基于F型梁的光纤光栅加速度传感器
0 引言
振动信号能够表征物体振动的基本特征,可以准确及时地反映设备结构的工作状态[1],因此通过检测设备的振动信号,可以准确评估其健康状况,并判定损伤位置[2]。传统的拾振器多为电类传感器,在高电压、强磁场的复杂环境下,电类传感器易受电磁干扰,并且其金属结构可能产生放电,改变监测设备的磁场分布,从而降低设备安全运行的可靠性[3]。光纤布拉格光栅(Fiber Bragg Grating,FBG)加速度传感器具有本质防爆、抗电磁干扰、耐高温、易于组网等优点,特别适合高电压、强磁场特殊环境中的应用。
光纤光栅加速度传感器根据设计结构大致可以分为芯轴式[4-7]、膜片式[8-10]、铰链式[11-15]与梁式[16-18]等。芯轴式FBG加速度传感器的性能受弹性体影响较大,弹性体刚度与灵敏度相互制约[19],必须选择合适的弹性体;基于膜片式的FBG传感器大多适用于高频的地震勘探等环境,不适于拾取低频信号;基于铰链式的FBG传感器工作频率范围大,灵敏度高,但其原理结构复杂;梁式FBG振动传感器不仅结构简单,而且具有稳定性高、横向抗干扰能力强等优点,被广泛用于一维加速度的测量[20]。贾振安等[21]设计了一种悬臂梁传感器,其谐振频率为90 Hz,灵敏度为121 pm/g,之后根据实验结果对悬臂梁进行了疲劳分析,并改进优化了传感器尺寸,大大提高了悬臂梁的疲劳寿命,但其工作频率范围较窄;张绪成等[22]利用L型悬臂梁设计了一种光纤光栅加速度传感器,该传感器谐振频率为280 Hz,测量带宽为1~240 Hz,灵敏度为52 pm/g,虽然其谐振频率高,测量带宽大,但是灵敏度较低,不适合测量微弱的振动信号。
综上所述,目前的悬臂梁式传感器很难同时满足测量带宽大、灵敏度高的需求,因此本文基于F型梁结构设计了一种光纤光栅加速度传感器,通过将光纤光栅远离悬臂梁中性层达到增敏的效果,并将光纤光栅悬空固定,从而有效避免啁啾效应,最后对直接封装和填充硅油后封装的传感器进行性能分析和对比。
1 理论分析
1.1 传感器工作原理
光纤光栅加速度传感器由一体化加工的F型梁和质量块以及一根带有光纤光栅的小段光纤组成,如
1.2 传感器理论分析
惯性式加速度传感器的力学模型可以简化为一个单自由度系统,主要包括质量块、弹簧和阻尼器,如
上述惯性式加速度传感器的幅频特性为
式中,
根据

图 3. 惯性传感器振动系统幅频响应曲线
Fig. 3. Amplitude frequency response curve of inertial sensor vibration system
由
提出的加速度传感器的力学模型如
当传感器随被测物体一起振动时,假设振动加速度为
式中,
式中,L为梁长,E为梁的弹性模量,I为梁的惯性矩,可以表示为
式中,b为梁的宽度,h为梁的厚度。则第二横梁的等效刚度为
因此传感器谐振频率为
如
