LPBF成形新型定向凝固镍基高温合金基础工艺研究
The traditional directional solidification process has made outstanding contributions to improving the thermal-mechanical fatigue performance and service cycle of turbine blades. However, the traditional directional solidification process has limitations. It not only hampers the formation of new generation of turbine blades with complex cooling structures, but also results in a coarse microstructure and microporosity, along with γ/γ' eutectic. These issues restrict further development of directional solidification turbine blades. Laser powder bed fusion (LPBF) technology can form almost any complex shape of metal components. Meanwhile, LPBF technology has an extremely high cooling rate (103?106 K/s) and temperature gradient (103?105 K/s), which is expected to eliminate the above-mentioned defects and form a finer microstructure. In this study, the densification behavior, microstructure, and solidification grain orientation of a new directionally solidified nickel-based superalloy, ZGH451, fabricated using LPBF are investigated. The feasibility of using LPBF technology to form a directionally solidified nickel-based superalloy, ZGH451, is confirmed.
A self-developed LPBF equipment is used for ZGH451 experiments. The equipment includes a fiber laser with a spot diameter of approximately 80 μm. Cubes measuring 10 mm×10 mm×5 mm are produced on 45# cast steel plates. Optical micrographs (OM) are collected using a optical microscope. The microstructures of the samples are observed using a scanning electron microscope (SEM). An SEM with electron backscattered diffraction (EBSD) is used to characterize the grain morphology and orientation characteristics. The EBSD scanning step is performed at 1.5 μm. The final calibration rates are all greater than 95%. The inter-dendritic precipitates of the samples are analyzed using a transmission electron microscope (TEM). The tensile specimens are tested on a high-precision electronic universal testing machine at a constant drawing rate of 2 mm/min.
This study successfully forms a crack-free, high-density (99.9%) ZGH451 sample. The primary dendrite spacing is less than 1 μm, and the secondary dendrite is underdeveloped. There are TiC and TaC particles precipitated between dendrites, and there is no obvious γ/γ' eutectic. The typical microscopic characteristics of cracks are observed by SEM, as shown in Figs. 6(a) and (b). The cracks mainly originate at the bottom of the molten track and grow along the building direction. The EBSD results indicate that the cracks [Fig.6(c)] exhibit evident intergranular cracking characteristics. Therefore, the cracks are determined to be solidification cracks. The occurrence of solidification cracks in the ZGH451 alloy formed via LPBF is closely related to its thermal stress state and microstructural characteristics during solidification. In this study, when the laser power is reduced from 200 W to 150 W, a crack-free sample is obtained at an appropriate laser energy density. A lower laser power or laser energy density leads to a faster cooling rate and finer microstructure, making it easier to adapt to the solidification stress at the end of solidification in the molten track. In addition, compared with the traditional directionally solidified superalloy, the ZGH451 alloy increases the proportion of the Hf element. Hf transforms the MC carbides from a continuous distribution to a dispersed distribution, improving the stability of the grain boundaries and carbides and suppressing cracking. In addition, researchers have found that the directional solidification trend of directionally solidified nickel-based superalloys formed by LPBF tends to weaken with increasing building height. Therefore, a crack-free ZGH451 sample (building height of 5 mm) is selected for characterization by EBSD at the bottom, middle, and top positions. As shown in Fig. 9, within the building height range used in this study, the directional solidification trend of the ZGH451 alloy is not weakened, and the directional solidification trends of the middle and top of the sample are more evident than that of the bottom of the sample. This phenomenon relates not only to the strong directional solidification tendency of the new directionally solidified alloy but also to the substrate used. Specifically, the extremely high heat input of the LPBF produces an extremely high temperature gradient from the top to the bottom along the building direction. However, because the substrate used in this study is a stainless-steel plate, the grain orientation at the bottom of the sample is relatively disordered. Under the competitive growth mechanism, as the building height continues to increase, the front edges of the grains deviating from the temperature gradient gradually lag behind those of the preferentially oriented grains and are eliminated. Due to the extremely fine microstructure of the sample, its yield strength [(979.5±31.0)MPa] is equivalent to that of the third-generation single-crystal nickel-based superalloy (1017 MPa). However, anisotropic grains are still generated by the lateral heat flux caused by the overlap of the molten tracks.
Within the process window of 150 W laser power, 0.02 mm layer thickness, 600?800 mm/s scanning velocity, and 0.06?0.08 mm hatch spacing, crack-free and high-density (99.9%) samples can be obtained. The solidified structure is primarily composed of columnar grains that grow along the build direction. The primary dendrite spacing is less than 1 μm, and the secondary dendrite is underdeveloped. There are TiC and TaC particles precipitated between dendrites, and there is no obvious γ/γ' eutectic. The as-built ZGH451 exhibits a texture along [001], but anisotropic grains remain owing to the complex heat flow in the overlapping area between adjacent molten tracks. The feasibility of using LPBF technology to form a directionally solidified nickel-based superalloy, ZGH451, has been confirmed; however, the problem of inconsistent orientation caused by complex heat flows still needs to be solved.
