基于飞秒激光打印的二氧化钒光谱动态调控结构
1 引 言
飞秒激光具有极高的峰值功率和极短的脉冲持续时间,基于飞秒激光的增材制造加工技术以无掩膜、加工精度高、热效应小与损伤阈值低等优点,可以实现复杂立体结构的真三维加工,已成为制造高精度二维、三维器件最具有发展前景的技术之一[1-6],被广泛应用于光学[7]、光子学[8]与生物医学等领域[9]。通过飞秒激光诱导光敏树脂材料的多光子吸收、光聚合效应[10-12],使得加工精度可突破衍射极限限制[13],实现高达9 nm的空间分辨率[14],因而适用于制造各种具有特异性功能的微纳器件[15-20],例如三维光子晶体[21-23]、金属结构[24-26]、导电器件[27-28]与远程响应机械器件[29]等。
微纳光学器件可在微纳尺度上实现对光波的操控[30],然而器件在加工成型后其光学性能随之固定下来,即仅具有静态的光学调控性能,例如基于飞秒激光微纳加工技术的树脂型微纳光学结构或器件实现光场聚焦[31]等。如果构成微纳光学器件的某一材料组分本身的电磁参数在外部激励刺激下可发生变化,那么基于该材料的微纳结构或器件的有效折射率随之发生变化,即可在外部刺激调控下实现对光波的动态调控[32]。相变材料二氧化钒(VO2)作为光活性物质,是一种强关联电子体系金属氧化物,具有Mott相变特性,在室温下VO2晶体为单斜石结构,载流子浓度为 ~ 1018 cm−3,表现出介质态特性,当温度升高至相变临界温度 ~ 68 ℃时,VO2晶体从单斜石结构转变为金红石结构,其载流子浓度提升5个数量级至 ~ 1023 cm−3,表现出类金属特性[33]。基于相变材料VO2的动态调控技术,主要是利用相变材料如VO2在外部激励刺激下发生晶态变化,继而导致材料电磁参数的变化,从而实现对基于相变材料的微纳光学结构有效折射率的调控,此种晶格变化带来的电磁参数变化具有超宽谱电磁响应特性[34],且其相变具有亚皮秒级(100 fs ~ 1 ps)响应速率[35],因而基于VO2的动态调控器件广泛应用于如开关调制[36]、电调控天线[37]、相变存储器[38]与太赫兹调控[39]等领域。由于VO2电磁参数具有色散特性,长波段相变前后折射率变化相对较大,短波段折射率变化相对较小,导致目前基于VO2材料光致相变的研究大多集中于长波红外、太赫兹波段[40],而在短波段如近红外、可见光波段,可利用超表面结构[41]或超材料结构[42]、谐振腔[43-44]等具有共振效应的微纳结构,以增强光波与VO2间相互作用,实现对短波段光波的动态调控。特别是基于VO2三维微纳光学器件可通过多维度精细控制材料与光之间相互作用,增强重叠积分,提升光调控效果,并可通过结构设计发展器件的特异性。具有短波段高效共振机制的微纳器件通常需要高精度微纳加工能力,虽然具有动态调控特性的VO2微纳光学结构已展示了其电磁调制性能,但受限于材料和三维微纳结构加工手段,目前一般采用自组装形成VO2反蛋白石结构或通过化学气相沉积技术(CVD)、原子层沉积技术(ALD)在三维骨架表面沉积一层VO2薄膜,再对沉积的薄膜进行高温晶化后处理来使得所沉积的VO2具有相变特性[32, 45]。由于此类方案存在三维微纳结构类型单一的问题,或高温后处理会造成骨架结构收缩,降低加工结构的精度,影响器件调制性能,因而都具有一定的局限性[46]。飞秒激光直写技术利用聚焦高强度光子束进行加工,具有工艺的高度可设计性、多衬底、非接触及低成本制造等优势,且具有可远超光学衍射极限的加工精度,有希望满足VO2微纳结构与器件加工技术的需求[47]。利用其特有的三维增材加工能力,飞秒激光直写技术可制备出立体微纳结构如三维层堆积结构、手性结构等,其能极大丰富亚波长光致相变组织的拓扑特性和几何构型,用以实现独特的光调制特性,如空间光、涡旋光的宽光谱偏振转换[48]、宽光谱光吸收[49]与宽光谱减反[50-51]等性能。
因此,我们提出利用光敏树脂单体材料的光聚合特性与VO2纳米晶材料的相变特性,构建功能化聚合物纳米复合材料,结合飞秒激光直写技术,实现基于VO2纳米晶的跨维度超构结构高精度一次成型加工,满足短波段光谱调控的需求。通过飞秒激光激发基于VO2纳米晶的光敏型纳米复合材料的双光子聚合效应,在光敏树脂单体分子交联成多链网络的过程中可将VO2纳米晶包裹在结构线条内部,使得所加工的结构器件内部含有VO2纳米颗粒。当外界温度达到相变临界温度时,结构中所包含的VO2纳米颗粒发生相变,使得结构的有效折射率发生变化,实现对光谱的动态调控。
2 基于VO2纳米晶光敏型纳米复合材料的制备
本文所有涉及VO2的实验使用的VO2材料均为VO2纳米颗粒,其粒径为30 ~ 50 nm。由于尺寸效应、表面效应等因素的存在,纳米颗粒处于不稳定的热力学状态,会相互吸引并团聚成为较大尺寸的颗粒。这种伴生的团聚现象导致纳米颗粒在光敏树脂单体中较难均匀分散。为了减少纳米颗粒团聚所带来的影响,通常会对纳米颗粒进行表面改性[52],通过物理、化学方法对纳米颗粒进行表面处理,改变其吸附性、化学反应活性、相容性与分散特性等,以消除聚集现象。
表面改性一般利用VO2纳米颗粒表面吸附的−OH与表面活性剂(如硅烷偶联剂)进行反应,在纳米颗粒表面形成一层有机分子,使得VO2纳米颗粒由亲水性转变为亲油性,从而可以均匀分散于光敏树脂单体中,改性整体流程图如
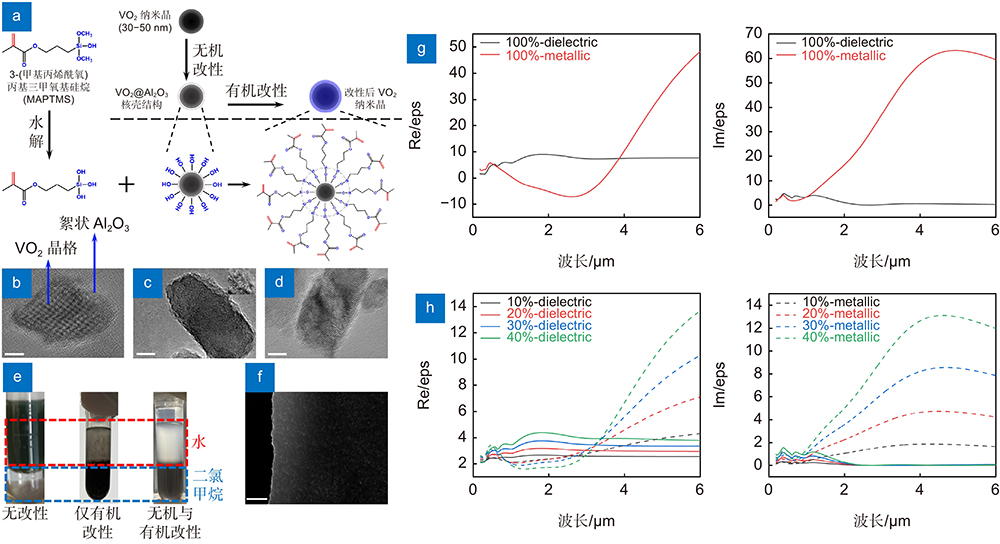
