超声对激光熔覆WC颗粒强化涂层耐磨防腐性能的影响(特邀)
0 引 言
海洋及矿山领域机械装备的叶片、截齿、轴等关键部件长期处于机械磨损与电化学腐蚀的恶劣服役环境[1],摩擦-腐蚀的交互作用会加速部件表面的破坏损伤,导致其服役寿命大幅下降[2]。为了延长装备的服役寿命、突破基材原有性能的限制,常采用喷涂[3]、电化学沉积[4]、原位合成[5]、高能束熔融沉积[6]等方法在部件表面制备性能优异的强化涂层。其中,激光熔覆技术因其冶金结合强度好、稀释率低、微观结构精细、力学性能优异等优势,已被广泛应用于航空、能源、海洋、矿山、冶金等多领域机械部件表面强化涂层的制备[6]。
针对部件表面高耐磨损需求,研究学者采用将碳化物[7]、氮化物[8]、氧化物[9]等硬质陶瓷颗粒与基体金属粉末进行机械混合,然后用激光熔覆技术将混合粉末熔覆在基体表面以制备陶瓷颗粒增强金属基复合涂层。其中,碳化钨(WC)由于其优异的化学稳定性、润湿性与附着力,已成为激光熔覆强化涂层最常用的陶瓷颗粒之一[10]。Benarji等[11]采用激光熔覆技术制备了SS316-WC复合强化涂层,发现WC体积分数的增加可以提高涂层的显微硬度与耐磨性能。Li等[12]采用激光熔覆技术制备了不同形状WC的Inconel 625-WC复合涂层,发现不同形状的WC在激光辐照下分解趋势不同,球形的WC更不易分解产生W6C2.54、M23C6等硬脆相,相较于非球形WC具有更好的耐磨性能。Wang等[13]采用不同粒径的WC颗粒制备了激光熔覆强化涂层,发现WC颗粒表现出溶解-扩散控制热损伤、坍缩-溶解-扩散控制热损伤和溶解-沉淀控制热损伤的不同特性,进而影响了WC颗粒表面合金反应层与枝晶的生长过程、碳化物的析出以及显微组织的均匀性。
研究表明,WC的引入虽然能提升涂层的耐磨性能,但在高能激光束作用下,WC颗粒的溶解会改变涂层的物相组成与显微结构[14],进而影响涂层的耐蚀性能。Zhang等[15]制备了不同WC含量的Inconel 625-WC复合涂层,发现随着WC含量的增加,析出碳化物会加剧固溶强化元素的局部偏析,涂层的耐磨性能逐渐提升,但耐腐蚀性能逐渐降低。Li[16]研究了WC颗粒的含量、形态和分布对激光熔覆涂层组织、力学性能、耐蚀性能和耐磨性能的影响,发现耐蚀性能随着WC含量的增加先提高后降低。Jayaraj等[17]研究了WC与不同粘结相制备的强化涂层的腐蚀行为,结果表明,WC与不同粘结相会产生不同显微组织,极大地影响了涂层钝化膜的生成稳定性,进而影响涂层的耐蚀性能。针对激光熔覆强化涂层的组织调控难题,国内外学者在激光熔覆过程中施以电[18]、磁[19]、热[20]、声[21]等外加能场辅助控形控性。其中,超声能场在金属熔池中产生的声空化效应、声流效应以及机械效应可以抑制元素偏析、细化晶粒[22]、促进增强相均匀分布[23],被认为是能够实现激光熔覆显微组织调控的有效方法。然而,当前超声辅助激光熔覆WC颗粒增强涂层的相关研究报道主要集中在耐磨性能的影响,Wang[24]和Lv[25]采用超声振动辅助激光熔覆制备了Inconel 718-WC复合涂层,发现超声振动可降低颗粒的聚集,细化晶粒,提升平均显微硬度和耐磨性能;Nie等[26]研究了超声振幅对激光熔覆WC陶瓷颗粒在熔池凝固过程中流动特性的影响,通过改变超声振幅促使WC陶瓷颗粒在熔池中逐渐上浮,提升了涂层硬度和耐高温磨损性能。然而,当前超声对WC颗粒增强复合涂层的耐蚀性能影响研究较为欠缺,对于耐蚀性能的影响机制尚不明晰,仍需进一步深入研究。
针对激光熔覆WC颗粒强化涂层高耐磨性能与高耐腐蚀性能难以兼容的问题,文中将超声振动引入激光熔覆过程,制备了有无超声作用下的WC颗粒强化涂层。分析了超声振动对复合涂层微观组织形貌、元素分布、WC表面合金层厚度的影响机制,并进一步开展了有无超声试样硬度、摩擦磨损与耐蚀性能测试。相关研究为制备具有高耐磨防腐性能的WC颗粒强化涂层提供了参考。
1 试验材料与方法
1.1 试验材料与设备