1 引言
涡轮叶片是航空发动机的关键零部件,长期在高温环境下服役,承受复杂交变载荷,工况条件极为恶劣[1-4]。长期以来,涡轮叶片一直采用承温能力较高的镍基高温合金制备。但是,随着现代先进航空发动机的快速发展,涡轮温度已普遍超过1800 K,远超常规镍基高温合金的承温极限[1-4]。因此,新一代涡轮叶片普遍采用空心冷却结构,工作状态下叶轮表面形成冷却气膜,进而将叶片基体温度降至1300 K以下[1-4]。同时,采用定向凝固技术消除镍基高温合金的横向晶界,也是进一步提高涡轮叶片承温能力,从而延长服役寿命的有效方案。目前,以液态金属冷却法(LMC法)为代表的传统定向凝固工艺已经在提高涡轮叶片热机械疲劳性能和服役周期方面做出了突出贡献[5]。但是,传统定向凝固工艺不仅难以成形具有复杂冷却结构的新一代涡轮叶片,还存在叶片显微组织粗大(一次枝晶间距可达几百微米)、易出现显微疏松、γ/γ'共晶难消除等问题[6],这极大地制约了定向凝固复杂冷却结构涡轮叶片的进一步发展。
激光粉末床熔融(LPBF)技术是极具发展潜力的激光增材制造技术[7-9],其采用“粉末逐层预铺+激光逐层熔凝沉积”的工艺原理,可以成形几乎任意复杂形状的金属零部件。同时,LPBF技术具有极高的熔池冷却速度(103~106 K/s)和温度梯度(103~105 K/s)[10-11],不但能够细化镍基高温合金的显微组织并消除共晶和显微疏松,还有望成形定向性极好的微细柱晶组织。因此,LPBF是定向凝固复杂冷却结构涡轮叶片的理想技术。
近年来,国内外学者对LPBF成形定向凝固镍基高温合金开展了初步研究。华中科技大学李帅[12]通过将LPBF技术采用的高斯光斑整形为均匀光斑,在一定条件下实现了非定向凝固专用镍基高温合金Inconel 718的定向凝固生长。但是,定向生长高度仅可维持约3 mm。英国伯明翰大学Carter等[13]利用LPBF实现了传统第一代定向凝固镍基高温合金CM247LC的定向生长,但成形层内部存在较多裂纹。华中科技大学潘爱琼等[14-15]建立了LPBF成形传统第一代单晶镍基高温合金SRR99的定向生长工艺窗口,成功制备出高度为2 mm的三维实体,晶体取向差几乎全部在10°范围内。然而,随着成形高度的进一步增加,定向凝固生长无法维持。同时,该研究未能消除成形材料中的凝固裂纹。华中科技大学李继康等[2]研究了LPBF成形传统第四代单晶镍基高温合金DD91的单道形貌及晶体取向,能够在较高激光功率(300 W)和较低扫描速度(500 mm/s)下获得形貌扁平、取向一致的单道。现有研究证实了采用LPBF技术成形定向凝固镍基高温合金的可行性,但成形对象都是传统定向凝固镍基高温合金甚至非定向凝固专用合金,其成分并未针对LPBF工艺极高的冷却速度和温度梯度进行优化,存在极易产生裂纹且定向生长难以维持等共性问题。
针对以上问题,本文设计了一款新型定向凝固镍基高温合金ZGH451。ZGH451是一款专为增材制造设计的定向凝固镍基高温合金,通过调控元素占比降低增材过程中开裂的倾向性。采用自主研制的LPBF设备开展了该合金的LPBF成形基础工艺研究。初步揭示了工艺参数对ZGH451合金致密度和冶金缺陷的影响规律,成功制备出无裂纹、高致密、显微组织细密及定向凝固特征和力学性能较好的镍基高温合金样品。
2 实验方法
2.1 LPBF实验材料及工艺参数
LPBF实验所选用的粉末原料为气雾化ZGH451镍基高温合金粉末。粉末元素成分如
表 1. ZGH451镍基高温合金的名义化学成分(质量分数,%)
Table 1. Nominal chemical compositions of ZGH451 nickel-based superalloy (mass fraction, %)
|
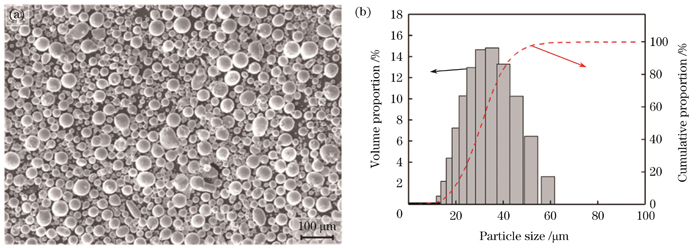