图 1. (a) VO2纳米晶颗粒无机改性与有机改性的原理、步骤示意图;(b-d) 使用三种不同无机物进行无机改性的单个VO2纳米晶颗粒的透射电子显微镜(TEM) 图片,(b)使用三氧化二铝改性,(c)使用二氧化钛改性,(d)使用二氧化硅改性,其中(b)图中VO2晶格周围存在Al2O3的无定形絮状物,标尺均为5 nm;(e) VO2纳米晶改性前后在水、二氯甲烷溶液中的分散特性对比,其中左图为未进行表面改性VO2纳米晶颗粒的分散特性,中间图为有机改性后的分散特性,右图为无机与有机改性后的分散特性;(f) 20 wt% VO2掺杂浓度的光敏型复合材料SEM图,标尺为1 μm;(g) 测试的纯VO2@Al2O3相变前后的介电常数曲线,其中左图为介电常数实部数据,右图为介电常数虚部数据,dielectric为相变前介质态,metallic为相变后类金属态;(h) 计算的10 wt%、20 wt%、30 wt%、40 wt%的VO2纳米晶光敏型复合材料升温相变前后的介电常数曲线,其中左图为介电常数实部数据,右图为介电常数虚部数据,dielectric实线为升温相变前介质态,metallic虚线为升温相变后类金属态
Fig. 1. (a) Schematic diagram of the principles and steps of inorganic modification and organic modification of VO2 nanocrystal particles; (b-d) A single transmission electron microscope (TEM) picture of VO2 nanocrystalline particles, (b) modified with aluminum oxide, (c) modified with titanium dioxide, (d) modified with silicon dioxide, and there is Al2O3 appearing as amorphous flocculent around the VO2 lattice in (b). The scales are all 5 nm; (e) The comparison of dispersion characteristics of VO2 nanocrystals before and after modification in water and dichloromethane solutions, where the left picture is without surface modification, the middle picture shows the dispersion properties after organic modification, and the right picture shows the dispersion properties after inorganic modification and organic modification; (f) SEM image of the photosensitive composite material with 20 wt% VO2. The scale is 1 μm; (g) Calculated refractive index curves of 10 wt%, 20 wt%, 30 wt%, 40 wt% VO2 nanocrystalline photosensitive composite materials before and after the phase transition with the rise of the temperature, where the left picture shows the data of the real part of the refractive index, and the picture on the right shows the data of the imaginary part of the refractive index. The solid line of dielectric represents the medium state before the phase transition with the rise of the temperature, and the metallic dotted line is the metalloid state after the phase transition with the rise of the temperature
为了探索表面电荷能对后续有机改性的影响,我们选择了三氧化二铝(Al2O3)、二氧化钛(TiO2)与二氧化硅(SiO2)三种不同金属氧化物进行相关无机改性实验。首先将三份100 mg VO2纳米颗粒粉末分别超声分散于三份等体积无水乙醇中;在1号VO2/乙醇分散液中,滴加异丙醇铝(TCI)无水乙醇分散液,经磁力搅拌老化(室温、1200 rpm、6 h)、离心(2000 rpm、20 min)、清洗(无水乙醇3次)后得到VO2@Al2O3核壳结构纳米颗粒,透射电子显微镜(TEM)图如
飞秒激光增材制造体系中光刻胶材料一般是由树脂单体、光引发剂、助引剂、光敏剂与荧光剂等组分组成的液体或者无定型固体材料。本文所采用的自配光刻胶材料包含单体季戊四醇三丙烯酸酯(PETA,Sigma-Aldrich)、光引发剂为1 wt% 4-(1-甲基乙基)-9H-噻吨-9-酮(ITX,TCI),荧光染料为0.01 wt% 香豆素(Sigma-Aldrich)。而改性后的VO2@Al2O3纳米颗粒通过磁力搅拌充分分散于上述光刻胶中,并制得基于VO2纳米晶的光敏型纳米复合材料。我们将VO2光敏型复合材料用4-甲基-2-戊酮(MIBK,安耐吉)以质量比1:9进行稀释,将稀释液以8000 rpm的转速旋涂于硅片上(旋涂时间为1 min),待溶剂挥发后进行紫外光固化,并进行相关扫描电子显微镜(SEM)表征。作为对比我们同时对改性后VO2纳米颗粒进行分散旋涂以及SEM形貌表征。如
