁模光。如图3(a)所示,光谱形状类似于一个矩形,中心波长为1560 nm,3 dB带宽为21 nm,这是耗散孤子(Dissipative Soliton, DS)的典型特征。时域图如图3(b)所示。脉冲间隔为54 ns,符合基频运转。图3(c)显示了30 MHz范围内的射频频谱,分辨率带宽为500 Hz。重复频率为18.5 MHz时,信噪比为55 dB。稳定锁模状态可以通过图3(c)中插入的300 MHz宽范围射频频谱来确认。由sech2函数拟合的脉冲自相关轨迹如图3(d)所示。自相关记录的半高全宽为8.9 ps,对应于5.8 ps的脉冲宽度。经计算,时间带宽乘积为15,表明输出脉冲具有较大的啁啾。将泵浦2固定在0.3 W,并将泵浦1从0.2 W增加到0.7 W,耗散孤子始终可以在示波器上保持基频运行,平均输出功率从5 mW增加到15.2 mW。脉冲能量和峰值功率可分别从0.27 nJ提高到0.82 nJ,和从41 W提高到124.4 W。
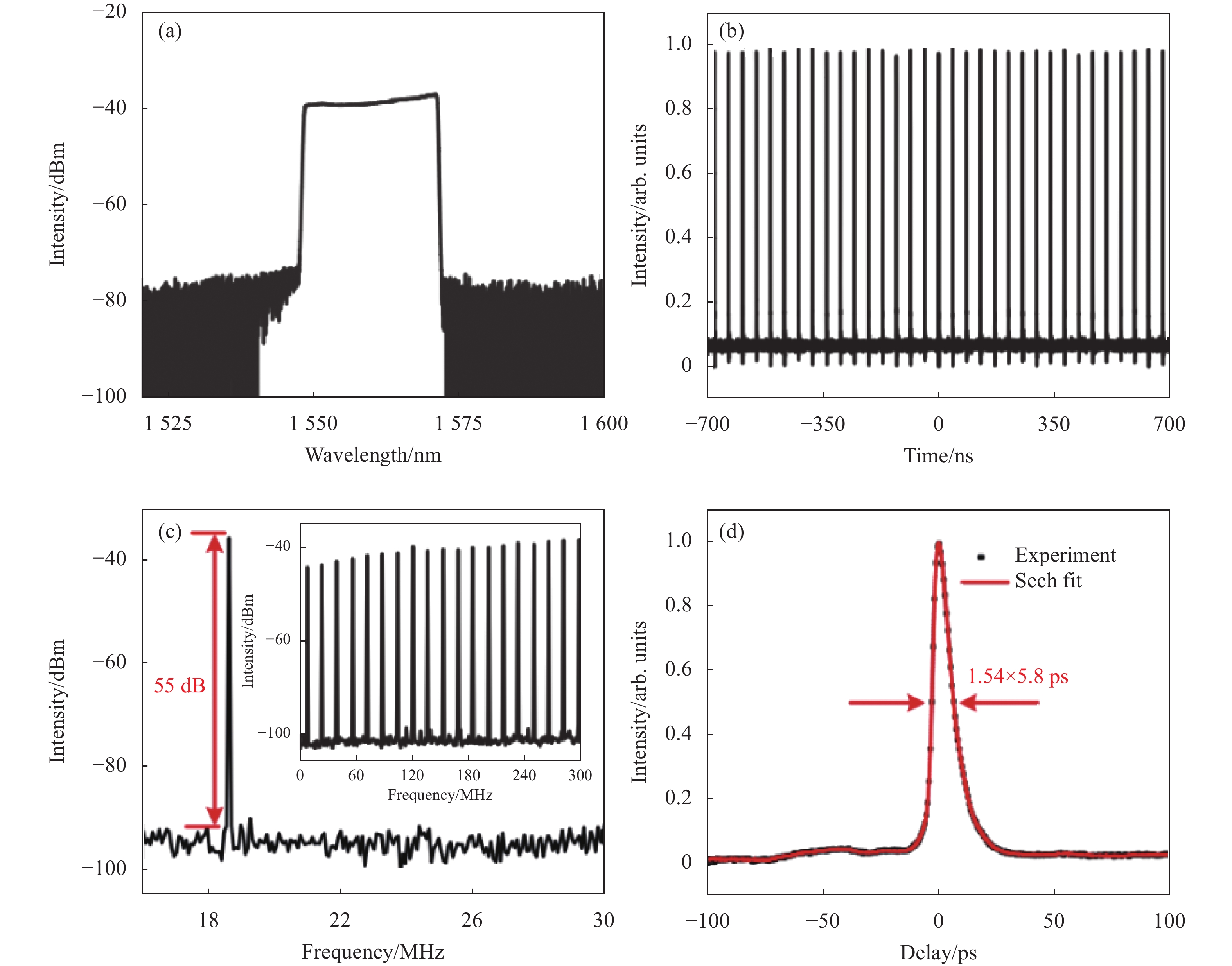
图 3. 锁模激光器运转在正常色散区。(a) 光谱;(b) 时域图;(c) 射频频谱,插图(射频频谱范围0~300 MHz); (d) 脉冲的自相关轨迹(黑色点),双曲正割拟合(红色曲线)
Fig. 3. Mode-locked laser operates in the normal dispersion region. (a) Optical spectrum; (b) Time-domain diagram; (c) RF spectrum, insert (range of RF spectrum in 300 MHz); (d) Autocorrelation trace of pulse (black point), Sech2 fit (red curve)