图 1. 气雾化ZGH451镍基高温合金粉末。(a)颗粒形貌;(b)粒径分布
Fig. 1. Gas-atomized ZGH451 nickel-based superalloy powder. (a) Particle morphology; (b) particle size distribution
采用自研的LPBF-300设备,开展ZGH451镍基高温合金的LPBF成形实验。LPBF-300搭载有一台光纤激光器,能够输出1.07 μm波长连续高斯激光,最大输出功率为500 W,光斑直径约为80 μm。以45号铸造钢板为基板开展基础工艺实验,成形对象为尺寸为10 mm×10 mm×5 mm的立方体。基于一系列预实验,确定可稳定成形、样品无明显宏观缺陷的工艺实验参数范围,如
表 2. LPBF实验参数
Table 2. Parameters for LPBF experiment
|
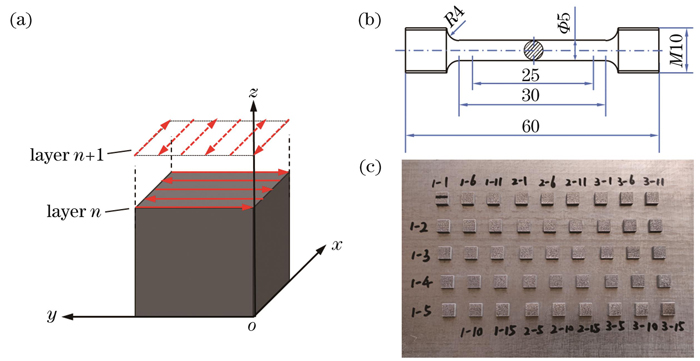
图 2. LPBF成形ZGH451镍基高温合金。(a)激光扫描策略;(b)拉伸试样尺寸;(c)成形样品
Fig. 2. Forming ZGH451 nickel-based superalloy by LPBF. (a) Laser scanning strategy; (b) size of tensile specimen; (c) formed samples
2.2 微观结构及力学性能表征
LPBF成形实验结束后,采用线切割技术从成形基板上切取ZGH451样品。使用图像法测量样品的致密度,具体方法为:首先用砂纸将ZGH451样品的竖直截面(与打印方向平行)打磨平整,并用颗粒粒径为2.5 μm的金刚石研磨剂将其抛光;然后,使用光学显微镜(OM)在竖直截面上随机选取3个区域拍摄OM图,并在Image-Pro Plus 6.0软件中对OM图片进行二值化处理;通过适当的阈值选取自动标定缺陷区域,得到缺陷区域的面积百分比;最后,求取3个区域的缺陷面积百分比的平均值,并与1相减得到相对致密度。
为获取样品的显微形貌,选用王水(10 mL HNO3+30 mL HCl)在机械抛光后的样品表面擦拭10 s,然后采用光学显微镜和电子扫描显微镜(SEM)进行观察。采用背散射电子衍射仪(EBSD)表征样品的晶粒形貌与取向特征。为保证EBSD标定率,采用电解液(体积分数为10%的HClO+体积分数为90%的CH3OH)在10 V电压下对样品表面实施25 s的电解抛光。EBSD测试时扫描步长为1.5 μm,最终标定率均大于95%。采用透射电子显微镜(TEM)分析样品的枝晶间析出相。为获得满足TEM测试要求的样品,首先利用线切割机从块体样品上切出厚度为0.3 mm的薄片,然后利用砂纸将其研磨至50 μm厚。进一步地,将薄片冲压成直径为3 mm的圆片,利用离子减薄仪在8 kV(离子减薄仪电子枪电压)/8°(入射角度)入射模式下刻蚀至样品出现孔洞,然后依次在6 kV/6°、4 kV/4°、2 kV/2°的条件下刻蚀30、20、10 min以获得薄区。
选取最佳工艺参数制备
3 分析与讨论
3.1 致密化行为与冶金缺陷
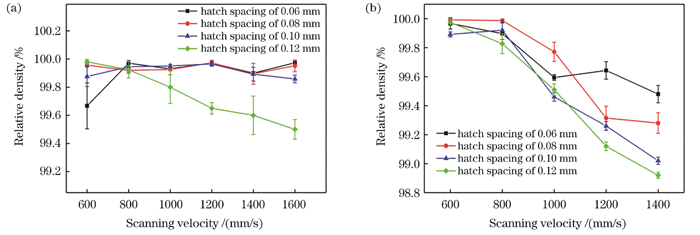
图 3. 不同激光功率下LPBF成形ZGH451合金致密度随扫描速度与扫描间距的变化曲线。(a) 200 W; (b) 150 W
Fig. 3. Variation curves of relative density of ZGH451 alloy formed by LPBF with scanning speed and scanning spacing under different laser powers. (a) 200 W; (b) 150 W