为更好地探索光敏型纳米复合材料的有效光学参数,我们将从VO2@Al2O3纳米颗粒的折射率和复合材料的有效折射率两部分分开讨论。我们首先在Si片上制备了 ~ 120 nm厚的VO2@Al2O3膜层,利用椭偏仪测得VO2在相变前后的色散曲线。VO2在相变前(25 ℃)为介质态,利用椭偏仪测试软件拟合时可采用柯西模型进行参数拟合,得到相变前VO2介电常数曲线;而当VO2相变后(75 ℃)变为类金属态时,拟合时采用类半导体拟合模型洛伦兹-德鲁德模型进行参数拟合,得到相变后的VO2介电常数曲线。如
其中:εeff是光敏型复合材料的有效介电常数,nresin是光刻胶的折射率,N是VO2纳米颗粒数密度,
其中:
3 飞秒激光直写加工系统与光谱动态调控测试系统
本实验使用的飞秒激光直写加工系统光路图如
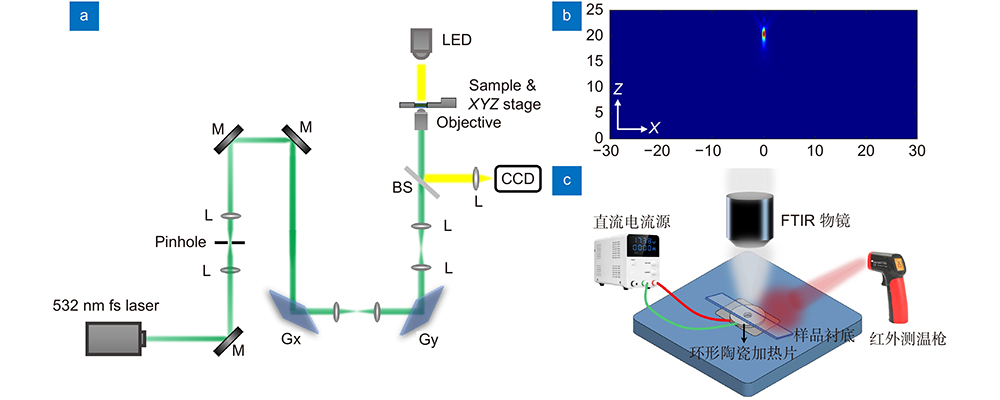
图 2. (a)飞秒激光直写加工系统光路示意图,其中M为反射镜,L为透镜,pinhole为滤波小孔,Gx与Gy为振镜系统,BS为分光棱镜,objective为物镜;(b)激光聚焦光斑的XOZ面光场分布;(c)光学器件光谱测试装置示意图,使用了环形陶瓷加热片连接直流电源对超构结构进行加热,使用红外测温枪表征超构结构的温度
Fig. 2. (a) Schematic diagram of the optical path of the femtosecond laser direct writing fabrication system. Among them, M is the mirror, L is the lens, Pinhole is the filter hole, Gx and Gy are the galvanometer system, BS is the beam splitter, and Objective is the objective lens; (b) XOZ surface optical field distribution of the laser focus spot; (c) Schematic diagram of the photonic device spectrum test device, which uses a ring-shaped ceramic heating plate connected to a DC power supply to heat the photonic device and uses an infrared temperature gun to test the temperature of photonic devices
针对VO2相变动态调控技术,我们构建了一套具有温度控制系统的微区傅里叶变换红外光谱仪测试系统,如
4 基于VO2光敏型复合材料的加工特性
光敏型复合材料中VO2浓度越高,光谱调制效果越明显,但是材料散射与吸收也随之增大,受材料色散影响飞秒激光峰值功率变化明显,导致结构加工难度变大。故我们选用VO2含量为20 wt%的光敏型纳米复合材料,该纳米复合材料能够在提供更高光谱调制效果的基础上,实现飞秒激光微纳加工。为了定量分析VO2光敏型复合材料在飞秒激光微纳加工系统中的非线性吸收效应,我们研究了飞秒激光阈值功率(Pth)与扫描速度之间的关系,如
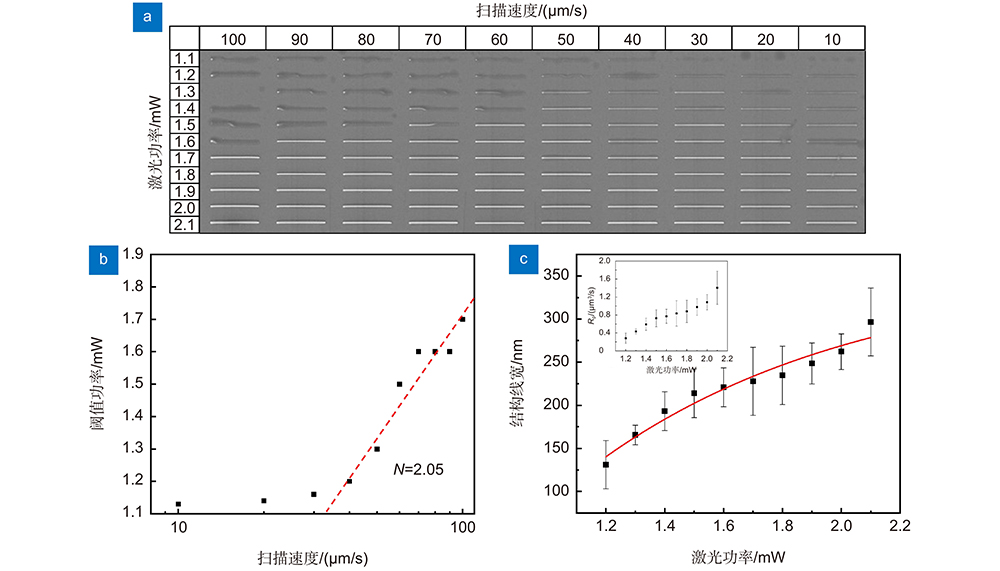
图 3. (a)不同激光功率、扫描速度下加工的单线结构SEM图片,其中VO2浓度为20 wt%;(b)基于20 wt% VO2光敏型复合材料在不同扫描速度下加工单线结构的激光阈值功率,激光阈值功率与扫描速度呈函数关系;(c)加工单线结构的结构线宽随功率变化图,其中扫描速度为20 μm/s,VO2浓度为20 wt%,插图为激光功率对聚合速率的影响
Fig. 3. (a) SEM images of the single-line structures processed at different laser powers and scanning speeds, where the VO2 concentration is 20 wt%; (b) Laser threshold power of the processed single-line structures at different scanning speeds based on 20 wt% VO2 photosensitive composite materials. The laser threshold power is a function of the scanning speed; (c) The line width of the processed single-line structures changes with the power, where the scanning speed is 20 μm/s, the VO2 concentration is 20 wt%, and the embedded figure is the laser power versus the polymerization rate
其中:N是光引发剂的非线性吸收系数,C是与光引发剂、单体性质有关的系数,激光阈值功率Pth定义为在给定扫描速度下引发光聚合所需的最小激光功率。非线性吸收系数N = 2.05说明了基于VO2纳米晶光敏型复合材料在飞秒激光诱导下发生的聚合反应过程是一个双光子吸收过程。
为进一步分析激光阈值功率与线宽之间的关系,我们固定扫描速度为20 μm/s,当激光功率从2.1 mW降低至1.2 mW时,结构线宽从 ~ 297 nm减小至 ~ 131 nm,即当激光功率减小至1.2 mW时,得到最小结构线宽为 ~ 131 nm。当光功率为1.1 mW时,光敏型复合材料不能发生光聚合。激光阈值功率与线宽的详细关系曲线如