当泵浦1功率超过0.7 W时,可以观察到耗散孤子脉冲运转不能在单脉冲下稳定,图4(a)所示为在780 mW泵浦功率下的时域图,受峰值功率钳制效应的影响,在示波器上由单脉冲锁模变成多脉冲锁模。根据时间带宽积理论,通过在腔外添加11 m长的单模光纤,可以将耗散孤子脉冲压缩到0.61 ps,见图4(b),经计算,峰值功率被提升到1.18 kW。
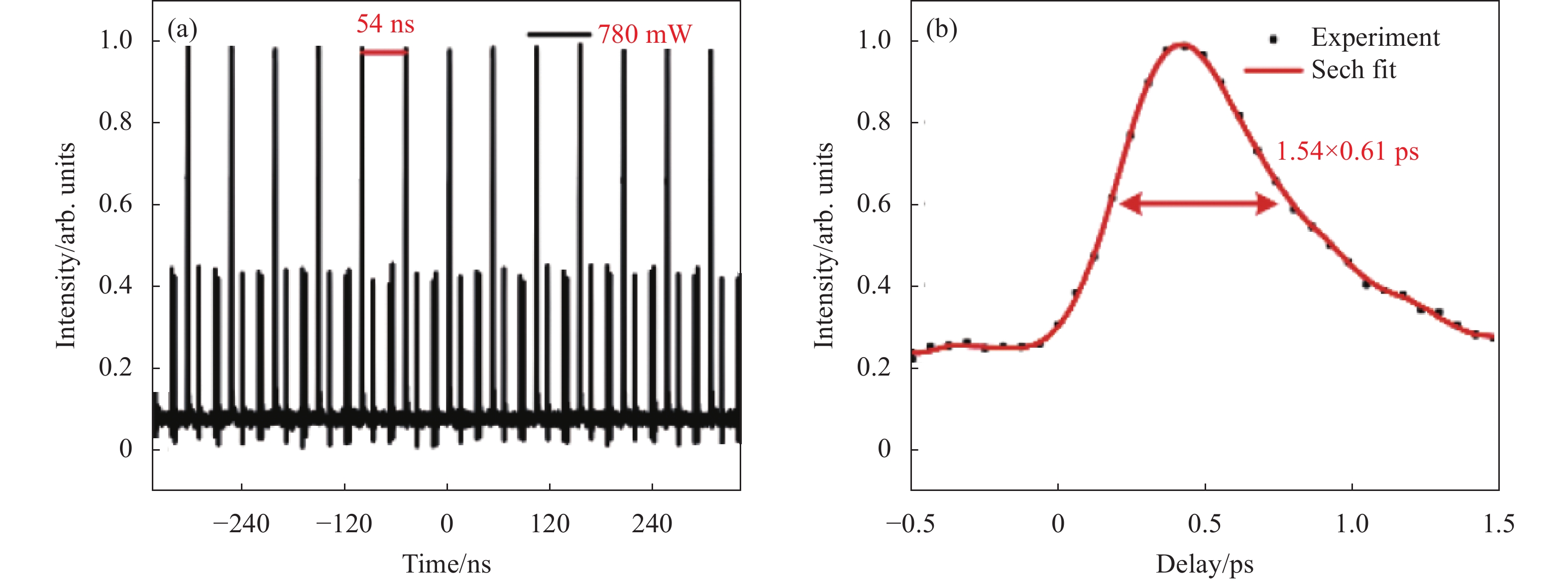
图 4. (a) 时域图;(b) 耗散孤子在11 m长单模光纤传输的自相关轨迹
Fig. 4. (a) Time-domain diagram; (b) Autocorrelation trace of dissipative soliton in 11 m single-mode fiber
在0.8 W的泵浦1功率下,通过仔细调整偏振控制器,耗散孤子的光谱变得更宽更平滑,如图5(a)所示。中心波长为1559 nm,3 dB带宽为16.54 nm。锁模脉冲序列如图5(b)所示。脉冲间隔为54 ns,与脉冲在腔内往返一周的时间一致。重复频率为18.5 MHz时,信噪比为50 dB,见图5(c)。图5(c)的插图表示0~300 MHz的宽范围射频频谱,表明脉冲序列具有良好的稳定性。为了验证脉冲类型,对输出的锁模脉冲进行自相关测试。图5(d)是高斯函数拟合的脉冲自相关轨迹。输出脉冲的半高全宽为16.5 ps,对应的脉冲宽度为11.7 ps。经计算,时间带宽积为23.9表示输出脉冲带有大的啁啾。

图 5. (a) 耗散孤子光谱的演变;(b) 时域图;(c) 射频频谱,插图(0~300 MHz范围内射频频谱);(d) 演化脉冲的自相关轨迹(黑色),高斯拟合(红色)
