All-fiber laser amplification at 1948 nm based on double-cladding Tm/Al co-doped photonic crystal fiber fabricated by laser additive manufacturing
1. Introduction
Rare-earth elements as doped materials in lasers have been developed for years, of which thulium (Tm)-doped lasers, emitting light in the mid-infrared 2.0 µm band, have become a hot spot in current research due to their unique advantages in ophthalmic medical treatment[1], atmospheric communication[2], and the military field[3]. A photonic crystal fiber (PCF) provides the characteristics of endless single mode, adjustable dispersion, and high nonlinearity. The main advantage of PCF in the application of fiber amplifiers and fiber lasers is that it can flexibly design the fine performance of double-cladding PCF[4]. Through the air hole, the outer cladding index of double-cladding PCF can approach one, thereby adding the NA of inner and outer cladding to improve the coupling efficiency of the pump laser. It also has the advantage of ensuring the signal light works with the signal mode at a large core diameter, and it not only separates the core fiber heart from the high-power laser but also keeps good beam quality. Meanwhile, a high doping concentration is required for achieving 200% quantum efficiency of cross-relaxation (CR)[5]. The flexible design advantages of PCFs can be well used in Tm-doped fibers[6]. In the ultrafast optical region, Gaida et al. achieved a pulse peak power of more than 4 MW at sub-700 fs pulse duration by Tm-doped PCF[7]. In 2020, Lee et al. attained a linearly-polarized amplifier with 1.5 ns, 100 kHz pulse train, and up to 250 µJ pulse energy[8]. In addition to these, many new reports about ultrafast optics will probably be optimized using PCF in the future[9–13]. In the CW laser region, Jansen et al. fabricated the large-area mode Tm-doped PCF and applied it to the laser. It finally got more than 52 W of output and 32.5% slope efficiency[4]. In 2013, Gaida et al. applied Tm-doped PCF rods and achieved 4 W seed output power at 1960 nm; the slope efficiency was 20.1%[14]. In 2016, Molardi et al. designed the Tm-doped PCF with a core diameter of and a hole size to pitch ratio of 0.2. The finite-element method simulated the single-mode operation and gained a ratio value of 0.54 between the fundamental mode and the high-order mode[15]. In 2022, Kang et al. prepared Tm/Ho co-doped lanthanum aluminosilicate PCF and presented strong broadband luminescence characteristics[16]. Furthermore, various fabrication methods have been applied to get a large mode area and high-doping core materials[17–20]. In 2019, Chen et al. fabricated the Tm/Al co-doped PCF by the laser sintering technique to get 740 mW output power at 1.95 µm and a slope efficiency of 16.73%[21]. In 2020, Liu et al. used laser additive manufacturing technology to produce Tm/Al co-doped 2 µm PCF and obtained a slope efficiency of 13.9%[22]. However, space structure was generally applied in the above research work due to the splicing difficulty of PCF, which is not conducive to the integration of fiber lasers. The doping homogeneity and concentration also need to be improved for the promising application of Tm-doped PCFs.
In this work, we fabricated a heavily Tm/Al co-doped PCF by laser additive manufacturing, and the laser properties in an all-fiber experiment were conducted. The splicing loss between the PCF and double-cladding passive fiber was discussed. The upconversion process of Tm-doped PCF under 1064 nm pulse laser excitation was also performed. This work helps to promote the applications of Tm-doped PCF around eye-safe as well as visible wavelength ranges.
2. Experiment
The Tm-doped PCF was fabricated by our self-developed laser additive manufacturing[22] and drawn to fiber through a stack-and-draw technique. Figure 1 shows the detail of the laser additive manufacturing process, i.e., the silica rod is fused using output energy from the CO2 laser, then Tm is added to obtain the Tm-doped rod, and the PCF is drawn after polishing.
The fiber profile and doping concentration were measured by electron probe microanalysis (EPMA) and are shown in Fig. 2. The double-cladding Tm/Al co-doped PCF has a good microstructure periodic arrangement. The diameters of the fiber core, inner cladding, outer cladding, and bare fiber were measured at 40, 200, 250, and 400 µm, respectively. The hole size to pitch ratio (d/Λ) was 0.1 for inner cladding, corresponding to a 13 µm pitch value. The concentrations of the Tm element in the PCF core in Fig. 2(b) have relatively good uniformity and no obvious cluster. The measured doping concentrations of and were 2.13% and 1.77% (mass fractions), respectively. Figure 2(c) is the result of the line scanning of PCF. Tm and Al are roughly evenly distributed in the fiber core under their respective orders of magnitude.