假设光纤光栅左端距离悬臂梁尾端的水平距离为
因此距离悬臂梁尾端
所以光纤光栅的应变为
光纤光栅的中心波长的变化量
式中,
所以传感器灵敏度为
2 参数优化及有限元分析
2.1 传感器参数优化
传感器的谐振频率和灵敏度决定了其频带范围与分辨率,但二者相互制约,而且谐振频率f与灵敏度S并非完全成反比,因此需要设计出最优参数,来得到合适的谐振频率与灵敏度。由
初步设定传感器的谐振频率为170 Hz,将灵敏度公式设为目标函数,约束条件为传感器各尺寸参数和谐振频率公式,使用序列二次规划方法进行求解[23]。考虑到传感器的尺寸及加工问题,传感器的优化模型设置为
式中,
表 1. FBG加速度传感器参数
Table 1. Parameters of FBG acceleration sensor
|
将
2.2 传感器有限元仿真
为了进一步研究传感器性能,利用ANSYS软件对传感器进行模态分析与谐响应分析。首先通过SOLIDWORKS建立传感器的三维模型,然后将三维模型导入到ANSYS中进行模态分析,得到该传感器的一阶模态振型图和三阶模态振型图,如
由
完成模态分析后,进一步对传感器进行谐响应分析。扫频范围设置为0~300 Hz,步长为5 Hz,施加方向为y方向、大小为5 m/s2的加速度载荷,设置阻尼比为0.02,模拟直接封装的传感器,选择模态叠加法进行谐响应分析。修改阻尼比为0.7,模拟填充硅油后的传感器,其他仿真条件不变,进行谐响应分析。传感器振幅最大处的模拟幅频响应曲线如
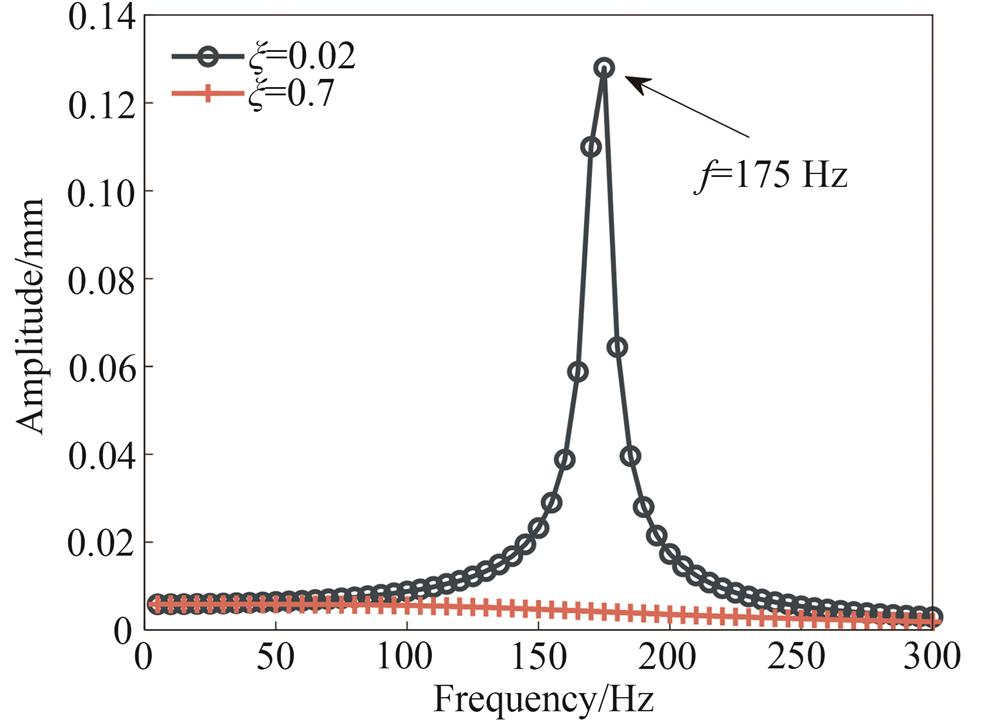
图 7. 传感器振幅最大处的模拟幅频响应曲线
Fig. 7. Simulated amplitude-frequency response curve at the maximum amplitude position of the sensor
由谐响应分析结果可知:传感器谐振频率在170~175 Hz之间,与一阶模态振型得到的谐振频率一致;
3 实验结果及分析
本文实验验证的传感器有两种类型,按照
实验测试系统包括激振系统和FBG传感器信号采集系统。激振系统采用丹麦B&K公司的振动平台作为振动源,由功率放大器、信号发生器和控制终端控制振动台的输入信号,激振系统中的标准压电式加速度传感器作为参考传感器。传感器信号采集系统包括FBG加速度传感器、光纤光栅解调仪与电脑,FBG加速度传感器固定在振动台上,传感器输出的信号经光纤光栅解调仪解调之后传至电脑,实验测试原理如
3.1 幅频响应测试
固定振动信号幅值为5 m/s2,进行扫频测试,扫频范围是10~240 Hz,步长为10 Hz,进行三次重复实验,取平均值,当接近谐振频率时,适当缩小步长,得到传感器1幅频响应实验结果。将传感器1更换为传感器2,重复上述实验步骤,得到传感器2的幅频响应实验结果。结果如