Fig. 5. (a) Evolution of DS spectrum; (b) Time-domain diagram; (c) RF spectrum, insert (0-300 MHz span range of PF spectrum); (d) Autocorrelation trace of evolution pulse (black), Gaussian fit (red)
将泵浦1功率从0.8 W增加到1 W,光谱形状没有明显变化,如图6(a)所示,并且,在示波器上始终没有观察到脉冲分裂的现象。因此,这个脉冲是无波分裂脉冲又称为高能量脉冲(high-energy pulse)。在泵浦功率为1 W时,如图6(b)所示,自相关仪测量的半高全宽为28.6 ps,在高斯拟合之后对应于20.2 ps的脉冲宽度。脉冲的顶部没有被完全的拟合,这是由于二阶色散和非线性共同引起的瞬时啁啾导致该区域内脉冲能量分布发生剧烈变化,同时,由于光谱展宽,高阶色散也会导致脉冲顶部扭曲。其中,无波分裂脉冲的脉冲啁啾在整个中心区域是小的而且是线性的,在脉冲的头部和尾部都是大的是非线性的[15],因此,脉冲可以抵抗腔中非线性相移的影响,并在高泵浦功率下保持无波分裂运转。随泵浦功率的增加,脉冲宽度从11.7 ps增加到20.2 ps,无波分裂脉冲光谱的中心波长几乎不变,但3 dB带宽从16.54 nm增加到21.43 nm。经计算,时间带宽积可以从23.9增加到53.43。较大的啁啾可以抵抗非线性相移的影响。因此,激光器可以直接输出稳定的高能量脉冲。与耗散孤子脉冲不同,在经过11 m的单模光纤,无波分裂脉冲的脉冲宽度变化并不明显。