试验基体材料为316L不锈钢板材,其化学成分如表1所示。在开展激光熔覆试验前,打磨板材以去除表面氧化膜,并使用酒精清洗表面污渍后干燥放置备用。试验所用316L粉末与WC陶瓷颗粒的形貌如图1(a)和(b)所示,其中316L粉末粒径为70~100 μm,WC陶瓷颗粒粒径为50~100 μm,试验前使用行星球磨机(QM-3SP4)将316L粉末与WC陶瓷颗粒按质量比1∶4进行充分混合,将混合粉末置于真空干燥箱进行干燥处理,保温温度120 ℃,保温时间60 min。
表 1. 316L不锈钢的化学成分(wt.%)
Table 1. Chemical composition of 316L stainless steel (wt.%)
|
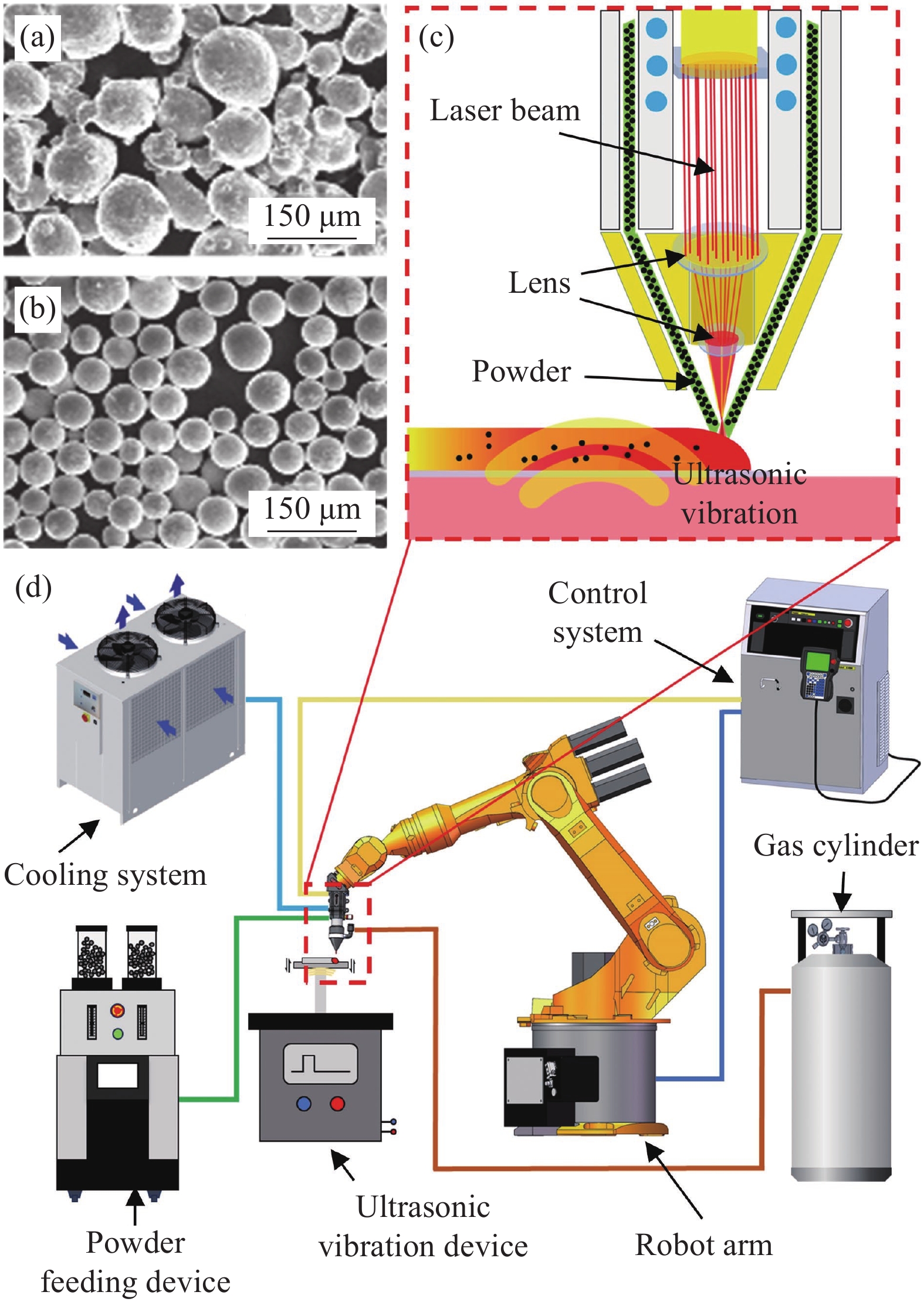
图 1. (a) 316L粉末;(b) WC粉末;(c) 超声辅助激光熔覆示意图;(d) 超声辅助激光熔覆试验平台
Fig. 1. (a) 316L powder; (b) WC powder; (c) Schematic diagram of ultrasonic assisted laser cladding; (d) Ultrasonic assisted laser cladding test platform
搭建的超声辅助激光熔覆试验平台如图1(d)所示。激光器为半导体激光器(Laserline,LDF400-2000),最大输出功率为2 000 W,波长范围900~1064 nm,焦距为25 mm,焦距处的激光光斑为直径4 mm的圆形光斑,光斑范围内的能量为平顶分布;运动与控制系统为6轴机器人(ABB,IRB2400-16/1.5),最大速度为150 (°)/s、轨迹精度与重复定位精度为0.06 mm;超声振动装置由超声波发生器、换能器、法兰盘、变幅杆以及控制面板等部件组成,其技术指标如表2所示,试验前使用螺栓将基体材料固定至超声变幅杆上端面以将超声能量稳定传输至基板;粉末输送与气氛保护气体均为氩气。
表 2. 超声振动装置关键技术指标
Table 2. Key technical parameters of ultrasonic vibration device
|
1.2 试验与分析测试方法
试验所用激光功率为1200 W,扫描速度为8 mm/s,搭接率为45%,送粉量为20 g/min,保护气流速为8 L/min,超声频率为20 kHz,超声功率为2500 W。
激光熔覆试验后,采用线切割机对熔覆层进行取样,尺寸为7 mm×3 mm×3 mm,随后进行镶样、打磨、抛光、腐蚀。采用光学显微镜(ZEISS,Axioscope)、扫描电子显微镜(ZEISS,EVO18)以及能谱仪(EDS,BRUKERXFlash 6130)对熔覆层的显微组织进行观察分析;采用自动显微硬度计(Shimadzu,HMV-2TADWXY)对熔覆层横截面沿深度方向进行硬度测试,加载载荷为100 N,保荷时间为10 s,采用球盘式摩擦磨损仪(MMQ-02)进行摩擦磨损测试,对磨球为Si3N4陶瓷球,直径为4.75 mm,测试载荷为20 N,磨损轨迹半径4.0 mm,电机转速为200 r/min,磨损时间为40 min,磨损测试前后使用电子秤(Lichen, FA123C)对试样称重以获得磨损质量,采用共聚焦显微镜(KEYENCE, VK-X1000)获取试样的三维磨损轮廓以及磨屑的微观形貌,采用电化学工作站(CHI760e)进行耐腐蚀性能测试,电解质溶液为3.5%质量分数的NaCl溶液,工作电极为1 cm2的铂片电极,参比电极为饱和甘汞电极,试样有效面积为1 cm2。
2 试验结果与讨论
2.1 超声对激光熔覆复合涂层微观组织的影响
