基于钬掺杂ZBYA玻璃光纤的中红外激光研究
下载: 1067次
与传统的ZBLAN(ZrF4-BaF2-LaF3-AlF3-NaF)玻璃相比,ZBYA(ZrF4-BaF2-YF3-AlF3)玻璃有着更加良好的热稳定性和化学稳定性。制备出ZBYA玻璃光纤,采用1150 nm拉曼光纤激光器作为泵浦源,使用Ho3+掺杂的ZBYA光纤作为增益介质实现了~2.9 μm波长的激光输出,最大输出功率达到了137 mW,斜率效率为8.9%。研究结果表明ZBYA玻璃光纤是一种潜在的用于实现中红外波段激光的增益材料。
The midinfrared (MIR) lasers at ~2.9 μm have been attracting increasing attention owing to their considerable applications in surgery, military, environmental monitoring, remote sensing, and nonlinear optics. In many ways, fiber laser has unique advantages for achieving MIR laser. In the past few decades, zirconium fluoride glass fiber, represented by ZBLAN (ZrF4-BaF2-LaF3-AlF3-NaF), has been widely used in MIR fiber lasers. However, the poor thermal and chemical stabilities of ZBLAN fibers pose a challenge to achieve higher power MIR lasers. Therefore, in this study, we fabricated ZBYA (ZrF4-BaF2-YF3-AlF3) glass fiber, which is more stable than the ZBLAN fiber, and the Ho3+ -doped ZBYA fiber was used as the gain medium to achieve a 2.9-μm MIR laser under 1150-nm pump. This result indicated that ZBYA glass fiber is a potential gain medium material for MIR fiber lasers.
In this work, the ZBYA glass with low water content was prepared and polished. The transmittance spectrum in the range of 200010000 nm of ZBYA glass was measured using Perkin-Elmer Fourier-transform infrared (FTIR) spectrometer (Fig. 1). Differential scanning calorimetry was used to measure the thermal properties of the core and cladding glass at a heating rate of 10 K/min using a Netzsch STA449F5 analyzer (Fig. 2). By comparing the transmittance changes of the ZBYA glass and ZBLAN glass after soaking them in water for a long period (24 h), it is demonstrated that ZBYA glass has higher chemical stability (Fig. 3). Then, we created the Ho3+ -doped ZBYA glass fiber and constructed a laser system with ZBYA fiber, an 1150-nm fiber laser, a focus lens, and a dichroic mirror (Fig. 5). An output power of ~2.9 μm was measured using a power detector, and the laser spectrum was observed with an optical spectrum analyzer (YOKOGAWA AQ6377) (Figs. 6 and 7).
The transition temperature (Tg) and crystallization temperature of core and cladding glass are 333 ℃ and 334 ℃ and 406 ℃ and 405 ℃, respectively (Fig. 2). The Tg of ZBYA glass is considerably higher than that of ZBLAN glass, indicating that ZBYA glass has a higher laser damage threshold. After soaking in water for 24 h, the transmittance of ZBYA glass was considerably higher than that of ZBLAN glass, demonstrating that ZBYA glass has superior chemical stability (Fig. 3). For ~2.9-μm laser system, a ~2.9-μm laser output is observed when the pump power is 97 mW. The highest output power is 137 mW, the corresponding pump power is 1.5 W, and the slope efficiency is 8.9%. The reason for the slope efficiency being far lower than the Stokes efficiency limit in the experiment is mainly owing to the high background loss of ZBYA fiber (3 dB/m) and the large core diameter (30 μm). The center wavelength of laser output is 2855 nm, and the full width at half maxima is 1.2 nm. As shown in Fig. 8, the stability of the laser output power is monitored. In 30 min, the laser output power gradually decreases from 137 mW to 131 mW. The gradual accumulation of hydroxyl groups at the fiber’s end during laser operation results in a 2.9-μm reduction in laser power; additionally, the laser power does not fluctuate sharply.