根据文献[16],无波分裂脉冲包含大量的非线性,很难被线性色散光纤压缩。为了区分耗散孤子和无波分裂脉冲,文中对比了耗散孤子和无波分裂脉冲的输出特性。从图6(c)可以看出,耗散孤子和无波分裂脉冲具有不同的斜率效率和输出功率。将泵浦1功率从0.2 W提高到0.7 W时,耗散孤子单脉冲输出功率从5 mW增加到15.2 mW,将泵浦1功率从0.8 W提高到1 W,无波分裂脉冲输出功率从41 mW增加至124.4 mW。如图6(d)所示,耗散孤子和无波分裂脉冲的最大光转换效率分别为1.52%和5.51%。与耗散孤子运转相比,无波分裂脉冲可以大大提高脉冲能量。无波分裂脉冲的最大脉冲能量为3.89 nJ,几乎是耗散孤子脉冲能量的五倍。同时,无波分裂脉冲的最大脉宽是耗散孤子最大脉宽的四倍。因此,无波分裂脉冲可以累积更多的啁啾避免脉冲分裂。通过提高泵浦功率,耗散孤子的最大峰值功率可以增加到124.4 W,而无波分裂脉冲的峰值功率几乎保持在185.4 W左右。
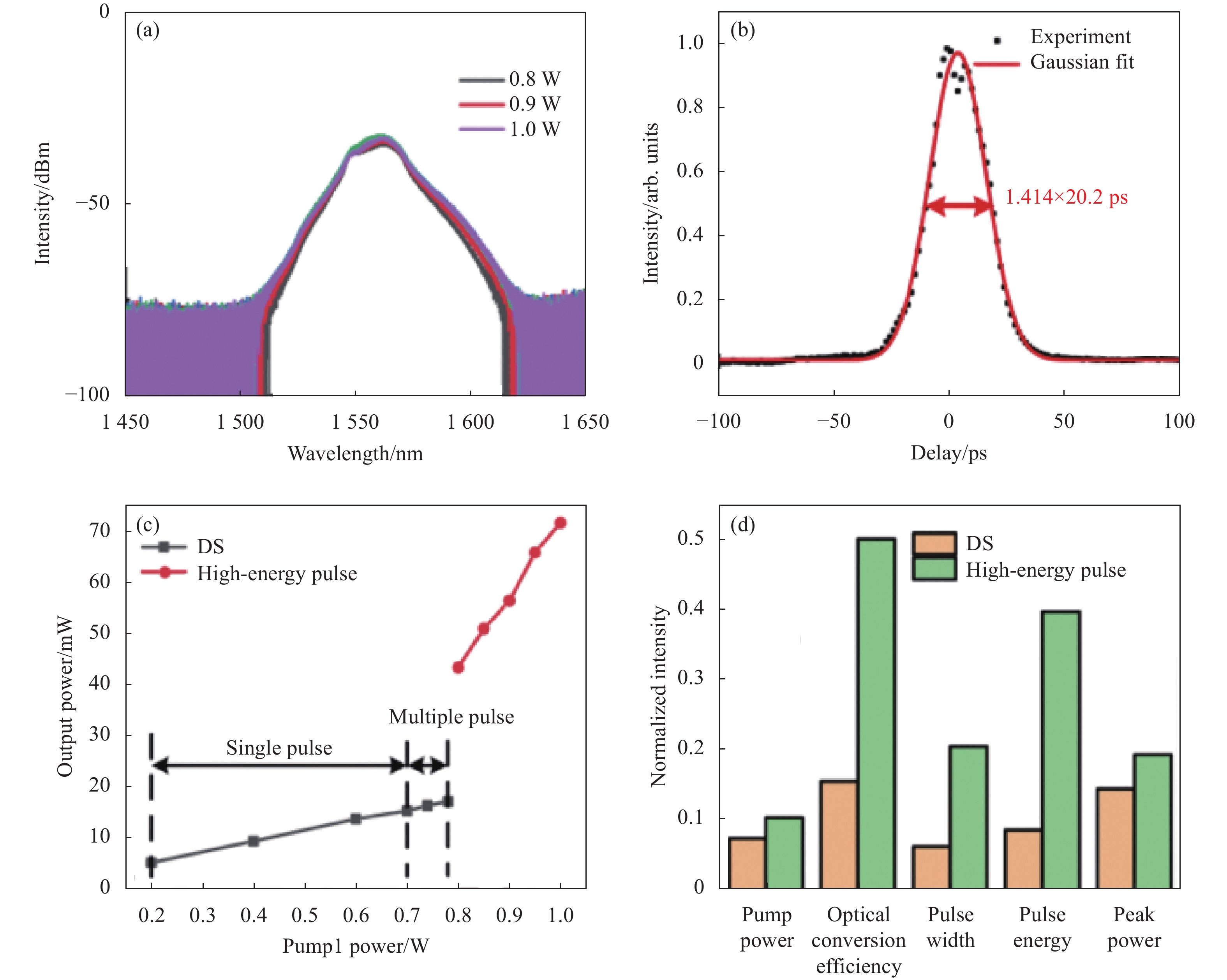
图 6. (a) 光谱随泵浦功率的演化;(b) 泵浦功率为1 W时的自相关轨迹;(c) 比较耗散孤子和无波分裂脉冲输出功率随泵1功率的变化;(d) 比较耗散孤子和无波分裂脉冲最大锁模泵浦功率、光转换效率、脉冲宽度、脉冲能量、峰值功率
Fig. 6. (a) Evolution of optical spectrum with pump power; (b) Autocorrelation trace at the pump power of 1 W; (c) Comparison of pump1 power vs output power between DS and