图2为有无超声振动作用下激光熔覆复合涂层中WC颗粒周围的金相组织,无超声作用下,WC周围表现出大量的柱状枝晶以及一部分的元素偏析带,如图2(a)和(c)所示,而在超声振动作用下,试样金相未见明显的偏析带,WC周围晶粒得到明显细化,如图2(b)和(d)所示。由于激光熔覆具有快速温升与冷却的特点,金属熔体凝固时,晶体易在WC外壁上进行非均质形核,并外延生长形成柱状枝晶,同时由于部分WC溶解导致结晶时元素分布不均匀,熔覆层易形成偏析带[27],有无超声作用下的WC颗粒强化涂层晶粒分布图如图2(e)如示,可见相较于无超声作用下的晶粒尺寸,有超声作用下WC颗粒周围的晶粒尺寸明显细化,进一步对其晶粒尺寸进行统计,在超声作用下,熔覆层平均晶粒尺寸从101.0 μm降至59.6 μm。
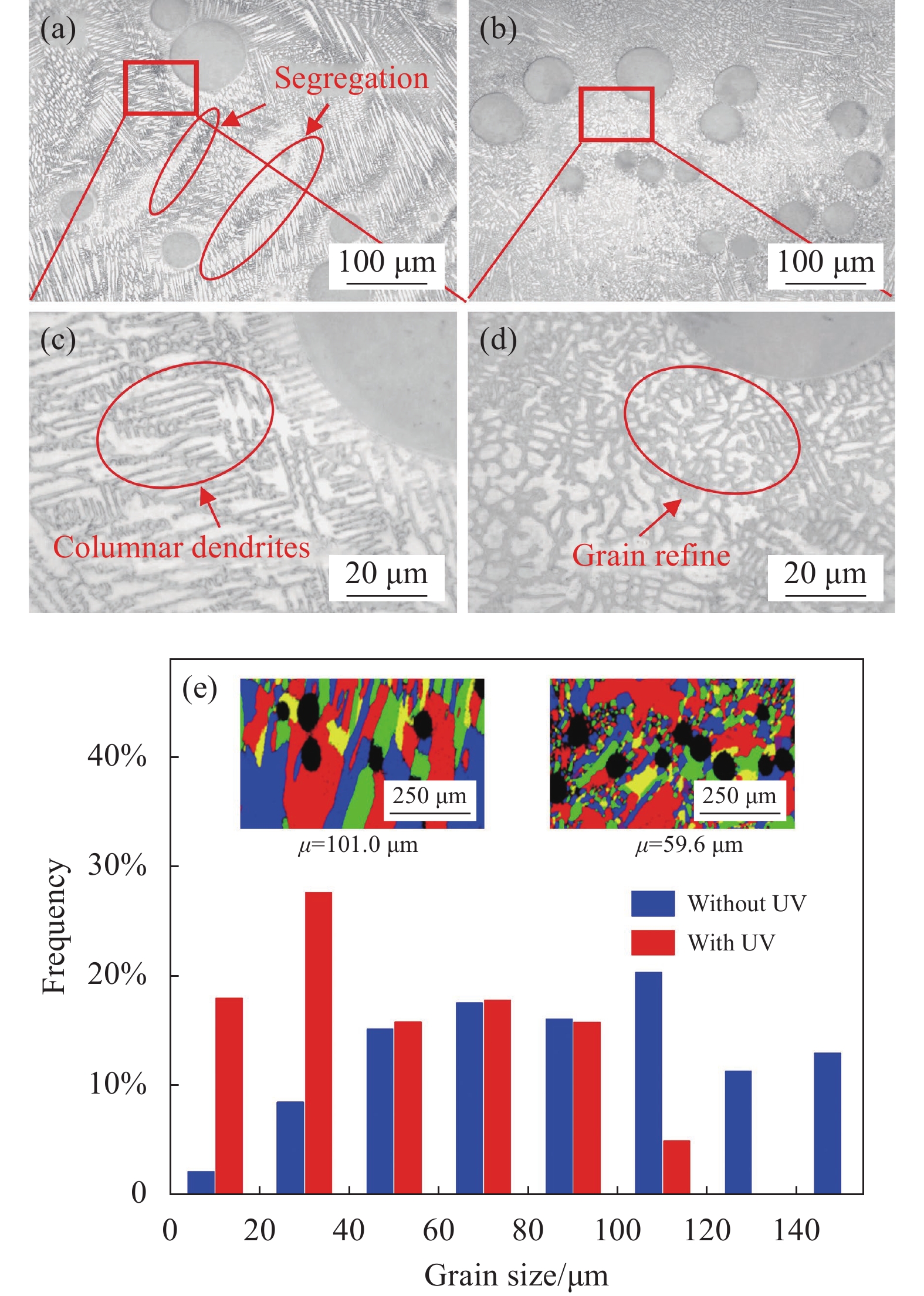
图 2. 熔覆层WC颗粒周围组织形貌。(a)、(c) 无超声;(b)、(d) 有超声;(e)晶粒尺寸分布对比
Fig. 2. Microstructure morphology around WC particle of cladding layer. (a), (c) Without ultrasound; (b), (d) With ultrasound; (e) Comparison of grain size distribution
图3为有无超声作用下WC颗粒周围微观组织的扫描电子显微镜形貌及其元素分布的点扫与面扫结果。无超声作用下,WC表面存在较厚的合金层,此外,在WC颗粒周围分布着大量细小的鱼骨状组织,如图3(a)所示;而在超声作用下,WC表面未见明显的合金层,周围散布着少许网状的析出相,如图3(b)所示。EDS结果表明,在无超声试样中WC表面合金层(P1、P2)中除了含有大量的W元素以外,还富集了Fe、Cr元素,P3为鱼骨状共晶组织,其Fe:(Ni,W)的原子比接近1∶1,P4的主要成分为Fe元素;在有超声的试样中,合金反应层明显变薄,点扫描能谱分析(P5)显示Fe、Cr元素富集现象减弱,P6、P7的主要元素均为Fe元素,其中P7的W元素含量稍高于P6。对WC径向进行元素线扫描,从无超声的试样(Line1)强度分布可以看出,在合金反应层中,Fe、W、Cr等元素均沿扩散方向梯度下降,同时在基体中由于存在大量析出相呈现波动变化,而有超声的试样中(Line2),WC与基体间的元素变化梯度更大,这表明合金层很薄,而在基体中各元素的强度变化不大,这是因为超声减少了析出相,均匀了组织。
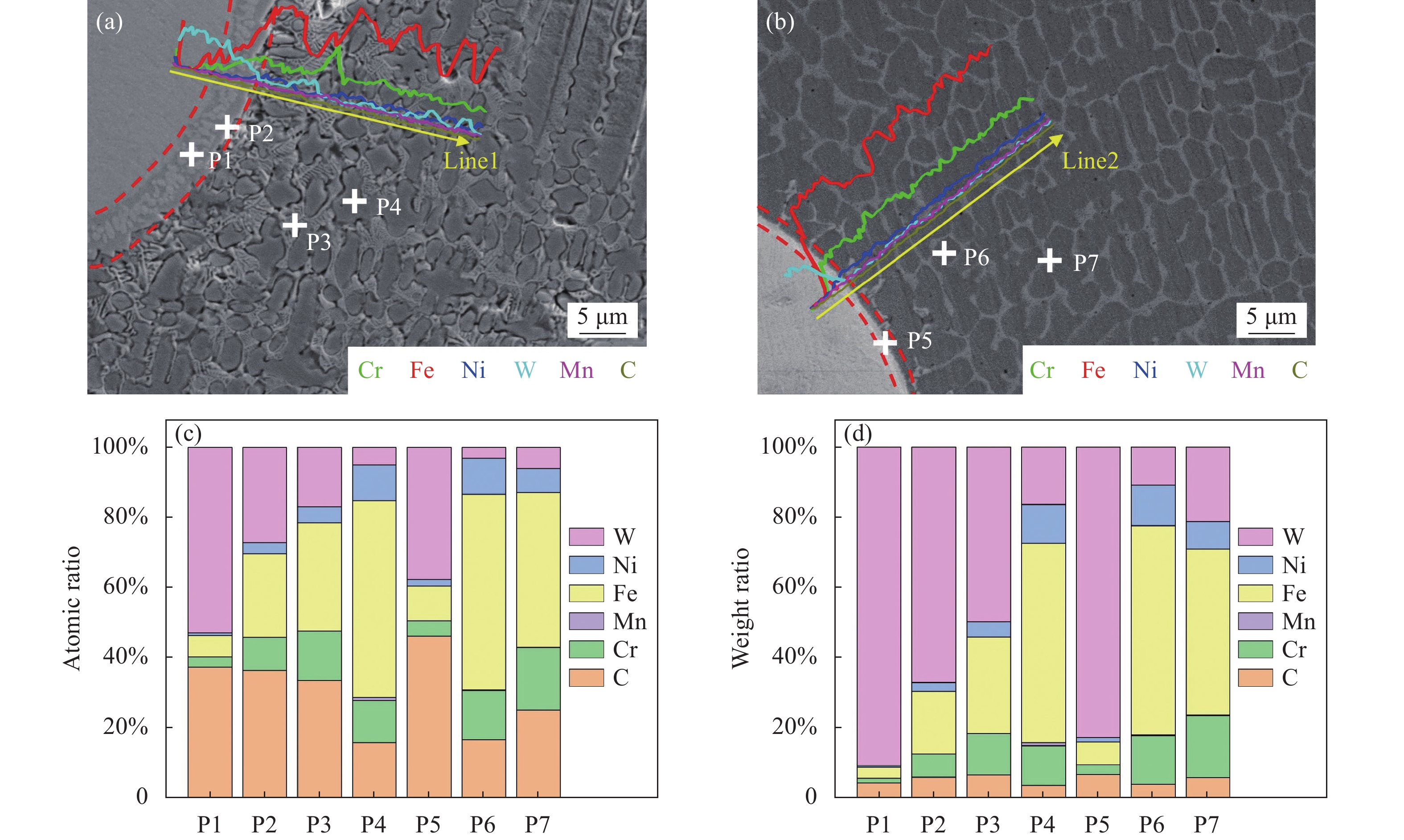
图 3. 有无超声作用下WC周围微观组织及元素分布。(a) 无超声;(b) 有超声;(c) 原子比对比;(d) 质量比对比
Fig. 3. Microstructure and element distribution around WC with and without ultrasound action. (a) Without ultrasound; (b) With ultrasound; (c) Comparison of the atomic ratio; (d) Comparison of of the mass ratio
由于激光的高能量,WC在熔池中吸收了大量的热量,分解为游离的W、C原子,当熔池中W含量(质量分数)为35%~52%时,即在WC颗粒的周围,熔池中将生成W2C和Fe3W3C[28]。随着熔池温度不断降低,在平衡状态下,熔池中容易生成M6C。故WC颗粒在凝固过程中易形成表面合金反应层,P1由于接近WC,含有更高含量的W元素,推测其主要成分为W2C;P2中Fe与W元素含量比接近1∶1,推测其为Fe3W3C,同时WC表面还有Cr、Ni等元素参与反应,整个合金反应层主要成分应为W2C、Fe3W3C和M6C(M为Fe、W、Cr和Ni)的混合组织,而周围的组织主要以316L的基体相γ-Fe(P4)为主,其中夹杂的鱼骨状碳化物(P3)根据其形状与Fe:(Ni,W)的原子比推测其为Fe3W3C富铁型碳化物与γ(Fe,Ni)的共晶组织[19];而在有超声的试样中,超声的空化作用导致合金层(P5)的溶解变薄,接近P1、P2的中间组成成分;对比P4、P6两点,发现其元素成分相近,这是因为超声不会影响熔覆层物相的组织成分,仅会减少析出相的含量[24-25],P7是有超声下熔覆层的析出相,并没有形成微小的鱼骨状碳化物,这是因为超声的声流作用促进熔池流动,降低了WC颗粒周围的W含量,减少了Fe3W3C的生成,故超声振动后WC颗粒周围组织依然以γ-Fe为主。