其中:d为结构线宽,Vs为扫描速度。聚合速率随激光功率的增加而增加,其值在0.282 ~ 1.407 μm3/s,如
在探究含20 wt% VO2光敏型复合材料的非线性效应与加工工艺基础上,结合显影、定影技术,我们完善了三维微纳光学结构的飞秒激光微纳加工技术。在激光功率为2 mW,扫描速度为10 μm/s时,我们实现了横向周期为2 μm、纵向周期为1.12 μm的三维层堆积结构加工,如

图 4. (a)三维层堆积结构模型示意图;(b-d)基于20 wt% VO2光敏型复合材料加工的三维层堆积结构,其中(b)为光学显微镜图片,(c)为SEM图片,(d)为SEM斜视图;(e)螺旋二十四面体模型示意图;(f-h)基于20 wt% VO2光敏型复合材料加工的螺旋二十四面体结构,其中(f)为光学显微镜图片,(g)为SEM图片,(h)为SEM放大图片
Fig. 4. (a) Schematic diagram of the three-dimensional layer stacking structure model; (b-d) The three-dimensional layer stacking structure processed based on 20 wt% VO2 photosensitive composite material, where (b) is the optical microscope picture, (c) is the SEM picture, and (d) is the SEM oblique view; (e) Schematic diagram of the spiral icosahedral structure model; (f-h) Spiral icosahedral structure processed based on 20 wt% VO2 photosensitive composite material, where (f) is the optical microscope image, (g) is the SEM image, and (h) is the SEM enlarged image
5 基于VO2光敏型复合材料的超构结构
在定量分析了基于VO2的光敏型复合材料的飞秒激光打印技术后,我们继续探索了基于VO2光敏型复合材料超构结构的光谱调控性能。针对平面微纳结构,我们设计出了一款由自配纯光刻胶组成的周期为6 μm,占空比为0.667,厚度为1.2 μm的衍射光栅,其结构形貌如

图 5. (a)纯光刻胶型一维光栅;(b)纯光刻胶型一维光栅器件升温前后的反射光谱,其中上图为测试数据,下图为模拟数据;(c) 20 wt% VO2型一维光栅;(d) 20 wt% VO2型一维光栅器件升温前后的反射光谱,其中上图为测试数据,下图为模拟数据;(e)单个周期的20 wt% VO2光栅XOZ平面的磁场z分量分布,其中上图光源波长为2.9 μm,下图光源波长为4.2 μm,白色虚线为单个周期光栅结构的截面轮廓;(f) 20 wt% VO2型woodpile结构;(g)纯光刻胶型woodpile结构升温前后的透射光谱;(h) 20 wt% VO2型woodpile结构升温前后的透射光谱,其中黑线为25 ℃,红线为75 ℃
Fig. 5. (a) Pure photoresist-type one-dimensional grating; (b) Reflection spectra of pure photoresist-type one-dimensional grating device before and after heating, where the upper figure is the test data, and the lower figure is the simulated data; (c) 20 wt% VO2 type one-dimensional grating; (d) Reflection spectrum of 20 wt% VO2 type one-dimensional grating device before and after heating, where the upper figure is the test data, and the lower figure is the simulated data; (e) Distribution of the z component of the magnetic field in the XOZ plane of a single periodic 20 wt% VO2 grating, where the upper picture shows the light source wavelength of 2.9 μm, and the lower picture shows the light source wavelength of 4.2 μm, the white dotted line is the cross-sectional profile of a single periodic grating structure; (f) 20 wt% VO2 type woodpile structure; (g) Transmission spectra of pure photoresist woodpile structure before and after heating; (h) Transmission spectra of 20 wt% VO2 type woodpile structure before and after heating, where the black line is 25 ℃, and the red line is 75 ℃