当激光功率降低至150 W时,扫描间距与致密度的关系依旧较为复杂。当扫描速度较低(≤800 mm/s)时,扫描间距与致密度的相关性差;随着扫描速度的提高,致密度逐渐表现出随扫描间距的减小而提升的趋势。相比之下,致密度与扫描速度的关联性更强。在所有扫描间距下,致密度都基本呈现出随扫描速度的提升而降低的趋势。与激光功率为200 W时的情况相比,致密度在不同扫描速度和扫描间距组合下的变化幅度更大,所能得到的最高致密度为99.9%,高于200 W时的最高值密度99.8%。
为了更加清晰地描述工艺参数整体对冶金缺陷的影响规律,引入激光能量密度E(J/mm3)[16]。其定义为单位体积内激光束提供的能量:
式中:P为激光功率(W);v为激光扫描速度(mm/s);h为层厚(mm);s为扫描间距(mm)。按照激光能量密度E与样品致密化状态和冶金缺陷特征的对应关系,可以绘制出不同激光功率下的工艺窗口,如
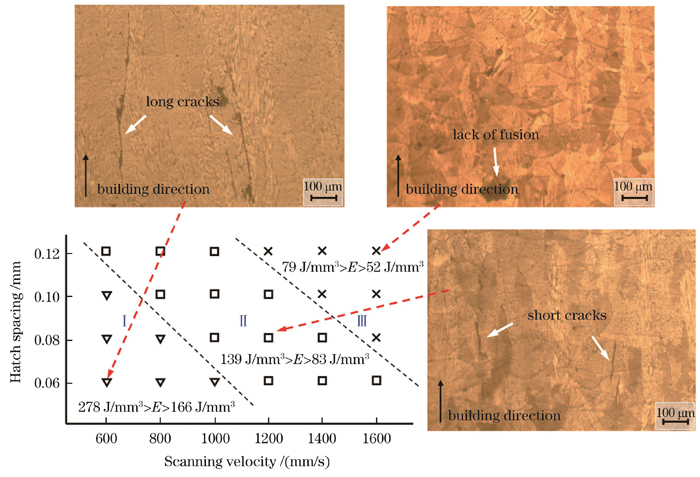
图 4. 激光功率为200 W时的工艺窗口和典型样品的冶金缺陷特征图
Fig. 4. Process window and metallurgical defect feature maps of typical samples at laser power of 200 W
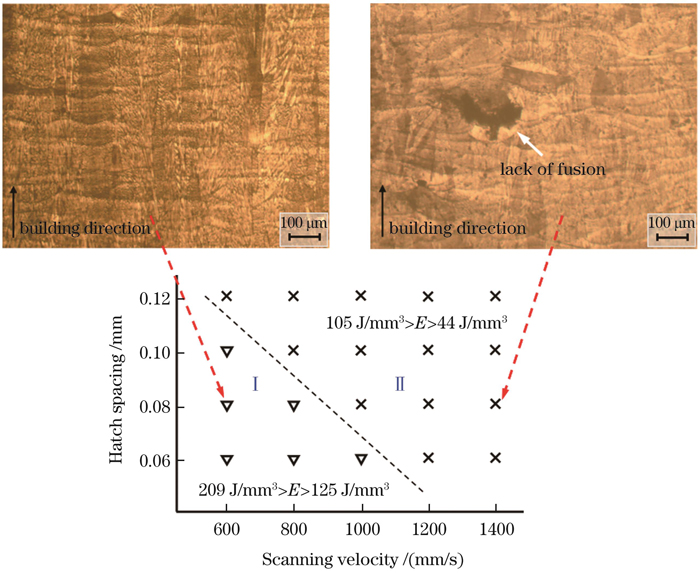
图 5. 激光功率为150 W时的工艺窗口和典型样品的冶金缺陷特征图
Fig. 5. Process window and metallurgical defect feature maps of typical samples at laser power of 150 W
从
从
3.2 裂纹产生与消除机理
如引言所述,凝固裂纹一直是LPBF成形定向凝固镍基高温合金的典型冶金缺陷[2,13-15]。为验证在LPBF成形ZGH451样品中发现的裂纹是否为凝固裂纹,对裂纹的典型显微特征进行了SEM观察,如
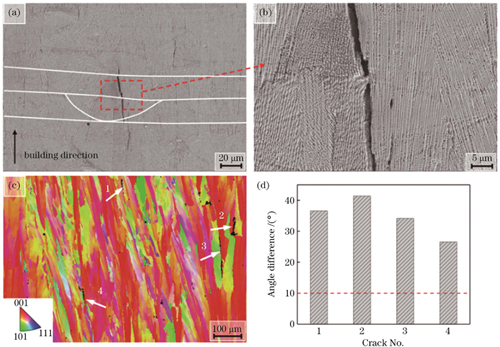
图 6. LPBF成形ZGH451合金裂纹的典型分布特征。(a)SEM图;(b)图6(a)的局部放大;(c)EBSD晶粒取向分布图;(d)裂纹两侧的晶粒角度偏差
Fig. 6. Typical distribution characteristics of cracks in ZGH451 superalloy formed by LPBF. (a) SEM image; (b) local magnification of Fig. 6 (a); (c) EBSD grain orientation distribution; (d) grain angle difference on both sides of crack
大量研究表明,凝固裂纹一般萌生于熔池凝固末期的固液混合糊状区,这是因为糊状区无法抵抗凝固收缩应力,枝晶间液膜被撕裂[17-18]。而相邻晶粒较大的取向差可能导致应力更加集中于两晶粒形成的晶界处,裂纹更加倾向在此处萌生。因此,LPBF成形ZGH451合金是否产生凝固裂纹与其在凝固期间的热应力状态和凝固组织特征密切相关。在本文中,当激光功率为200 W时,单位面积内的凝固裂纹长度随激光能量密度E的下降而减小;当激光功率从200 W降低至150 W时,可在适当的激光能量密度E下获得无裂纹样品。造成这些现象的一个重要原因在于,LPBF成形过程中的热应力一般随激光功率或激光能量密度的降低而减小[19-20]。另一方面,根据激光加工冶金学基本原理,LPBF成形期间的熔池冷却速度通常随激光功率或激光能量密度E的降低而提升,并导致显微组织的细化[21-22]。现有研究表明,金属的熔凝组织越细密,越容易在熔池凝固末期适应凝固应力[23-24]。因此,这应该也是LPBF成形ZGH451合金可在较低的激光功率下实现无裂纹成形的一个原因。此外,还需要指出的是,相较于传统的定向凝固高温合金,本文专门设计的ZGH451合金增加了Hf占比。Hf具有使MC碳化物由连续分布向弥散分布转变以及提高晶界和碳化物稳定性的作用,能够在一定程度上抑制开裂[25-26]。