由实验结果可知:传感器1的幅频响应曲线与模拟幅频响应曲线基本一致,谐振频率为168 Hz,与理论值偏差为0.93%,且幅频响应曲线在0~50 Hz范围内较为平坦,与仿真结果有一定差距,这可能是因为传感器在制作时存在误差;传感器2的幅频响应曲线在0~100 Hz范围内较为平坦,与
为了进一步获得两个传感器所能测得的最低频率,在0~2 Hz内,设置步长为0.1 Hz,不断加大激振器频率,通过实验发现,传感器1与传感器2所能测得的最低频率均为1.5 Hz,因此传感器1和传感器2的工作频率分别为1.5~50 Hz、1.5~100 Hz。
由于振动台的振动加速度也受振动频率的影响,因此按照
表 2. 激振条件设置
Table 2. Excitation condition setting
|
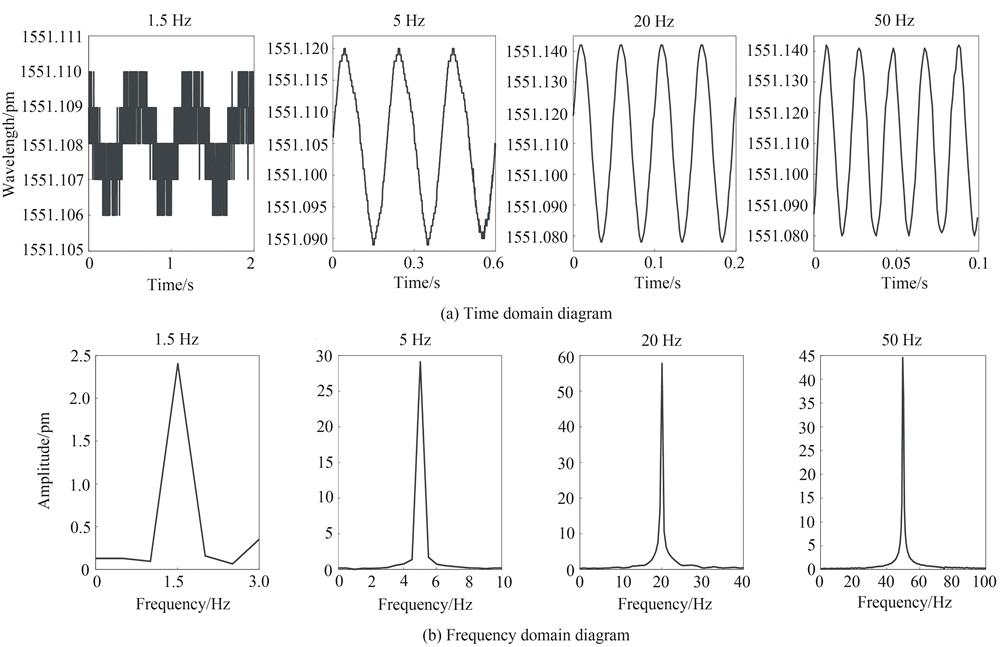
图 10. 不同激励条件下传感器1的波长时域图和频谱图
Fig. 10. Time domain diagram and frequency domain diagram of sensor 1 under different excitation conditions
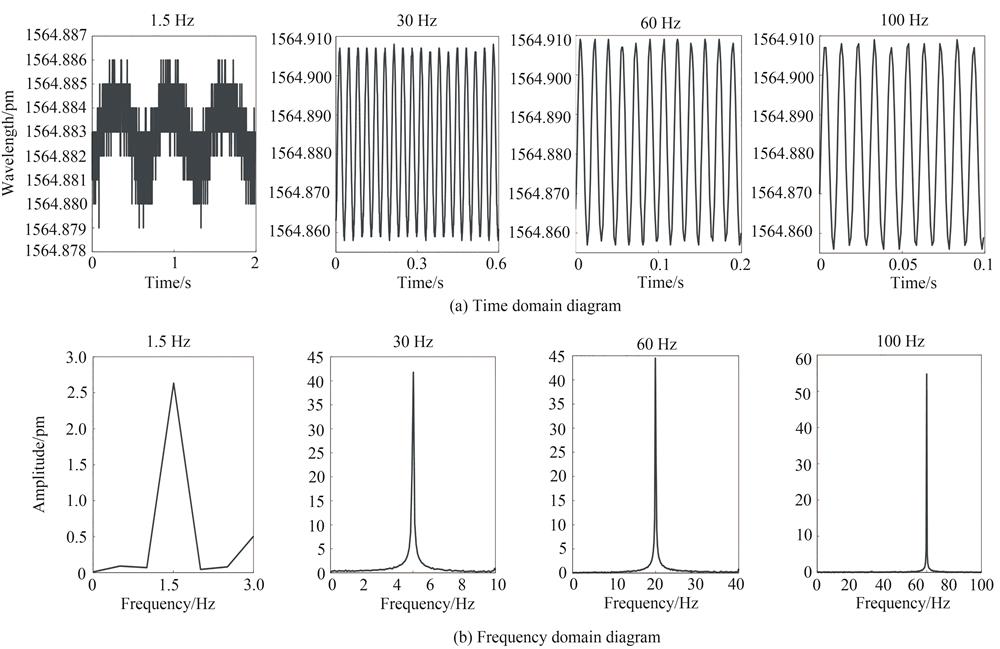
图 11. 不同激励条件下传感器2的波长时域图和频谱图
Fig. 11. Time domain diagram and frequency domain diagram of sensor 2 under different excitation conditions
由
3.2 灵敏度测试
调整激振器频率分别为20、30、50 Hz,加速度变化范围为2~10 m/s2,进行三组实验,每组实验取三次重复实验数据的平均值。得到传感器1的灵敏度实验结果,如
由实验结果可知传感器1在20 Hz、30 Hz、50 Hz时的灵敏度分别为159.35 pm/g、155.82 pm/g、164.44 pm/g,取灵敏度平均值159.87 pm/g作为传感器1的实际灵敏度,其与理论值偏差为3.29%,这可能是由于传感器机械加工误差引起的。传感器2在30 Hz、50 Hz、70 Hz时的灵敏度分别为133.77 pm/g、133.28 pm/g、133.57 pm/g,取灵敏度平均值133.54 pm/g作为传感器2的实际灵敏度,其比传感器1的灵敏度低26.33 pm/g,这可能是因为传感器1与传感器2内部的拾振结构无法做到完全一致,同时传感器2内部填充的硅油有一定阻力,会降低传感器2灵敏度,但传感器2在各频率下的灵敏度更接近,这也表示使用传感器2带来的误差会比使用传感器1带来的误差小。
3.3 横向抗干扰测试
固定振动信号幅值为5 m/s2,频率为40 Hz,分别测量两个传感器在主轴方向与非主轴方向(与主轴方向垂直)的波长漂移量,得到二者的横向抗干扰实验结果,如