此外,我们进一步探索了立体光学结构对光谱的调控特性,设计并制备出了周期为2 μm、纵向周期为1.12 μm的纯光刻胶型和基于20 wt% VO2聚合物纳米复合材料型三维层堆积微纳光学结构,经测量结构线宽分别为420 nm和640 nm。纯光刻胶在室温25 ℃ (黑线)和高温75 ℃ (红线)时,结构的测试光谱变化较小,如
6 结 论
本文提出了一种使用飞秒激光直写加工生成基于VO2超构结构的方案,该方案避免了传统ALD沉积VO2技术中高温后处理带来的结构失真,提高了器件加工精度。通过改性的方法使VO2纳米晶均匀分散在光刻胶中制成复合光刻胶,在具有相变的基础上保留其在飞秒激光下双光子聚合的能力,通过光刻胶的交联聚合反应,将VO2纳米晶包裹在结构线条内部,使得所加工的结构器件内部含有VO2,利用VO2材料在68 ℃左右发生相变的特性,在外界温度改变的情况下,内部的VO2发生相变,其电磁参数的变化使得结构整体的有效折射率发生变化,从而实现光谱动态调控的效果。利用复合光刻胶加工了二维平面光栅器件并对其进行了动态光学性能测试,测试结果与理论计算结果保持良好的一致性。同时使用复合光刻胶加工了复杂立体超构结构,证明了其加工复杂三维结构的能力,并测试出基于VO2的三维层堆积微纳光学结构具有约33%的动态宽带调控。本文为动态调控器件的加工与测试提供了新的思路和方法。
Overview: Optically functionalized meta-structures exhibit salient advantages in controlling and manipulating light-matter interactions at the micro-/nano- scale. These structures could enable a wide range of processes such as spectral engineering, phase manipulation, polarization control, filtering, modulation, optical angular momentum generation, and polarization conversion. The spectral properties of these artificial meta-structures emerge from optical resonances, which strongly relies on the size, shape, and arrangement of the metallic or dielectric meta-structures. By tailoring these parameters, it is possible to efficiently manipulate the spectra and achieve tunable spectra, especially in the near-infrared and visible range. Integrating or embedding materials or structures with tunable optical properties could be a better approach to overcome the limitation of being stuck in a fixed morphological nanostructure. As a Mott transition material, vanadium dioxide (VO2) can directly change its refractive index due to the insulating-metallic transition (IMT) at ~68 °C, which has been proved by applying temperature, electrical fields, or light triggers in the visible, infrared, and other spectral regions. By utilizing the meta-structure of vanadium dioxide, the regulation of the effective refractive index of micro-/nano- optical devices can be realized. However, the traditional electron beam lithography and focused ion beam machining are more suitable for the fabrication of 2D meta-structures, which greatly limits the device design and the spectra engineering. Compared with these processing technologies, femtosecond laser direct writing technology that utilizes focused high-intensity photon beam for processing and has the advantages such as freeform fabrication, non-conductive substrate and non-contact, is expected to meet the requirements of micro-/nano- fabrication of the VO2-based meta-structures.