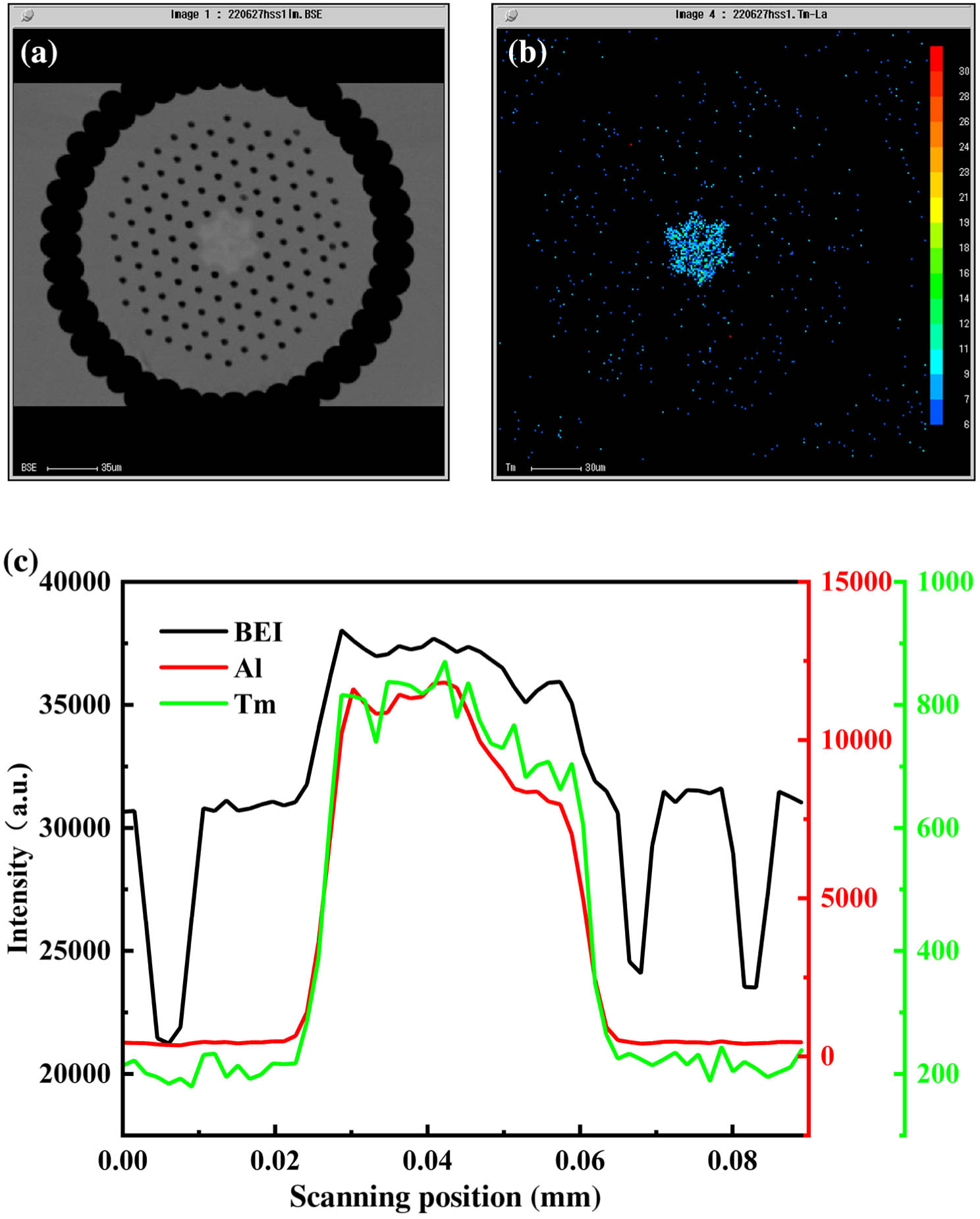
Fig. 2. (a) Electron microscope image of Tm/Al co-doped silica fiber; (b) EPMA mapping of the Tm elements in the core; (c) linear analysis of the PCF core.