无超声熔覆层中析出的鱼骨状碳化物(P3)其大多属于硬脆相,极易在WC颗粒周围形成梯度的硬度分布,易产生应力集中,因而韧性较差,在摩擦时更易破裂影响耐磨性能。超声对析出相的改善有望提升熔覆层耐磨性能。
为了进一步验证超声对元素分布的影响,采用面扫描的方式对WC周围进行元素分析,如图4(a)所示,无超声作用时WC周围的W元素分布在Fe、Ni元素之中;而在施加超声的试样中,如图4(b)所示,Fe、Ni、W等元素分布相对均匀,没有明显的夹杂现象。

图 4. WC颗粒周围元素面分布图。(a) 无超声;(b) 有超声
Fig. 4. Surface distribution of elements around WC particle. (a) Without ultrasound; (b) With ultrasound
在激光辐照下,WC发生分解,释放出游离的W和C原子。在随后的快速冷却过程中,由于时间有限,W和C原子无法迅速扩散到更大的范围,因此在WC颗粒周围形成了富W的碳化物,由于W的碳化物具有极高的熔点,在熔池中更易凝固析出,会在面扫能谱图中表现出Fe、Ni和W元素存在交错分布的现象。而超声的引入,声流效应加速了熔池流速,主要的合金元素诸如Fe、Ni、W也得以均匀化,其中W元素也因此扩散到更远的距离,降低了WC颗粒周围的W元素,也进一步影响了析出相的生成。
超声的作用机制如图5所示,在激光熔覆过程中,超声的空化效应会产生大量的空化泡,空化泡在超声声压与熔体驱使的共同作用下坍缩溃灭。空化气泡的膨胀会吸收周围液体的大量热量,导致熔池局部区域的过冷;而空化泡溃灭的瞬间会产生微射流,破碎在WC颗粒表面生长的初生枝晶形成细小均匀的组织结构,并将其分散到熔池的各个部位,形成新的形核质点,这两个因素都有助于提高熔池成核速率[29],进而导致微观结构的细化。同时,空化泡溃灭产生局部高温高压促使WC表面合金层的溶解,伴随着超声的声流作用,这些溶解的合金元素均匀地分布在整个熔覆层中,可以避免熔池凝固时元素的不均匀分布,抑制偏析。晶粒细化,晶界增加,对位错运动的阻碍增加,材料形变的阻力变大,有利于熔覆层硬度提升。而元素的不均匀会在晶界和晶内形成微原电池反应,加速涂层表面腐蚀,极大地降低熔覆层的耐蚀性能[30],超声可以实现组织和元素的均匀化,有利于减少复合涂层内部的电势差距,减小腐蚀电流,提高涂层的耐蚀性能。
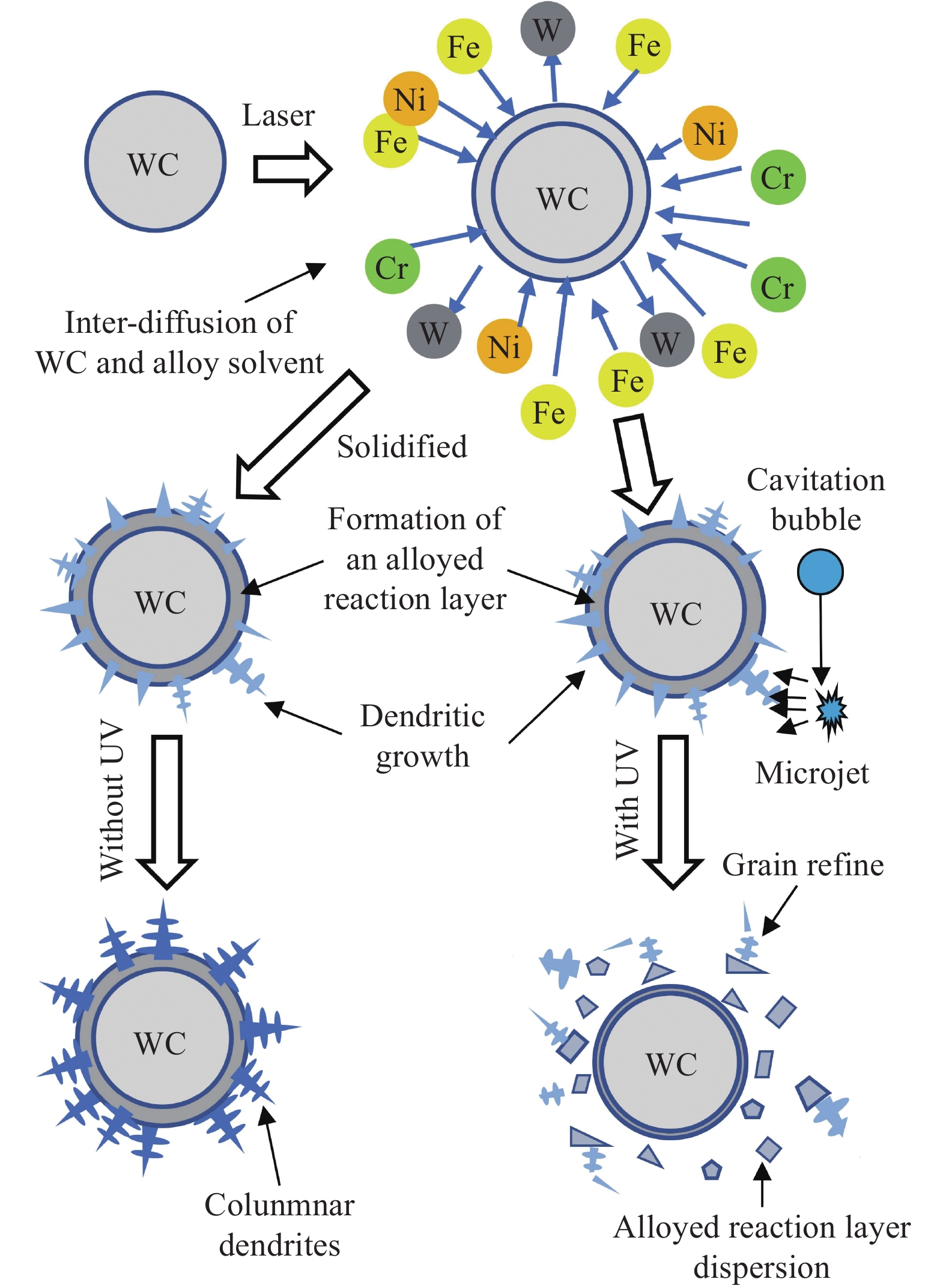
图 5. 超声对WC合金层与枝晶的作用机制
Fig. 5. Mechanism of ultrasonic vibration on WC alloy reaction layer and dendrites
2.2 超声对激光熔覆复合涂层硬度分布的影响
图6(a)为有无超声试样横截面沿深度方向的显微硬度分布,测试方向由熔覆层的顶端延伸至基体。相较于基体,无论有无超声作用下的熔覆层显微硬度均有明显提升,熔覆层与基板间热影响区的显微硬度迅速下降,直到达到基体的硬度(200 HV0.1)。其中,在无超声试样中,熔覆层硬度分布在310 HV0.1,显微硬度的波动范围为75 HV0.1,而添加超声的试样熔覆层硬度分布在425 HV0.1之间,显微硬度的波动范围为30 HV0.1,相较于无超声试样显微硬度提升了37%。图6(b)为有无超声作用下WC周围的硬度分布,硬度测试以WC颗粒为中心,间隔90°,步距40 μm进行打点并计算其平均硬度,无超声作用下,WC四周硬度由480 HV0.1下降至320 HV0.1,呈逐步降低的趋势,超声作用下,WC周围硬度分别为426 HV0.1、417 HV0.1和413 HV0.1,分布较为均匀。
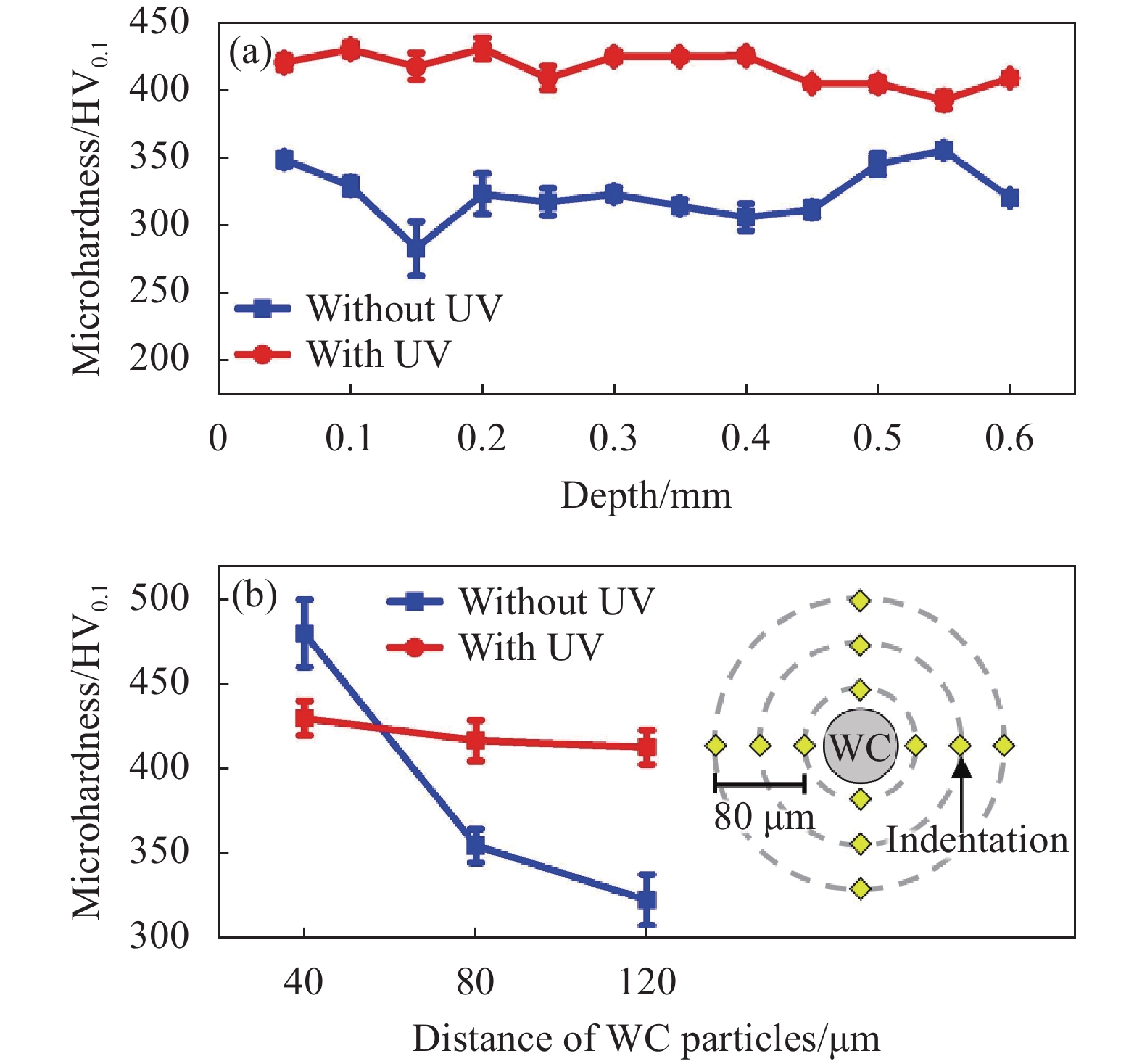