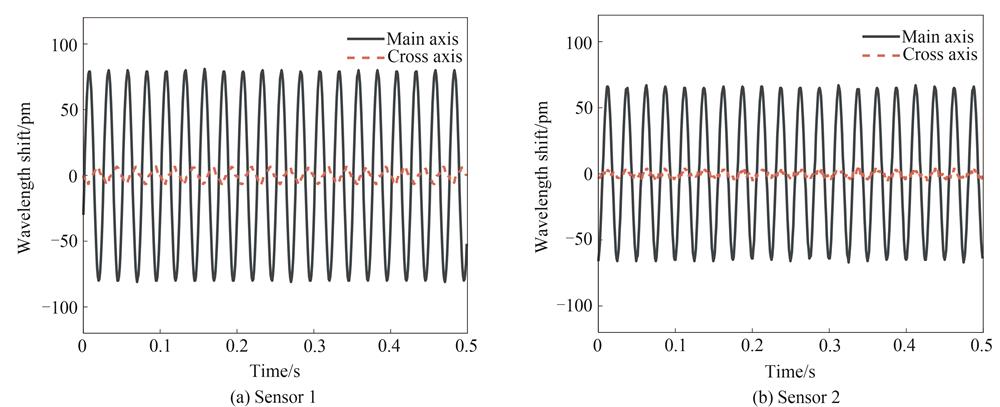
图 13. 传感器1和传感器2横向抗干扰测试曲线
Fig. 13. Curve of cross-axis anti-interference characteristics of sensor 1 and sensor 2
由
4 结论
本文设计了一种基于F型梁增敏结构的光纤光栅加速度传感器,对直接封装的传感器1与填充硅油的传感器2进行了仿真模拟与实验分析。实验结果表明传感器1与传感器2的幅频响应曲线与模拟谐响应曲线基本一致,传感器1的谐振频率约为168 Hz,测量带宽为1.5~50 Hz,灵敏度系数为159.87 pm/g;传感器2的测量带宽为1.5~100 Hz,灵敏度系数为133.54 pm/g。实验证明通过填充硅油可以使得传感器的幅频响应曲线更加平缓,扩大传感器的测量带宽,提高了传感器的横向抗干扰能力,这种方法虽然降低了传感器的灵敏度系数,但传感器在各频率下的灵敏度更加稳定,有效降低了传感器的使用误差。
[1] 周萌. 大量程高灵敏度的光纤Bragg光栅低频振动传感器研究[D]. 长春: 吉林大学, 2020.
ZHOUMeng. Research on large range and high sensitivity fiber Bragg grating low frequency vibration sensor[D]. Changchun: Jilin University, 2020.
[2] 周浩强. 光纤光栅振动加速度传感器的优化设计及振动体的振动模态分析[D]. 西安: 西安石油大学, 2013.
ZHOUHaoqiang. Fiber grating vibration acceleration sensor optimization design and the model analysis for the body the vibration[D]. Xi'an: Xi'an Shiyou University, 2013.
[3] 杨军, 周明, 邹洪刚, 等. 大型水轮发电机端部振动在线监测[J]. 设备管理与维修, 2021, 42(7): 156-157.
YANG Jun, ZHOU Ming, ZOU Honggang, et al. On-line monitoring of end vibration of large hydro generators[J]. Equipment Management and Maintenance, 2021, 42(7): 156-157.
[4] WANG X, GUO Y, XIONG L, et al. High frequency optical fiber Bragg grating accelerometer[J]. IEEE Sensors Journal, 2018, 18(12): 4954-4960.