3.3 显微组织
选取无裂纹、高致密ZGH451合金样品进行显微组织表征,典型SEM图如

图 7. LPBF成形无裂纹、高致密ZGH451合金典型样品的SEM图。(a)显微组织整体形貌;(b)熔道底部粗化的枝晶;(c)枝晶间析出物;(d)熔道边缘异取向枝晶。
Fig. 7. SEM images of typical samples of crack-free and high-density ZGH451 superalloy formed by LPBF. (a) Overall morphology of microstructure; (b) coarsened dendrites at bottom of molten track; (c) precipitates between dendrites; (d) different oriented dendrites at edge of molten track
观察
ZGH451与传统单晶/定向凝固镍基高温合金类似,可能会由于Al、Ti等元素在枝晶间偏析而形成熔点低、高温强度差的γ/γ'共晶,在持久断口中常能观察到裂纹起源于γ/γ'共晶。现有研究表明,γ/γ'共晶的形貌和含量会随着冷却速度的变化而改变[30]。因此,无法单从SEM形貌判断白色颗粒物是否为γ/γ'共晶。需要对高致密、无裂纹样品进行TEM表征以确定白色颗粒物的实际成分。
LPBF成形典型高致密、无裂纹ZGH451样品的TEM分析结果如
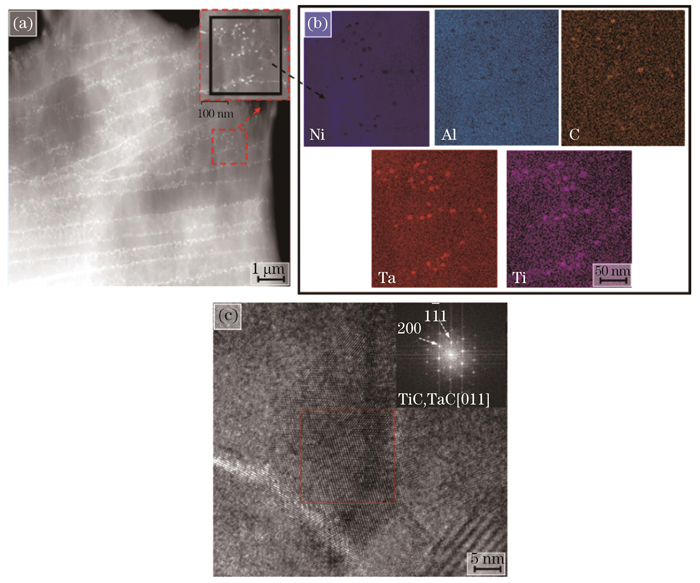
图 8. LPBF成形无裂纹、高致密ZGH451合金样品的析出相特征分析。(a)TEM暗场像;(b)EDX元素面分布;(c)HR-TEM像及电子衍射斑点图
Fig. 8. Analysis of precipitated phase characteristics of crack-free and high-density ZGH451 superalloy samples formed by LPBF. (a) TEM dark field image; (b) EDX element surface distribution; (c) HR-TEM image and electron diffraction spot diagram
3.4 凝固晶粒取向
在此前的研究中,研究者们发现,LPBF成形镍基高温合金的定向生长趋势往往随构建高度的增加而减弱[13-15]。因此,特意选取外形尺寸为10 mm×10 mm×5 mm(构建高度为5 mm)的无裂纹、高致密LPBF成形ZGH451合金典型样品,分别对其底部、中部及顶部进行EBSD表征,对应的EBSD图谱如

图 9. LPBF成形无裂纹、高致密ZGH451样品不同位置的EBSD图谱。(a)底部晶粒形态与取向特征的反极图(IPF);(b)中部晶粒形态与取向特征的IPF图;(c)顶部晶粒形态与取向特征的IPF图;(d)底部{001}特征晶面极图;(e)中部{001}特征晶面极图;(f)顶部{001}特征晶面极图