图 6. 有无超声试样横截面的显微硬度分布。(a) 沿深度方向的硬度分布;(b) WC周围硬度分布
Fig. 6. Microhardness distribution of cross sections of samples with and without ultrasound. (a) Hardness distribution along depth; (b) Hardness distribution around WC particle
综合考虑图2~图5,无超声的试样存在大量碳化物析出相,这些碳化物硬度较高,其分布不均匀导致硬度测试出现较大的波动,而超声作用导致WC表面合金层溶解扩散,将W的析出相均匀在整个熔覆层中,不仅提升了硬度性能,还减少了硬度波动的范围。无超声的试样熔覆层中越靠近WC的区域,W、C等对硬度提升较强的元素含量越高,而超声的引入对元素的均匀化减少了元素的梯度变化,表现出更为平缓的硬度梯度分布。综上所述,超声作用在激光熔覆过程中,通过促进WC表面合金层的溶解扩散、均匀元素分布,显著提高了熔覆层的平均硬度并使其分布更加均匀。
2.3 超声辅助激光熔覆复合涂层耐磨损性能分析
图7(a)为WC颗粒强化涂层摩擦系数随时间变化的曲线,在摩擦磨损的初始阶段,摩擦副表面相对光滑,摩擦系数较低。随着摩擦时间的增长,摩擦副接触面积不断扩大,摩擦系数迅速上升。当摩擦副达到相对稳定状态后,无超声的试样摩擦系数围绕0.6上下波动,而有超声的试样摩擦系数围绕着0.5上下波动。图7(b)为经过摩擦磨损试验后涂层的质量损失情况,无超声的熔覆层磨损质量为8.8 mg,有超声的熔覆层磨损质量为6.5 mg,磨损率计算公式如公式(1)所示:
式中:Mr为磨损率;M为质量损失;d为磨环直径;N为摩擦磨损旋转转数。
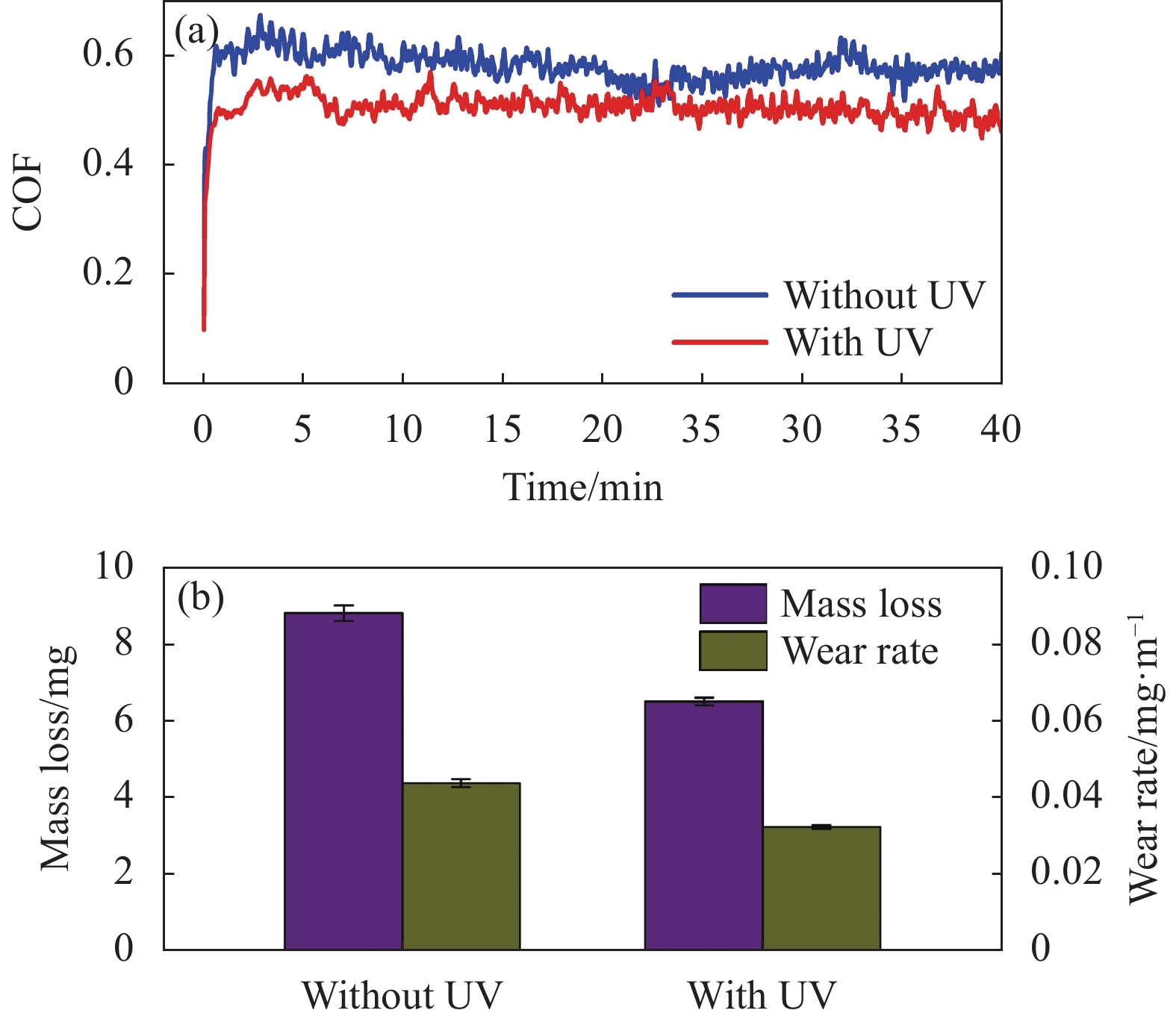
图 7. (a) 有无超声试样摩擦系数曲线;(b) 有无超声下的磨损量与磨损率
Fig. 7. (a) Friction coefficient curve of samples with and without ultrasound; (b) Wear amount and wear rate of samples with and without ultrasound
超声的加入使得磨损率从0.0438 mg/m 降低到0.0323 mg/m,降低了26.2%。同时,无超声作用下涂层摩擦系数呈现先减小再升高的趋势,而超声作用下涂层摩擦系数相对稳定,这是由于无超声作用下涂层组织与硬度分布不均,随着磨痕的深入,由于涂层不同区域硬度存在差异,其耐磨损性能存在差异;而在超声作用下,涂层组织硬度的分布均匀,涂层具有较为一致的耐磨损性能。
图8为WC颗粒强化涂层磨损后磨痕三维形貌,可以看出均是由磨粒磨损为主,对比图8(a)~(d)可以看出,无超声的试样磨痕犁沟较深,最高深度约为53 μm,有超声的试样磨痕犁沟较浅,仅26 μm左右,但是犁沟底部依然有多处不均匀的起伏,结合磨痕形貌,如图8(e)~(f)可见,未施加超声的试样有着更深的沟槽,表示其有着更高的磨损率,这与图8的结果保持一致。
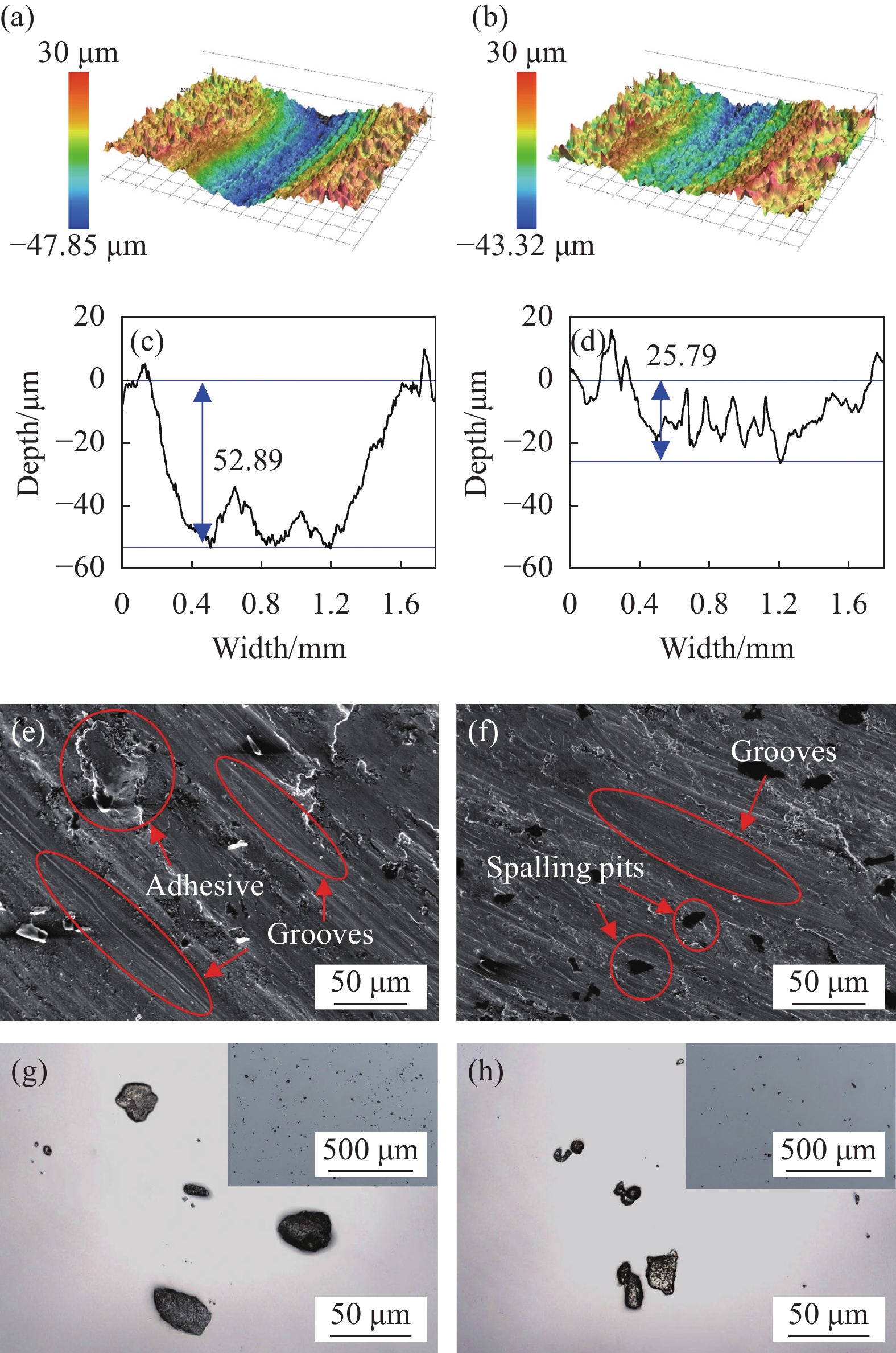
图 8. 有无超声试样磨痕轮廓和磨屑形貌。(a)、(c) 、(e)、(g) 无超声;(b)、(d)、(f)、(h) 有超声
Fig. 8. Cross section profile and morphology of the worn surface. (a), (c), (e), (g) Without ultrasound; (b), (d), (f), (h) With ultrasound
此外,相较于未施加超声的试样,磨痕表面有多处细小的剥落坑,进一步采取少量磨屑进行形貌拍摄,可见超声作用下的试样磨屑相较于无超声作用下更为细小。在摩擦磨损时,涂层会剥落一部分磨屑颗粒,对磨损表面产生耕犁和微切削作用,在材料表面留下沟状痕迹,而脱落的磨粒在载荷的作用下会压入摩擦面,并挤压出层片状的磨屑(图8(g)),同时在涂层中形成犁沟[31];在超声作用下,质地更为坚硬的涂层组织阻碍了磨屑对涂层进一步的磨损,产生了较小的磨屑(图8(h)),犁沟也相对较浅。