[5] LIANGQ, SUNQ, XUJ, et al. A new fiber optic accelerometer based on fiber Bragg grating[C]. SPIE, 2012, 8332: 95-102.
[6] 于洋, 孟洲, 罗洪. 对称推挽式光纤光栅振动传感器设计研究[J]. 半导体光电, 2011, 32(1): 118-122.
[7] ZHANG Y S, QIAO X G, LIU Q P, et al. Study on a fiber Bragg grating accelerometer based on compliant cylinder[J]. Optical Fiber Technology, 2015, 26: 229-233.
[8] FAN W, WEI J, GAO H, et al. Low-frequency fiber bragg grating accelerometer based on diaphragm-type cantilever[J]. Optical Fiber Technology, 2022, 70: 102888.
[9] LIU Q, QIAO X, ZHAO J, et al. Novel fiber Bragg grating accelerometer based on diaphragm[J]. IEEE Sensors Journal, 2012, 12(10): 3000-3004.
[10] LIU Q, QIAO X, JIA Z, et al. Large frequency range and high sensitivity fiber Bragg grating accelerometer based on double diaphragms[J]. IEEE Sensors Journal, 2014, 14(5): 1499-1504.
[11] 梁磊, 朱振华, 李美格, 等. 基于双光纤光栅的柔性铰链加速度传感器设计[J]. 仪表技术与传感器, 2020(11): 1-4, 9.
LIANG Lei, ZHU Zhenhua, LI Meige, et al. Design of flexible hinge acceleration sensor based on double fiber Bragg grating[J]. Instrument Technique and Sensor, 2020(11): 1-4, 9.
[12] 刘文敏, 戴玉堂, 魏禹. 基于双光纤光栅的高灵敏度低频加速度传感器[J]. 光电子 · 激光, 2021, 32(9): 911-918.