In this paper, we demonstrate a feasible approach for fabricating meta-structures with flexibly manipulated effective refractive indices by incorporating VO2 nanoparticles in a matrix of acrylate resin. This approach involves tailoring the phase transition of VO2-based photosensitized nanocomposites. Through the implementation of this effective-refractive-index tailorable photosensitized nanocomposite, the direct printing of meta-structures can be achieved in a single attempt without the post-heat treatment. Therefore, it avoids the undesired structural shrinking and poor adhesion between the sample and the substrate that may occur in the traditional VO2 deposition methods like atomic layered deposition. By cross-linking polymerization reaction of the photosensitized nanocomposites, the VO2 nanocrystals are embedded into the potopolymerized structures. As a result, the optical response of the as-fabricated meat-structures could be dynamically tunable through the thermotropic phase transition of the VO2 nanocrystals in the nanocomposites. In addition, the broadband amplitude modulation of ~33% is observed in the three-dimensional hierarchical meta-structures. This strategy holds immense promise for future versatile utilization of the nanocrystal-based photosensitive nanocomposites to achieve numerous functionalities in meta-structures via femtosecond laser printing technique.
[1] 李佳群, 闫剑锋, 李欣, 等. 透明介质材料的超快激光微纳加工研究进展[J]. 中国激光, 2021, 48(2): 0202019.
Li J Q, Yan J F, Li X, et al. Research advancement on ultrafast laser microprocessing of transparent dielectrics[J]. Chin J Lasers, 2021, 48(2): 0202019.
[2] Capel A J, Rimington R P, Lewis M P, et al. 3D printing for chemical, pharmaceutical and biological applications[J]. Nat Rev Chem, 2018, 2(12): 422-436.
[3] Kiefer P, Hahn V, Nardi M, et al. Sensitive photoresists for rapid multiphoton 3D laser micro‐and nanoprinting[J]. Adv Opt Mater, 2020, 8(19): 2000895.
[4] Mayer F, Ryklin D, Wacker I, et al. 3D two‐photon microprinting of nanoporous architectures[J]. Adv Mater, 2020, 32(32): 2002044.
[5] Kawata S, Sun H B, Tanaka T, et al. Finer features for functional microdevices[J]. Nature, 2001, 412(6848): 697-698.
[6] Lay C L, Koh C S L, Lee Y H, et al. Two-photon-assisted polymerization and reduction: emerging formulations and applications[J]. ACS Appl Mater Interfaces, 2020, 12(9): 10061-10079.
[7] Hua J G, Liang S Y, Chen Q D, et al. Free‐form micro‐optics out of crystals: femtosecond laser 3D sculpturing[J]. Adv Funct Mater, 2022, 32(26): 2200255.
[8] Corrielli G, Crespi A, Osellame R. Femtosecond laser micromachining for integrated quantum photonics[J]. Nanophotonics, 2021, 10(15): 3789-3812.
[9] Ovsianikov A, Ostendorf A, Chichkov B N. Three-dimensional photofabrication with femtosecond lasers for applications in photonics and biomedicine[J]. Appl Surf Sci, 2007, 253(15): 6599-6602.
[10] Gansel J K, Thiel M, Rill M S, et al. Gold helix photonic metamaterial as broadband circular polarizer[J]. Science, 2009, 325(5947): 1513-1515.
[11] Jiang M L, Song S C, Li Y J, et al. 3D high precision laser printing of a flat nanofocalizer for subwavelength light spot array[J]. Opt Lett, 2021, 46(2): 356-359.
[12] Turner M D, Schröder-Turk G E, Gu M. Fabrication and characterization of three-dimensional biomimetic chiral composites[J]. Opt Express, 2011, 19(10): 10001-10008.
[13] McMillen B, Zhang B T, Chen K P, et al. Ultrafast laser fabrication of low-loss waveguides in chalcogenide glass with 0.65 dB/cm loss[J]. Opt Lett, 2012, 37(9): 1418-1420.
[14] Gan Z S, Cao Y Y, Evans R A, et al. Three-dimensional deep sub-diffraction optical beam lithography with 9 nm feature size[J]. Nat Commun, 2013, 4: 2061.
[15] Yee D W, Lifson M L, Edwards B W, et al. Additive manufacturing of 3D‐architected multifunctional metal oxides[J]. Adv Mater, 2019, 31(33): 1901345.
[16] Liu Y J, Wang H, Ho J, et al. Structural color three-dimensional printing by shrinking photonic crystals[J]. Nat Commun, 2019, 10(1): 4340.
[17] Saha S K, Wang D E, Nguyen V H, et al. Scalable submicrometer additive manufacturing[J]. Science, 2019, 366(6461): 105-109.