2.4 超声辅助激光熔覆复合涂层耐腐蚀性能分析
图9为WC颗粒强化涂层在3.5%氯化钠模拟海水溶液中的动态电位极化曲线,有无超声的试样均表现出相似的曲线走势,分别在−0.40 V和−0.39 V开始发生腐蚀,进入钝化区,随后分别在1.05 V和1.04 V附近钝化膜被击穿发生点蚀,其中无超声的试样腐蚀电流密度曲线高于有超声的试样,在各电位值下都相对较大,腐蚀电流密度分别为5.20 μA/cm2和2.13 μA/cm2。有无超声试样的电化学腐蚀参数如表3所示。
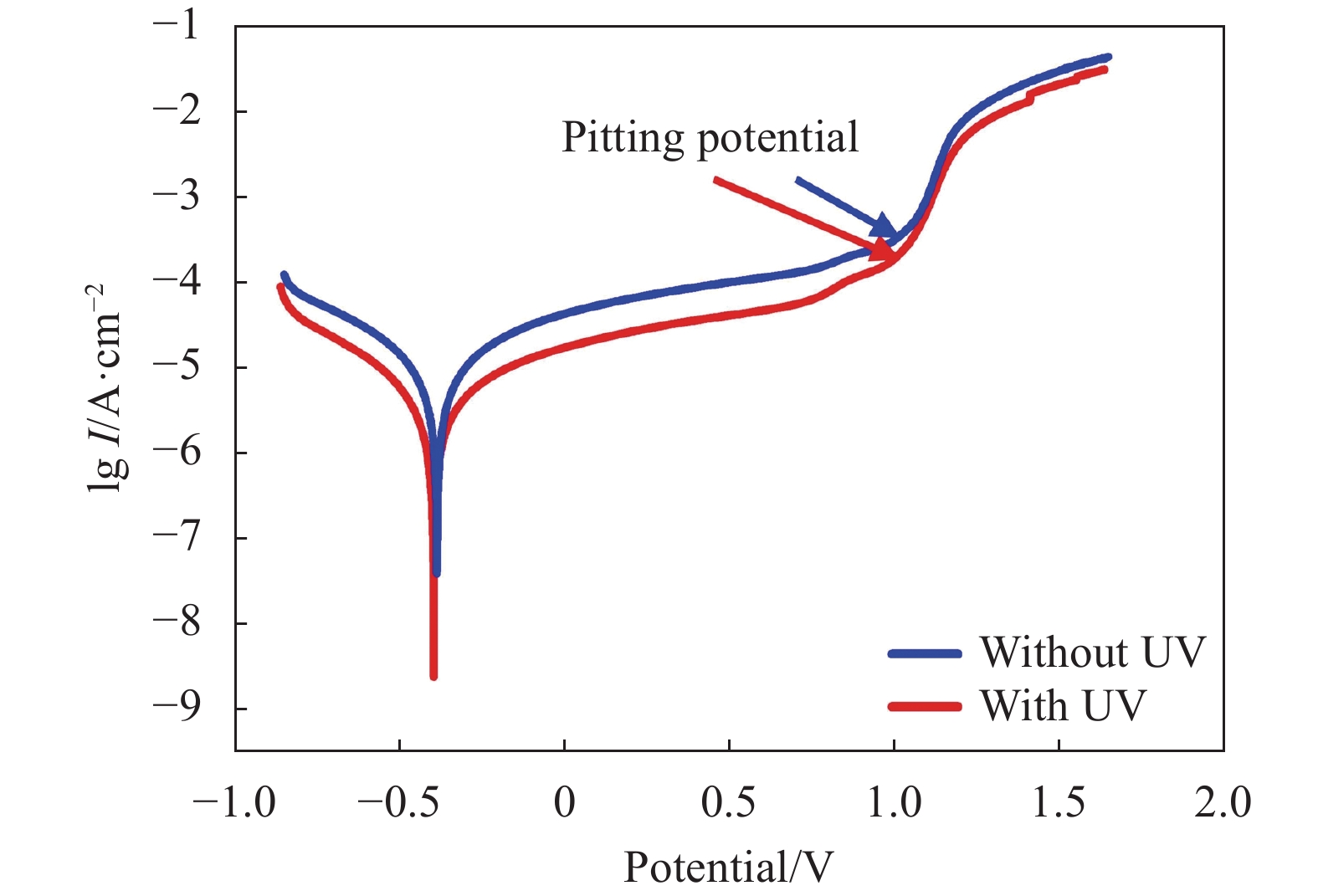
图 9. 有无超声试样动态电位极化曲线
Fig. 9. Dynamic potential polarization curves of samples with and without ultrasound
表 3. 电化学腐蚀参数
Table 3. Electrochemical corrosion parameters
|
为了进一步验证超声对WC颗粒强化涂层耐蚀性能的影响,进行了电化学阻抗谱(EIS)分析,所选择的等效电路模型为R(Q(R(QR))),如图10(c)所示,其中,Rs表示未补偿溶液电阻,Rct表示电荷转移电阻,Rf表示钝化膜电阻,CPE1和CPE2表示恒相元件。图10(a)为有无超声作用下的奈奎斯特曲线,其由两个部分组成:实部Z′和虚部Z′′。电容弧表示熔覆层与电解质界面的腐蚀反应,电容阻抗环的半径越大,其耐腐蚀性越好。超声作用下熔覆层表现出更大的电容弧,即更好的耐蚀性能。图10(b)为有无超声试样的波德图。在低频段,超声作用下试样曲线阻抗模量|Z|更高,相角值更大,表明腐蚀界面处存在更致密的钝化膜;在高频范围内,超声作用下的试样曲线同样拥有更高的阻抗模量|Z|。一般来说,阻抗模量|Z|越大,材料的耐蚀性能越好,相角的振幅与材料的电解质渗透阻力直接相关,较高的相角表示材料渗透阻力增强,具有更好的耐腐蚀性能。对比有无超声作用下试样的|Z|值和相角值,超声作用下的WC颗粒强化涂层具有更强的渗透阻力,即更好的耐腐蚀性能。
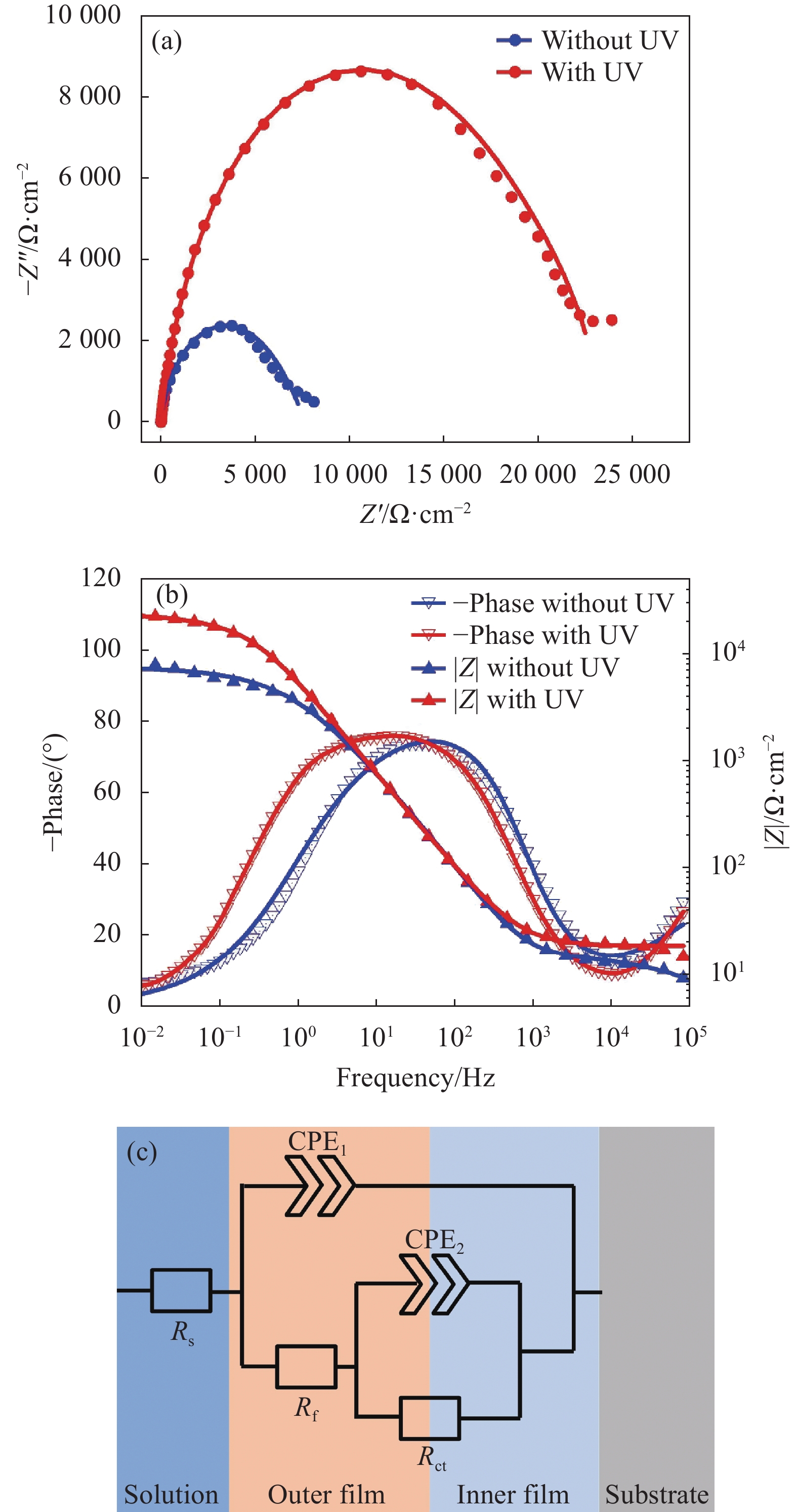
图 10. 有无超声试样在3.5 wt% NaCl溶液中的阻抗谱。(a) 奈奎斯特图;(b) 波德图;(c)等效电路图
Fig. 10. EIS of samples in 3.5 wt% NaCl solution with and without ultrasound. (a) Nyquist plot; (b) Bode plot; (d) Equivalent circuit mode
由于无超声的试样中组织和元素分布不均匀,这种不均匀的涂层结构会产生微小电流加剧腐蚀,大幅降低材料的耐蚀性能[32]。而超声振动的引入不仅会均匀复合涂层中元素的分布,其细化效果还会使得晶粒增加,晶界变多,一般来说,腐蚀通道的增加会降低熔覆层的耐蚀性能,但是超声对熔覆层中元素偏析的抑制提高了元素的均匀程度,减少了局部微原电池的数量,此时更高密度的晶界加速钝化膜的生长,更易形成致密的钝化膜,阻碍阳极反应的进行,整体腐蚀电流降低。同时,均匀的组织和元素分布提高了电解质的渗透阻力,最终提高了其抗腐蚀性能[33-34]。
3 结 论
文中采用超声辅助激光熔覆技术在316L不锈钢表面制备了WC颗粒强化涂层,对比分析了有无超声作用下涂层的组织形貌、硬度、耐磨性能以及耐腐蚀性能,结果如下:
1)无超声的熔覆层中,WC周围存在大量柱状晶,同时伴随着部分元素偏析带,由于超声的声空化效应,有超声的熔覆层中WC周围晶粒平均尺寸从101.0 μm降至59.6 μm,没有明显的偏析现象;