Fig. 9. EBSD maps of crack-free and high-density ZGH451 superalloy samples formed by LPBF at different positions. (a) Inverse pole figure (IPF) of grain morphology and orientation characteristics at bottom; (b) IPF of grain morphology and orientation characteristics at middle; (c) IPF of grain morphology and orientation characteristics at top; (d) pole figure of {001} characteristic crystal plane at bottom; (e) pole figure of {001} characteristic crystal plane at middle; (f) pole figure of {001} characteristic crystal plane at top
值得说明的是,尽管LPBF成形ZGH451合金表现出了较强的定向凝固特征,但在
3.5 力学性能
对LPBF成形无裂纹、高致密ZGH451合金样品进行了室温拉伸实验。结果表明,其屈服强度(YS)为(979.5±31.0)MPa、极限抗拉强度(UTS)为(1062.5±81.0)MPa、延伸率(EL)为(3.48±0.20)%。
表 3. LPBF成形ZGH451与其他单晶高温合金的屈服强度对比[35-36]
Table 3. Comparison of yield strength between ZGH451 formed by LPBF superalloy and other single crystal superalloys[35-36]
|
为研究断裂机理,在SEM下对样品断口进行观察,如
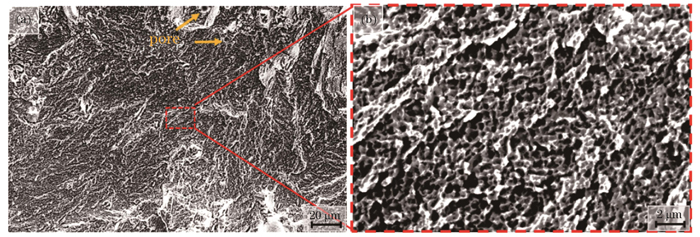
图 10. LPBF成形无裂纹、高致密ZGH451的拉伸断口SEM图
Fig. 10. SEM images of tensile fractures of crack-free and high-density ZGH451 formed by LPBF
4 结论
研究了LPBF制备新型定向凝固镍基高温合金ZGH451的基础工艺,取得了如下主要结论。
1) 样品的主要冶金缺陷是未熔合和裂纹,通过优化激光功率、扫描速度和扫描间距等工艺参数,能够获得无裂纹、高致密样品(致密度为99.9%);
2) 裂纹主要萌生于熔池底部,并具有沿晶开裂的特征,主要位于大角度晶界处,属于凝固裂纹。通过降低激光功率,能够有效降低凝固热应力并细化组织,从而消除凝固裂纹;
3) LPBF成形无裂纹、高致密ZGH451合金的显微组织主要由一次枝晶间距小于1 μm的微细柱状枝晶构成,枝晶间存在MC型碳化物,并未观察到有害的γ/γ'共晶相;
4) EBSD结果表明,总高度为5 mm的样品整体沿[001]构建方向定向生长。随着构建高度的增加,定向凝固趋势先增加后基本保持不变。但是,由于相邻熔道之间产生的侧向热流改变了熔道边缘区域的温度梯度方向,LPBF成形ZGH451合金内部仍存在少量异取向晶粒,仍需进一步研究相关的控制方法;
5) LPBF成形无裂纹、高致密ZGH451合金的力学性能较为优异,其室温屈服强度优于传统定向工艺制备的第二代单晶高温合金(DD6和CMSX-4),并且与第三代单晶高温合金(DD9)性能相当。
[1] Pierret S, Evans A, Paradowska A M, et al. Combining neutron diffraction and imaging for residual strain measurements in a single crystal turbine blade[J]. NDT & E International, 2012, 45(1): 39-45.