In this study, ZBYA glass is prepared and tested and its better thermal and chemical stabilities are demonstrated. Accordingly, we fabricate the Ho3+ -doped ZBYA glass fiber and achieve a ~2.9-μm laser. The maximum output power is 137 mW, and the slope efficiency is 8.9%. Currently, the background of fiber is still high owing to the purity of raw materials; however, by optimizing the preparation process to reduce fiber loss, it is expected to achieve higher output power and slope efficiency. Furthermore, silica fiber and ZBYA fiber’s fusion splicing technology should be investigated to reduce the complexity of laser systems. The future work should focus on the preparation of double-cladding fibers to increase the maximum pump power and obtain higher power output.
1 引言
波长为~2.9 μm的激光在医疗、**、环境监测以及遥感等领域有着广泛的应用,受到了越来越多的关注[1]。目前,产生中红外波段激光的激光器主要有以下几种:半导体激光器、固体激光器、自由电子激光器、化学激光器、气体激光器等[2-6]。这些激光器都各自有着不同的缺点,严重阻碍了高功率中红外激光的研究进展。光纤激光器有着光束质量好、转换效率高、易于散热和易于集成等优点,是一种高效的中红外激光产生方式。
由于石英光纤的声子能量较高(1100 cm-1), 很难应用于产生及传输波长超过2.5 μm的光,因此中红外波段的光纤激光器往往使用低声子能量的氟化物光纤。氟化物光纤主要有氟化锆基玻璃光纤、氟化铝基玻璃光纤以及氟化铟基玻璃光纤,其中,基于氟化锆基玻璃的ZBLAN(ZrF4-BaF2-LaF3-AlF3-NaF)光纤是最成熟的一种。实现~2.9 μm的激光通常依靠Er3+、Ho3+、Dy3+三种稀土离子。2018年,拉瓦尔大学的Aydin等[7]通过在Er3+掺杂的ZBLAN光纤上刻写光纤布拉格光栅,采用两个980 nm半导体激光器作为泵浦源,在2.82 μm处实现了41.6 W的激光输出,斜率效率为22.9%。与Er3+离子相比,Ho3+离子的5I6→5I7能级跃迁过程的发射截面更靠近3 μm,可实现更长波长的中红外激光输出。2015年,Crawford等[8]采用1150 nm的激光器泵浦Ho3+/Pr3+共掺的双包层ZBLAN光纤,获得了最高输出功率为7.2 W的中红外激光,并且实现了2.82~2.97 μm波长范围的可调谐激光输出。