2)无超声WC颗粒强化涂层平均显微硬度为310 HV0.1,WC周围的硬度从480 HV0.1下降到320 HV0.1,由于超声对组织的均匀化,WC颗粒强化涂层平均显微硬度为425 HV0.1,WC周围的硬度从426 HV0.1下降到413 HV0.1;
3)无超声的试样失重量和磨损率分别为8.8 mg和0.0438 mg/m,有超声的试样失重量和磨损率分别为6.5 mg和0.0323 mg/m,无超声的试样磨痕犁沟最大深度约为53 μm,有超声的试样磨痕犁沟仅26 μm左右,超声振动对硬度的均匀提升使磨损率显著降低;
4)有无超声的熔覆层电化学试样腐蚀电流密度分别为2.13 μA/ cm2和5.20 μA/cm2,超声细化晶粒加速钝化膜生成,提升了复合涂层的耐蚀性能。
[1] Gou Wenjuan, Zhang Hui, Li Huiping, , et al. Effects of silica sand on synergistic erosion caused by cavitation, abrasion, and corrosion[J]. Wear, 2018, 412-413(C): 120-126.
[6] 高东强, 王蕊, 陈威, , et al. 激光熔覆改善材料性能的研究进展[J]. 热加工工艺, 2017, 46(12): 14-18.
Gao D, Wang R, Chen W, . Research progress of improving material properties by laser cladding[J]. Hot Working Technology, 2017, 46(12): 14-18.
[7] Wang W Z, Zhang C, Zhang Z, , et al. Effects of carbides on abrasion-corrosion performance of carbide reinforced composites in saline silica slurries[J]. Wear, 2023, 526-527: 204949.
[10] 李倩, 陈发强, 王茜, , et al. 激光熔覆WC增强Ni基复合涂层的研究进展[J]. 表面技术, 2022, 51(2): 129-143.
Li Q, Chen F, Wang Q, . Research progress of laser-cladding WC reinforced Ni-based composite coating[J]. Surface Technology, 2022, 51(2): 129-143.
[13] Wang X Y, Zhou S F, Dai X Q, , et al. Evaluation and mechanisms on heat damage of WC particles in Ni60/WC composite coatings by laser induction hybrid cladding[J]. International Journal of Refractory Metals & Hard Materials, 2017, 64: 234-241.
[14] 刘洪喜, 冷凝, 张晓伟, , et al. 40 Cr刀具表面激光熔覆WC/Co50复合涂层的微观组织及其磨损性能[J]. 红外与激光工程, 2016, 45(1): 0120001.
[16] 李英. 激光熔覆niwc复合涂层的组织与冲蚀磨损性能研究[D]. 河南理工大学, 2021.
Li Ying. Study on the microstructure erosion wear properties of laser cladding NiWC composite coating [D]. Jiaozuo: Henan Polytechnic University, 2021. (in Chinese)