wave-free breaking pulse; (d) Comparing between maximum lock-mode pump power, optical conversion efficiency, pulse width, pulse energy, peak power for DS and wave-free breaking pulse
随后,将掺铒光纤放大器放大的无波分裂脉冲和耗散孤子分别注入4 m长的部分锥形高非线性光纤中,获得超连续谱。耗散孤子和无波分裂脉冲在经过掺铒光纤放大器放大后最大输出功率分别为84 mW和227 mW,对应于4.7 nJ和12.4 nJ的脉冲能量。光谱的频移由
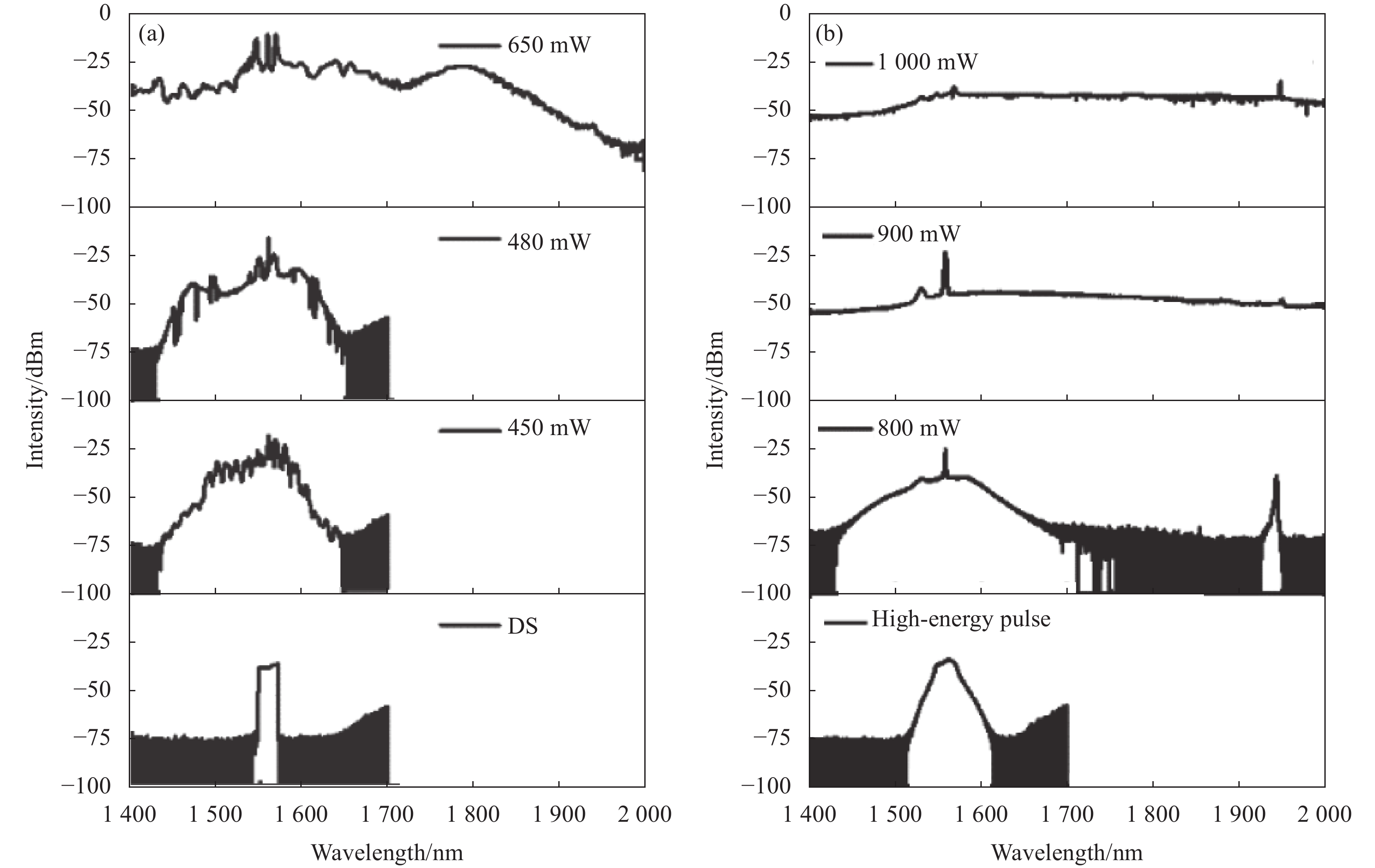
图 7. 超连续谱宽度随泵浦功率增加的演化图。(a)基于耗散孤子产生的超连续谱;(b)基于无波分裂脉冲产生的超连续谱
Fig. 7. Evolution of supercontinuum with the increasing of pump power. (a) Supercontinuum generated by dissipative soliton; (b) Supercontinuum generated by wave-free breaking pulse
3 结 论
文中采用非线性偏振旋转锁模结构实现超快脉冲输出,通过色散管理实现耗散孤子和无波分裂脉冲的转换。在耗散孤子锁模状态下,耗散孤子最大脉冲能量为0.82 nJ,峰值功率为124.4 W。通过调整腔参数和增加泵浦功率,可以实现无波分裂脉冲的锁模状态。无波分裂脉冲的脉冲能量可以增加到3.89 nJ,是耗散孤子脉冲能量的5倍。