与上述两种稀土离子相比,Dy3+离子在3 μm波段有着更宽的发射截面,可实现3 μm以上波长的激光输出。2018年,Majewski等[9]在掺Dy3+的单包层ZBLAN光纤中实现了2807~3308 nm的可调谐激光输出,最大输出功率为0.17 W。2019年,Fortin等[10]通过同带泵浦的方式泵浦5.5 m长的Dy3+掺杂ZBLAN光纤,在3.24 μm处实现了10.1 W的激光输出。氟化铝基玻璃光纤作为一种新型的氟化物光纤,近些年在中红外光纤激光方面得到了迅速发展[11-13]。2018年,吉林大学的Jia等[11]在掺Ho3+的氟化铝基玻璃光纤中实现了2.87 μm的中红外激光输出,由于光纤损耗较高,该光纤激光器的最高输出功率仅为57 mW。2020年,Wang等[12]通过Ho3+/Pr3+共掺,提高了氟化铝基玻璃光纤中Ho3+离子~2.9 μm激光的性能,将输出功率增大到173 mW,斜率效率为10.4%。2021年,Liu等[13]在Ho3+/Pr3+共掺氟化铝基玻璃光纤中实现了功率为1.13 W的激光输出,并且维持了45 min的瓦级激光输出。氟化铟基光纤的声子能量在三种氟化物玻璃光纤中最低(~510 cm-1),更有利于实现中红外激光输出。2018年,Maes等[14]采用原子数分数为10% 的Ho3+掺杂商用氟化铟基玻璃光纤,在室温环境下实现了3.92 μm激光的输出,最高输出功率约为200 mW。
虽然ZBLAN光纤的发展已经较为成熟,但是ZBLAN光纤较差的热稳定性和化学稳定性对于进一步提高中红外光纤激光的输出功率是一个挑战。ZBYA玻璃作为另一种氟化锆基玻璃,有着与ZBLAN玻璃相近的声子能量(580 cm-1),但是其抗潮解性能更好,玻璃转变温度更高[15],这意味着ZBYA玻璃光纤有着更高的抗激光损伤阈值,在高功率中红外光纤激光产生中有着巨大的应用潜力。目前对于ZBYA玻璃光纤的报道仅仅集中在微腔激光器中,2019年,Zhao等[16]在掺Tm3+的ZBYA玻璃微球中实现了2 μm的激光输出;2020年,他们在Tm3+/Ho3+共掺的ZBYA玻璃微球中实现了1.5 μm、1.84 μm和2.08 μm三波长激光输出[17]。由于ZBYA玻璃光纤在拉制过程中容易析晶,拉制难度较大,因此ZBYA玻璃光纤激光器未见报道。
本文制备出较低损耗的Ho3+掺杂的ZBYA玻璃光纤,在2.855 μm波长处实现了137 mW的激光输出,证明ZBYA玻璃光纤在中红外光纤激光器中具有较大的应用潜力。
2 实验和结果
采用吸注法制备了单包层的ZBYA光纤预制棒,纤芯组分为50ZrF4-33BaF2-7AlF3-6.5YF3-2PbF2-1.5HoF3 (分子式前面的数字为原子数分数,单位为%,下同),包层组分为50ZrF4-33BaF2-7AlF3-10YF3。将上述高纯原料(纯度为99.99%)充分研磨混合后,在马弗炉中以850 ℃熔制1 h,然后在320 ℃的铜质模具中退火3 h来消除玻璃中残余内应力。由于OH-在~3 μm波长处有很强的吸收性,为了去除玻璃中的OH-,整个熔制与退火过程均在充满氮气的干燥手套箱内进行。纤芯组分玻璃在2000~10000 nm的透过光谱如
采用差示扫描量热法(DSC)分别对纤芯和包层玻璃进行测试,结果如
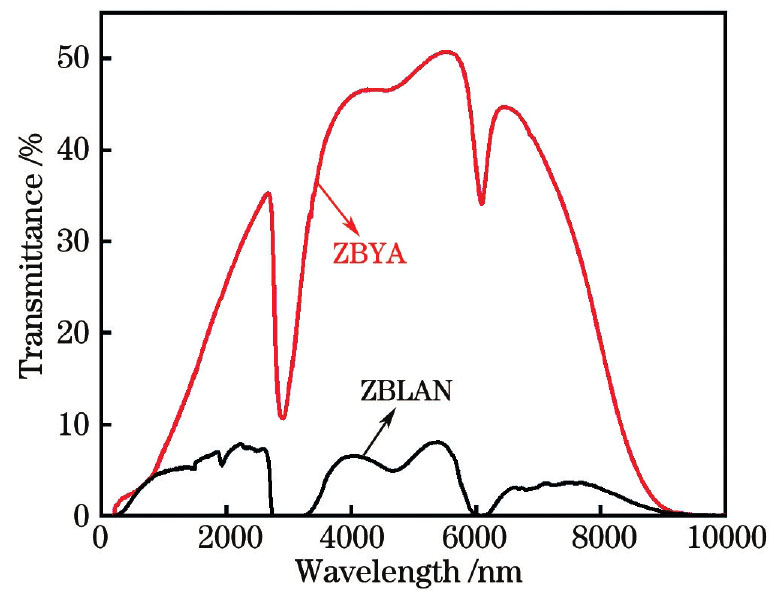