[17] Jayaraj J, Elo R, Surreddi K B, , et al. Electrochemical and passivation behavior of a corrosion-resistant WC-Ni (W) cemented carbide in synthetic mine water[J]. International Journal of Refractory Metals & Hard Materials, 2023, 114: 106227.
[19] 宋诗英, 王梁, 胡勇, , et al. 稳态磁场辅助激光熔注制备梯度涂层[J]. 中国激光, 2016, 43(5): 0503005.
[21] 姚喆赫, 沈奇艳, 葛宏江, , et al. 超声对激光熔覆成形中熔池润湿行为的影响研究[J]. 表面技术, 2022, 51(10): 20-29.
Yao Zhehe, Shen Qiyan, Ge Hongjiang, . Influence of ultrasound on the wetting behavior of molten pool in laser cladding[J]. Surface Technology, 2022, 51(10): 20-29.
[26] 聂学武, 周建忠, 徐家乐, , et al. 超声振幅对激光熔覆WC/IN718复合涂层组织及性能的影响[J]. 表面技术, 2020, 49(9): 206-214.
[27] Barr C, Da Sun S, Easton M, , et al. Influence of macrosegregation on solidification cracking in laser clad ultra-high strength steels[J]. Surface & Coatings Technology, 2018, 340: 126-136.
[28] Antoni-zdziobek A, Shen J Y, Durand-charre M. About one stable and three metastable eutectic microconstituents in the Fe-W-C system[J]. International Journal of Refractory Metals & Hard Materials, 2008, 26(4): 372-382.
[34] Xu X, Du J L, Luo K Y, , et al. Microstructural features and corrosion behavior of Fe-based coatings prepared by an integrated process of extreme high-speed laser additive manufacturing[J]. Surface & Coatings Technology, 2021, 422: 127500.
Article Outline
姚喆赫, 戴温克, 邹朋津, 余沛坰, 王发博, 迟一鸣, 孙振强, 张群莉, 姚建华. 超声对激光熔覆WC颗粒强化涂层耐磨防腐性能的影响(特邀)[J]. 红外与激光工程, 2024, 53(1): 20230542. Zhehe Yao, Wenke Dai, Pengjin Zou, Peijiong Yu, Fabo Wang, Yiming Chi, Zhenqiang Sun, Qunli Zhang, Jianhua Yao. Effects of ultrasonic vibration on wear and corrosion resistance of WC particles reinforced coating produced by laser cladding (invited)[J]. Infrared and Laser Engineering, 2024, 53(1): 20230542.