LIU Wenmin, DAI Yutang, WEI Yu. High sensitivity and low-frequency acceleration sensor based on double fiber Bragg gratings[J]. Journal of Optoelectronics · Laser, 2021, 32(9): 911-918.
[13] 王赟, 戴玉堂, 刘文敏, 等. 基于柔性铰链的光纤光栅二维加速度传感器的优化设计[J]. 光子学报, 2019, 48(8): 0806003.
[14] 张法业, 姜明顺, 隋青美, 等. 基于柔性铰链结构的高灵敏度低频光纤光栅加速度传感器[J]. 红外与激光工程, 2017, 46(3): 260-267.
ZHANG Faye, JIANG Mingshun, SUI Qingmei, et al. High sensitivity and low-frequency FBG acceleration sensorsbased on flexure hinge structure[J]. Infrared and Laser Engineering, 2017, 46(3): 260-267.
[15] YAN B, LIANG L. A novel fiber Bragg grating accelerometer based on parallel double flexible hinges[J]. IEEE Sensors Journal, 2020, 20(9): 4713-4718.
[16] 王宏亮, 周浩强, 高宏, 等. 基于双等强度悬臂梁的光纤光栅加速度振动传感器[J]. 光电子 · 激光, 2013, 24(4): 635-641.
WANG Hongliang, ZHOU Haoqiang, GAO Hong, et al. Fiber grating acceleration vibration sensor with double uniformstrength cantilever beams[J]. Journal of Optoelectronics · Laser, 2013, 24(4): 635-641.
[17] KHAN M, PANWAR N, DHAWAN R. Modified cantilever beam shaped FBG based accelerometer with self temperature compensation[J]. Sensors and Actuators A: Physical, 2014, 205(10): 79-85.
[18] BASUMALLICK N, CHATTERJEE I, BISWAS P, et al. Fiber Bragg grating accelerometer with enhanced sensitivity[J]. Sensors and Actuators A: Physical, 2012, 173(1): 108-115.
[19] 曾宇杰. 光纤布拉格光栅低频加速度传感器技术研究[D]. 长沙: 国防科学技术大学, 2015.
ZENGYujie. Research on FBG accelerometer with low frequency[D]. Changsha: National University of Defense Technology, 2015.
[20] 魏莉, 刘壮, 李恒春, 等. 基于“士”字形梁增敏结构的光纤光栅振动传感器[J]. 光学学报, 2019, 39(11): 1106004.
[21] 贾振安, 张星, 李康, 等. 一种悬臂梁式光纤光栅振动传感器研究[J]. 压电与声光, 2019, 41(1): 21-24.
JIA Zhen'an, ZHANG Xing, LI Kang, et al. Study on a cantilever beam FBG vibration sensor[J]. Piezoelectrics and Acoustooptics, 2019, 41(1): 21-24.
[22] 张绪成, 孙志慧, 杨元元, 等. 基于L型悬臂梁的光纤光栅加速度传感器[J]. 光电子·激光, 2021, 32(7): 696-702.
ZHANG Xucheng, SUN Zhihui, YANG Yuanyuan, et al. Fiber Bragg grating accelerometer based on L-shaped cantileverbeam[J]. Journal of Optoelectronics · Laser, 2021, 32(7): 696-702.
[23] 唐冲. 基于Matlab的非线性规划问题的求解[J]. 计算机与数字工程, 2013, 41(7): 1100-1102, 1185.
TANG Chong. Nonlinear programming problem solution based on Matlab[J]. Computer and Digital Engineering, 2013, 41(7): 1100-1102, 1185.
Article Outline
甘维兵, 张艺璇, 张寅杰, 王宇, 刘念, 张翠. 基于F型梁的光纤光栅加速度传感器[J]. 光子学报, 2023, 52(6): 0606001. Weibing GAN, Yixuan ZHANG, Yinjie ZHANG, Yu WANG, Nian LIU, Cui ZHANG. Fiber Bragg Grating Accelerometer Based on F-beam[J]. ACTA PHOTONICA SINICA, 2023, 52(6): 0606001.