图 3. ZBYA玻璃和ZBLAN玻璃浸水24 h后的透过率光谱
Fig. 3. Transmittance spectra of ZBYA and ZBLAN glasses after immersion in water for 24 h
实验中选用1150 nm拉曼光纤激光器作为泵浦源,1150 nm的泵浦光可将Ho3+离子从基态5I8能级直接激发到2.9 μm的激光上能级5I6,通过5I6向5I7能级的辐射跃迁产生2.9 μm的激光。由于5I7能级的寿命高于5I6能级,因此Ho3+离子中~2.9 μm发光的能级跃迁过程是一个自终止过程。为了获得~2.9 μm的激光发射,必须设法减少5I7能级布居的粒子数。在高掺杂浓度的条件下,主要通过以下两个过程实现:1)激发态吸收(ESA)过程(5I7→5I4);2)能量传递上转换(ETU)过程(5I7,5I7 → 5I5,5I8)。具体过程如
2.9 μm光纤激光实验装置如
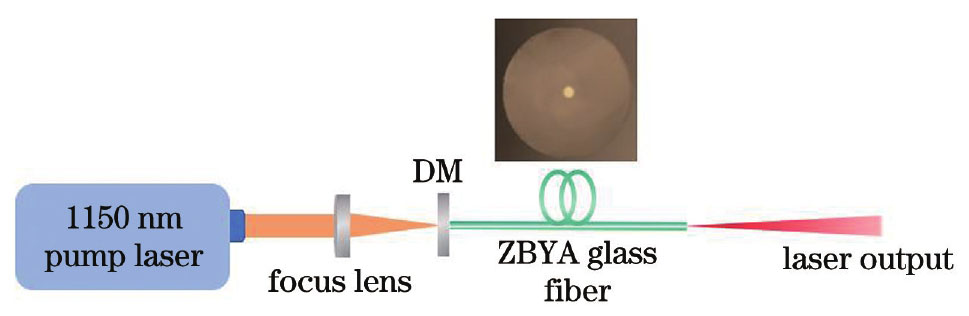
图 5. 实验装置示意图,插图为ZBYA玻璃光纤的横截面照片
Fig. 5. Schematic of the experimental set-up, the inset shows cross-section photo of ZBYA glass fiber
2.9 μm激光的输出功率随泵浦功率的变化如
本实验还测量了输出功率为137 mW时激光输出功率的稳定性。在测量~2.9 μm激光的输出功率时,通过在激光输出端放置滤光片(2400 nm长通滤光片)来过滤掉残余的泵浦光。激光输出功率的时间稳定性曲线如
ZBYA玻璃光纤作为一种新型的氟化锆基玻璃光纤,目前的问题主要存在于光纤损耗方面,较大的光纤损耗导致其输出激光的效率较低,之后通过提高原材料的纯度以及优化光纤拉制工艺,有望进一步降低ZBYA玻璃光纤的损耗。除了降低光纤损耗之外,后续的工作还应该继续探索石英光纤与ZBYA玻璃光纤的熔接技术,以降低激光系统的复杂程度,提高光纤端面能够承受的泵浦功率。在未来的工作中,探索双包层ZBYA玻璃光纤的制备方法尤为重要。单包层光纤由于泵浦光都集中在纤芯中,纤芯承受了较大的功率密度,很容易使光纤端面受到损伤;而双包层光纤通过包层泵浦的方式,能够显著增大光纤能够承受的最大泵浦功率,从而进一步提高激光输出功率。
3 结论
本研究制备出单包层结构的Ho3+掺杂的ZBYA玻璃光纤,在1150 nm激光的泵浦下,实现了~2.9 μm的激光输出,最大输出功率为137 mW,斜率效率为8.9%。此结果表明ZBYA玻璃光纤是一种潜在的用于实现中红外光纤激光输出的增益介质。
[1] Jackson S D. Towards high-power mid-infrared emission from a fibre laser[J]. Nature Photonics, 2012, 6(7): 423-431.
[2] 彭英才, 赵新为, 尚勇. 中红外量子级联激光器的研究进展[J]. 微纳电子技术, 2007, 44(9): 845-852.
Peng Y C, Zhao X W, Shang Y. Progress of the mid-infrared quantum-cascade lasers[J]. Micronanoelectronic Technology, 2007, 44(9): 845-852.