[18] Kelly B E, Bhattacharya I, Heidari H, et al. Volumetric additive manufacturing via tomographic reconstruction[J]. Science, 2019, 363(6431): 1075-1079.
[19] Jia Y C, Wang S X, Chen F. Femtosecond laser direct writing of flexibly configured waveguide geometries in optical crystals: fabrication and application[J]. Opto-Electron Adv, 2020, 3(10): 190042.
[20] 姜美玲, 张明偲, 李向平, 等. 超分辨光存储研究进展[J]. 光电工程, 2019, 46(3): 180649.
Jiang M L, Zhang M S, Li X P, et al. Research progress of super-resolution optical data storage[J]. Opto-Electron Eng, 2019, 46(3): 180649.
[21] Vyatskikh A, Ng R C, Edwards B, et al. Additive manufacturing of high-refractive-index, nanoarchitected titanium dioxide for 3D dielectric photonic crystals[J]. Nano Lett, 2020, 20(5): 3513-3520.
[22] Frölich A, Fischer J, Zebrowski T, et al. Titania woodpiles with complete three-dimensional photonic bandgaps in the visible[J]. Adv Mater, 2013, 25(26): 3588-3592.
[23] Hossain M M, Gu M. Broadband optical absorptions in inversed woodpile metallic photonic crystals[J]. Opt Mater Express, 2012, 2(8): 996-1002.
[24] Vyatskikh A, Delalande S, Kudo A, et al. Additive manufacturing of 3D nano-architected metals[J]. Nat Commun, 2018, 9(1): 593.
[25] Wen H J, Song S C, Xie F, et al. Great chiral fluorescence from the optical duality of silver nanostructures enabled by 3D laser printing[J]. Mater Horiz, 2020, 7(12): 3201-3208.
[26] Xu B B, Xia H, Niu L G, et al. Flexible nanowiring of metal on nonplanar substrates by femtosecond‐laser‐induced electroless plating[J]. Small, 2010, 6(16): 1762-1766.
[27] Xiong W, Liu Y, Jiang L J, et al. Laser‐directed assembly of aligned carbon nanotubes in three dimensions for multifunctional device fabrication[J]. Adv Mater, 2016, 28(10): 2002-2009.
[28] Long J, Xiong W, Wei C Y R, et al. Directional assembly of ZnO nanowires via three-dimensional laser direct writing[J]. Nano Lett, 2020, 20(7): 5159-5166.
[29] Xia H, Wang J, Tian Y, et al. Ferrofluids for fabrication of remotely controllable micro‐nanomachines by two‐photon polymerization[J]. Adv Mater, 2010, 22(29): 3204-3207.
[30] 谢常青, 朱效立, 牛洁斌, 等. 微纳金属光学结构制备技术及应用[J]. 光学学报, 2011, 31(9): 0900128.
Xie C Q, Zhu X L, Niu J B, et al. Micro-and nano-metal structures fabrication technology and applications[J]. Acta Opt Sin, 2011, 31(9).
[31] Fang W, Lei J, Zhang P D, et al. Multilevel phase supercritical lens fabricated by synergistic optical lithography[J]. Nanophotonics, 2020, 9(6): 1469-1477.
[32] Golubev V G, Kurdyukov D A, Pevtsov A B, et al. Hysteresis of the photonic band gap in VO2 photonic crystal in the semiconductor-metal phase transition[J]. Semiconductors, 2002, 36(9): 1043-1047.
[33] Peter A P, Martens K, Rampelberg G, et al. Metal‐insulator transition in ALD VO2 ultrathin films and nanoparticles: morphological control[J]. Adv Funct Mater, 2015, 25(5): 679-686.
[34] Ke Y J, Wang S C, Liu G, et al. Vanadium dioxide: the multistimuli responsive material and its applications[J]. Small, 2018, 14(39): 1802025.
[35] Hallman K A, Miller K J, Baydin A, et al. Sub‐picosecond response time of a hybrid VO2: silicon waveguide at 1550 nm[J]. Adv Opt Mater, 2021, 9(4): 2001721.
[36] Wang H, Yang Y, Wang L P. Wavelength-tunable infrared metamaterial by tailoring magnetic resonance condition with VO2 phase transition[J]. J Appl Phys, 2014, 116(12): 123503.
[37] Han C R, Parrott E P J, Humbert G, et al. Broadband modulation of terahertz waves through electrically driven hybrid bowtie antenna-VO2 devices[J]. Sci Rep, 2017, 7(1): 12725.
[38] Liu L, Kang L, Mayer T S, et al. Hybrid metamaterials for electrically triggered multifunctional control[J]. Nat Commun, 2016, 7: 13236.
[39] Wang R, Yang W Y, Gao S, et al. Direct-writing of vanadium dioxide/polydimethylsiloxane three-dimensional photonic crystals with thermally tunable terahertz properties[J]. J Mater Chem C, 2019, 7(27): 8185-8191.
[40] Zhang Y B, Wu P H, Zhou Z G, et al. Study on temperature adjustable terahertz metamaterial absorber based on vanadium dioxide[J]. IEEE Access, 2020, 8: 85154-85161.
[41] Hashemi M R M, Yang S H, Wang T Y, et al. Electronically-controlled beam-steering through vanadium dioxide metasurfaces[J]. Sci Rep, 2016, 6: 35439.