[2] 李继康, 张振武, 杨源祺, 等. 激光选区熔化DD91镍基单晶高温合金的单道形貌、晶体取向和微观组织[J]. 中国激光, 2022, 49(14): 1402103.
[3] Nkoi B, Pilidis P, Nikolaidis T. Performance of small-scale aero-derivative industrial gas turbines derived from helicopter engines[J]. Propulsion and Power Research, 2013, 2(4): 243-253.
[4] 刘维伟, 张定华, 史耀耀, 等. 航空发动机薄壁叶片精密数控加工技术研究[J]. 机械科学与技术, 2004, 23(3): 329-331.
Liu W W, Zhang D H, Shi Y Y, et al. Study on net-shape NC machinging technology of thin-balde of aeroengine[J]. Mechanical Science and Technology, 2004, 23(3): 329-331.
[5] 刘林, 张军, 沈军, 等. 高温合金定向凝固技术研究进展[J]. 中国材料进展, 2010, 29(7).
Liu L, Zhang J, Shen J, et al. Advances in directional solidification techniques of superalloys[J]. Materials China, 2010, 29(7).
[6] 刘林, 孙德建, 黄太文, 等. 高梯度定向凝固技术及其在高温合金制备中的应用[J]. 金属学报, 2018, 54(5): 615-626.
Liu L, Sun D J, Huang T W, et al. Directional solidification under high thermal gradient and its application in superalloys processing[J]. Acta Metallurgica Sinica, 2018, 54(5): 615-626.
[7] 顾冬冬, 张红梅, 陈洪宇, 等. 航空航天高性能金属材料构件激光增材制造[J]. 中国激光, 2020, 47(5): 0500002.
[8] 文世峰, 季宪泰, 周燕, 等. 激光选区熔化成形模具钢的发展现状及前景[J]. 激光与光电子学进展, 2018, 55(1): 011404.
Wen S F, Ji X T, Zhou Y, et al. Development status and prospect of selective laser melting of mould steels[J]. Laser & Optoelectronics Progress, 2018, 55(1): 011404.
[9] Phan M A L, Fraser D, Gulizia S, et al. Horizontal growth direction of dendritic solidification during selective electron beam melting of a Co-based alloy[J]. Materials Letters, 2018, 228: 242-245.
[10] Khairallah S A, Anderson A. Mesoscopic simulation model of selective laser melting of stainless steel powder[J]. Journal of Materials Processing Technology, 2014, 214(11): 2627-2636.
[11] 蒋华臻, 房佳汇钰, 陈启生, 等. 激光选区熔化成形316L不锈钢工艺、微观组织、力学性能的研究现状[J]. 中国激光, 2022, 49(14): 1402804.
[12] 李帅. 激光选区熔化成形镍基高温合金的组织与性能演变基础研究[D]. 武汉: 华中科技大学, 2017.
LiS. Basic study on microstructure and properties evolution of nickel-based superalloy formed by laser selective melting[D]. Wuhan: Huazhong University of Science and Technology, 2017.
[13] Carter L N, Martin C, Withers P J, et al. The influence of the laser scan strategy on grain structure and cracking behaviour in SLM powder-bed fabricated nickel superalloy[J]. Journal of Alloys and Compounds, 2014, 615: 338-347.
[14] 潘爱琼, 张辉, 王泽敏. 选区激光熔化镍基单晶高温合金的熔池显微组织[J]. 激光与光电子学进展, 2017, 54(7): 071402.
[15] 潘爱琼, 张辉, 王泽敏. 镍基单晶高温合金选区激光熔化成形工艺及组织[J]. 中国激光, 2019, 46(11): 1102007.
[16] Simchi A. Direct laser sintering of metal powders: mechanism, kinetics and microstructural features[J]. Materials Science and Engineering: A, 2006, 428(1/2): 148-158.
[17] Ghaini F M, Sheikhi M, Torkamany M J, et al. The relation between liquation and solidification cracks in pulsed laser welding of 2024 aluminium alloy[J]. Materials Science and Engineering: A, 2009, 519(1/2): 167-171.
[18] Hatami N, Babaei R, Dadashzadeh M, et al. Modeling of hot tearing formation during solidification[J]. Journal of Materials Processing Technology, 2008, 205(1/2/3): 506-513.
[19] 边培莹, 尹恩怀. 选区激光熔化激光功率对316L不锈钢熔池形貌及残余应力的影响[J]. 激光与光电子学进展, 2020, 57(1): 011403.