在锥形HNLF中,使用无波分裂脉冲作为种子源比使用耗散孤子脉冲产生超连续谱的平坦性更好。并且产生的超连续谱范围均覆盖了三个主要的通信波段(S波段、C波段、L波段)。这种平坦超连续谱不仅对多信道波分复用光源应用领域的研究有重要意义,而且这种平坦且覆盖了水分子吸收区的超连续谱光源还可以用于生物医学成像等领域。
[10] 纪海莹, 王天枢, 熊浩, 马万卓, 袁泉, 孙梦茹, 林鹏, et al. 位于第三近红外窗口的平坦光纤超连续谱产生[J]. 应用光学, 2021, 42(3): 565-570.
[11] 高静. 可调谐锁模光纤激光器泵浦的超连续谱光源[J]. 光学精密工程, 2018, 26(1): 25-30.
[13] 邹宝英, 戴佳男, 洪伟毅. 光纤中飞秒双脉冲束缚态产生超连续谱的研究[J]. 中国激光, 2020, 47(7): 0706003.
[14] 李颖奎, 任行, 李鑫, 杜垒, 王天枢, 姜会林, et al. 耗散孤子和束缚态脉冲可切换的超连续谱产生[J]. 红外与激光工程, 2022, 51(7): 20220035.
任行, 王天枢, 杜垒, 李鑫, 肖林, 周见红. 光纤中无波分裂脉冲的平坦超连续谱产生[J]. 红外与激光工程, 2023, 52(5): 20220745. Hang Ren, Tianshu Wang, Lei Du, Xin Li, Lin Xiao, Jianhong Zhou. Flat supercontinuum generation via wave-free breaking pulse in the optical fiber[J]. Infrared and Laser Engineering, 2023, 52(5): 20220745.