[42] Driscoll T, Palit S, Qazilbash M M, et al. Dynamic tuning of an infrared hybrid-metamaterial resonance using vanadium dioxide[J]. Appl Phys Lett, 2008, 93(2): 024101.
[43] Taylor S, Yang Y, Wang L P. Vanadium dioxide based Fabry-Perot emitter for dynamic radiative cooling applications[J]. J Quant Spectrosc Radiat Transfer, 2017, 197: 76-83.
[44] Kim M K, Lee D S, Yang Y H, et al. Switchable diurnal radiative cooling by doped VO2[J]. Opto-Electron Adv, 2021, 4(5): 200006.
[45] Long L S, Taylor S, Wang L P. Enhanced infrared emission by thermally switching the excitation of magnetic polariton with scalable microstructured VO2 metasurfaces[J]. ACS Photonics, 2020, 7(8): 2219-2227.
[46] Premkumar P A, Toeller M, Radu I P, et al. Process study and characterization of VO2 thin films synthesized by ALD using TEMAV and O3 precursors[J]. ECS J Solid State Sci Technol, 2012, 1(4): P169-P174.
[47] Ji R, Hua Y N, Chen K, et al. A switchable metalens based on active tri-layer metasurface[J]. Plasmonics, 2019, 14(1): 165-171.
[48] Yan D X, Meng M, Li J S, et al. Vanadium dioxide-assisted broadband absorption and linear-to-circular polarization conversion based on a single metasurface design for the terahertz wave[J]. Opt Express, 2020, 28(20): 29843-29854.
[49] Ding F, Zhong S M, Bozhevolnyi S I. Vanadium dioxide integrated metasurfaces with switchable functionalities at terahertz frequencies[J]. Adv Opt Mater, 2018, 6(9): 1701204.
[50] Liu C, Wang S C, Zhou Y, et al. Index-tunable anti-reflection coatings: Maximizing solar modulation ability for vanadium dioxide-based smart thermochromic glazing[J]. J Alloys Compd, 2018, 731: 1197-1207.
[51] 杨港, 郭迎辉, 蒲明博, 等. 基于相关性选择的微型计算光谱探测技术[J]. 光电工程, 2022, 49(10): 220130.
Yang G, Guo Y H, Pu M B, et al. Miniature computational spectral detection technology based on correlation value selection[J]. Opto-Electron Eng, 2022, 49(10).
[52] 王宝利, 朱振峰. 无机纳米粉体的团聚与表面改性[J]. 陶瓷学报, 2006, 27(1): 135-138.
Wang B L, Zhu Z F. Accumulation and surface modification of inorganic nano-particles[J]. J Ceram, 2006, 27(1).
[53] Werdehausen D, Staude I, Burger S, et al. Design rules for customizable optical materials based on nanocomposites[J]. Opt Mater Express, 2018, 8(11): 3456-3469.
[54] Song S C, Li Y J, Yao Z F, et al. 3D laser nanoprinting of optically functionalized structures with effective-refractive-index tailorable TiO2 nanoparticle-doped photoresin[J]. Nanomaterials, 2021, 12(1): 55.
[55] 夏小兰, 曾宪智, 宋世超, 等. 基于柱矢量光调控的纵向超分辨率准球形多焦点阵列[J]. 光电工程, 2022, 49(11): 220109.
Xia X L, Zeng X Z, Song S C, et al. Longitudinal super-resolution spherical multi-focus array based on column vector light modulation[J]. Opto-Electron Eng, 2022, 49(11).
[56] 曹耀宇, 谢飞, 张鹏达, 等. 双光束超分辨激光直写纳米加工技术[J]. 光电工程, 2017, 44(12): 1133-1145.
Cao Y Y, Xie F, Zhang P D, et al. Dual‐beam super‐resolution direct laser writing nanofabrication technology[J]. Opto-Electron Eng, 2017, 44(12).
[57] 张心正, 夏峰, 许京军. 激光超衍射加工机理与研究进展[J]. 物理学报, 2017, 66(14): 144207.
Zhang X Z, Xia F, Xu J J. The mechanisms and research progress of laser fabrication technologies beyond diffraction limit[J]. Acta Phys Sin, 2017, 66(14).
[58] Yu H Y, Ding H B, Zhang Q, et al. Three-dimensional direct laser writing of PEGda hydrogel microstructures with low threshold power using a green laser beam[J]. Light Adv Manuf, 2021, 2(1): 31-38.
[59] Gao W, Chao H, Zheng Y C, et al. Ionic carbazole-based water-soluble two-photon photoinitiator and the fabrication of biocompatible 3D hydrogel scaffold[J]. ACS Appl Mater Interfaces, 2021, 13(24): 27796-27805.
Article Outline
朱家琦, 吴世宇, 宋世超, 曹耀宇. 基于飞秒激光打印的二氧化钒光谱动态调控结构[J]. 光电工程, 2023, 50(7): 230095. Jiaqi Zhu, Shiyu Wu, Shichao Song, Yaoyu Cao. Femtosecond laser printing of vanadium dioxide based optical meta-structures with tunable spectra engineering[J]. Opto-Electronic Engineering, 2023, 50(7): 230095.