[20] Norman A F, Drazhner V, Prangnell P B. Effect of welding parameters on the solidification microstructure of autogenous TIG welds in an Al-Cu-Mg-Mn alloy[J]. Materials Science and Engineering: A, 1999, 259(1): 53-64.
[21] Hunt J D. Steady state columnar and equiaxed growth of dendrites and eutectic[J]. Materials Science and Engineering, 1984, 65(1): 75-83.
[22] Chen Y, Chen H, Chen J Q, et al. Numerical and experimental investigation on thermal behavior and microstructure during selective laser melting of high strength steel[J]. Journal of Manufacturing Processes, 2020, 57: 533-542.
[23] Hu J, Shi Y N, Sauvage X, et al. Grain boundary stability governs hardening and softening in extremely fine nanograined metals[J]. Science, 2017, 355(6331): 1292-1296.
[24] Zhang H, Zhu H H, Nie X J, et al. Effect of zirconium addition on crack, microstructure and mechanical behavior of selective laser melted Al-Cu-Mg alloy[J]. Scripta Materialia, 2017, 134: 6-10.
[25] Chen Q Z, Jones N, Knowles D M. The microstructures of base/modified RR2072 SX superalloys and their effects on creep properties at elevated temperatures[J]. Acta Materialia, 2002, 50(5): 1095-1112.
[26] 余竹焕, 张洋, 翟娅楠, 等. C、B、Hf在镍基高温合金中作用的研究进展[J]. 铸造, 2017, 66(10): 1076-1081.
Yu Z H, Zhang Y, Zhai Y N, et al. The research progress of the role of C, B and Hf in nickel-based superalloy[J]. Foundry, 2017, 66(10): 1076-1081.
[27] Han G M, Yang Y H, Yu J J, et al. Temperature dependence of anisotropic stress-rupture properties of nickel-based single crystal superalloy SRR99[J]. Transactions of Nonferrous Metals Society of China, 2011, 21(8): 1717-1721.
[28] Liang Y J, Li J, Li A, et al. Experimental optimization of laser additive manufacturing process of single-crystal nickel-base superalloys by a statistical experiment design method[J]. Journal of Alloys and Compounds, 2017, 697: 174-181.
[29] 梁静静, 杨彦红, 周亦胄, 等. 激光立体成形镍基单晶高温合金显微组织研究[J]. 稀有金属材料与工程, 2017, 46(12): 3753-3759.
Liang J J, Yang Y H, Zhou Y Z, et al. Microstructures of nickel-base single-crystal superalloy prepared by laser solid forming[J]. Rare Metal Materials and Engineering, 2017, 46(12): 3753-3759.
[30] 崔红保, 郭景杰, 毕维生, 等. 高温度梯度下Al-In偏晶合金定向凝固组织的演化规律[J]. 金属学报, 2004, 40(12): 1253-1256.
Cui H B, Guo J J, Bi W S, et al. The evolution of unidirectional solidification microstructure of the Al-In monotectic alloys in high temperature gradient[J]. Acta Metallrugica Sinica, 2004, 40(12): 1253-1256.
[32] 孟翔, 田象军, 程序, 等. 激光增材制造DZ40M的微观组织及热处理研究[J]. 中国激光, 2018, 45(10): 1002008.
[33] Rappaz M, Gandin C A. Probabilistic modelling of microstructure formation in solidification processes[J]. Acta Metallurgica et Materialia, 1993, 41(2): 345-360.
[34] Gandin C A, Rappaz M. A coupled finite element-cellular automaton model for the prediction of dendritic grain structures in solidification processes[J]. Acta Metallurgica et Materialia, 1994, 42(7): 2233-2246.
[36] Sengupta A, Putatunda S K, Bartosiewicz L, et al. Tensile behavior of a new single-crystal nickel-based superalloy (CMSX-4) at room and elevated temperatures[J]. Journal of Materials Engineering and Performance, 1994, 3(1): 73-81.
[37] Song R J, Ponge D, Raabe D. Influence of Mn content on the microstructure and mechanical properties of ultrafine grained C-Mn steels[J]. ISIJ International, 2005, 45(11): 1721-1726.
Article Outline
周润森, 魏恺文, 梁静静, 陈佳, 李高航, 屈亮, 刘梦娜, 李祥友, 孙晓峰, 曾晓雁. LPBF成形新型定向凝固镍基高温合金基础工艺研究[J]. 中国激光, 2023, 50(24): 2402304. Runsen Zhou, Kaiwen Wei, Jingjing Liang, Jia Chen, Gaohang Li, Liang Qu, Mengna Liu, Xiangyou Li, Xiaofeng Sun, Xiaoyan Zeng. Basic Process of New Directional Solidification Nickel‑Based Superalloy Fabricated by Laser Powder Bed Fusion[J]. Chinese Journal of Lasers, 2023, 50(24): 2402304.