[3] 张利明, 周寿桓, 赵鸿, 等. Fe2+中红外固体激光器技术综述[J]. 激光与红外, 2012, 42(4): 360-364.
Zhang L M, Zhou S H, Zhao H, et al. Introduction of Fe2+ doped mid-infrared solid-state laser[J]. Laser and Infrared, 2012, 42(4): 360-364.
[4] Beck M, Hofstetter D, Aellen T, et al. Continuous wave operation of a mid-infrared semiconductor laser at room temperature[J]. Science, 2002, 295(5553): 301-305.
[5] Pollnan M. The route toward a diode-pumped 1-W erbium 3 μm fiber laser[J]. IEEE Journal of Quantum Electronics, 1997, 33(11): 1982-1990.
[6] Spencer D J, Beggs J A, Mirels H. Small-scale cw HF(DF) chemical laser[J]. Journal of Applied Physics, 1977, 48(3): 1206-1211.
[7] Aydin Y O, Fortin V, Vallée R, et al. Towards power scaling of 2.8 μm fiber lasers[J]. Optics Letters, 2018, 43(18): 4542-4545.
[8] Crawford S, Hudson D D, Jackson S D. High-power broadly tunable 3-μm fiber laser for the measurement of optical fiber loss[J]. IEEE Photonics Journal, 2015, 7(3): 1-9.
[9] Majewski M R, Woodward R I, Jackson S D. Dysprosium-doped ZBLAN fiber laser tunable from 2.8 μm to 3.4 μm, pumped at 1.7 μm[J]. Optics Letters, 2018, 43(5): 971-974.
[10] Fortin V, Jobin F, Larose M, et al. 10-W-level monolithic dysprosium-doped fiber laser at 3.24 μm[J]. Optics Letters, 2019, 44(3): 491-494.
[11] Jia S J, Jia Z X, Yao C F, et al. Ho3+ doped fluoroaluminate glass fibers for 2.9 μm lasing[J]. Laser Physics, 2018, 28(1): 015802.
[12] Wang S B, Zhang J Q, Xu N N, et al. 2.9 μm lasing from a Ho3+/Pr3+ co-doped AlF3-based glass fiber pumped by a 1150 nm laser[J]. Optics Letters, 2020, 45(5): 1216-1219.
[13] Liu M, Zhang J Q, Xu N N, et al. Room-temperature watt-level and tunable~3 μm lasers in Ho3+/Pr3+ co-doped AlF3-based glass fiber[J]. Optics Letters, 2021, 46(10): 2417-2420.
[14] Maes F, Fortin V, Poulain S, et al. Room-temperature fiber laser at 3.92 μm[J]. Optica, 2018, 5(7): 761-764.
[15] Zhao H Y, Wang R C, Wang X, et al. Intense mid-infrared emission at 3.9 μm in Ho3+-doped ZBYA glasses for potential use as a fiber laser[J]. Optics Letters, 2020, 45(15): 4272-4275.
[16] Zhao H Y, Li A Z, Yi Y T, et al. A Tm3+-doped ZrF4-BaF2-YF3-AlF3 glass microsphere laser in the 2.0 μm wavelength region[J]. Journal of Luminescence, 2019, 212: 207-211.
[17] Zhao H Y, Yi Y T, Wang X, et al. Triple-wavelength lasing at 1.50 μm, 1.84 μm and 2.08 μm in a Ho3+/Tm3+ co-doped fluorozirconate glass microsphere[J]. Journal of Luminescence, 2020, 219: 116889.
[18] Hanna D C. Fluorideglass fiber optics[J]. Journal of Modern Optics, 1991, 38(11): 2332-2333.
徐昌骏, 张集权, 刘墨, 王顺宾, 王鹏飞. 基于钬掺杂ZBYA玻璃光纤的中红外激光研究[J]. 中国激光, 2022, 49(1): 0101016. Changjun Xu, Jiquan Zhang, Mo Liu, Shunbin Wang, Pengfei Wang. Midinfrared Laser in Ho3+ -Doped ZBYA Glass Fiber[J]. Chinese Journal of Lasers, 2022, 49(1): 0101016.