A master-oscillator-power-amplifier (MOPA) system was established to measure the fiber laser properties, as shown in Fig. 3. The seed source was a 1948 nm self-built laser that can provide 4 W output power, and the 793 nm remaining pump light was removed through a cladding power stripper (CPS). A combiner was used to couple the seed source and 793 nm pump power through the signal and pump arm, respectively. Afterward, the double-cladding Tm/Al co-doped PCF was spliced to the combiner pigtail by LZM-100 to amplify the seed source power. At the end of the PCF, the laser beam was collimated by a lens. Then, the residual 793 nm pump light was filtered by a long-pass filter behind the lens. Finally, the spectrum and the power level were measured by an optical spectrum analyzer (OSA) and optical power meter, respectively.
3. Analysis of Results
In the PCF cutting process, the wavefront of the oscillating wave forms a circle, with the knife edge as the center of the circle[23]. Since the air holes are tightly arranged rings that prevent the propagation of oscillating waves, a suitable tension force choice is important. The uneven end face of the fiber cut is shown in Fig. 4(a). The fiber will not break under weak tension force, while too much tension force will cause a time difference when the inner and outer claddings of the fiber break, resulting in a different tension force and an uneven end face. For our fabricated Tm-doped PCF, the cutting parameters were optimized to be 470 gN of cut tension and 2700 gN of left and right clamping force. A smooth fiber end was obtained, as shown in Fig. 4(b), facilitating subsequent splicing operations.
A laser (Fujikura LZM-100) was utilized to splice the double-cladding Tm/Al co-doped PCF with the combiner output pigtail[24], which has a visible advantage in dealing with air-hole collapse. When the heating temperature of the fiber end is higher than its softening temperature, the surface tension of the material will exceed the viscosity, causing the pores to collapse rapidly during the splicing process. Figure 5(a) shows the air hole force images when the PCF is heated. The point P on the outside of the hole was mainly forced by the surface tension , the gravity , and the pressure formed by the difference in pressure inside and outside the hole. During the splicing process, the PCF air hole communicates with the atmosphere, and can be regarded as zero. During force analysis, only gravity and surface tension are considered. The force analysis of the P point is shown in Eq. (1),

Fig. 5. (a) PCF air-hole force map; (b) the picture of collapsed air hole; (c) acceptability results of splice PCF with passive fiber.
The deformation distances of the P point at the axis and axis can be expressed by and ,
According to the start condition, , , and , the deformation distance of the air hole is as follows:
From Eqs. (6) and (7), we can see that is insensitive to temperature, and will decline when the temperature increases, resulting in the air-hole collapse. The validation of collapse is shown by Eq. (8),
Equation (8) shows that was related to ; the higher the temperature, the faster drops, and the faster the air holes collapse. The PCF air hole has collapsed under the high temperature in Fig. 5(b). For reliable splicing quality, the final cut end face angle should be less than 1°. To avoid the collapse of the PCF air hole caused by heating under high temperature, the power of the laser is controlled to be 18 W and the discharge duration is 1200 ms, which can minimize the air-hole collapse and make the splice effect reach the ideal degree. If the core diameter is not equal, the transverse tension will make the splice point fracture, but it can withstand longitudinal tensile. The quality of the weld is independent of its ability to withstand lateral tensile forces.
The PCF with combiner pigtails has a splice loss of 0.23 dB. Losses due to mode-field mismatch are also introduced when the PCF and the pigtail are concatenated under the condition that the core diameters are perfectly aligned; that is, intrinsic losses are neglected, and the PCF air holes do not collapse[25].
Figure 6 is the near-Gaussian shape beam profile of the PCF fiber amplifier, where a slightly irregular beam was observed due to multiple transverse modes. Another reason may be the mode mismatch: the seed source splice with PCF has a light leakage absorbed by cladding. The splice quality is an important factor in the amplifier system, and it will be improved in our next work.
Figure 7(a) shows the laser spectra measured by MAYA2000 Pro (200 to 1100 nm) and NIR Quest 256 (900 to 2500 nm). The spectral intensity at 1948 nm was enhanced with the pump power increasing. Figure 7(b) is the MOPA system amplifier slope efficiency. At 0.56 W seed input power, about 1 W of power amplification was achieved. Finally, 1.59 W laser output power at 1948 nm was obtained, corresponding to 13% of slope efficiency. The output laser power was linearly enhanced, and further increase was limited by the available pump power. The low slope efficiency might be attributed to the doping fluctuation, and higher doping concentration is expected to help improve efficiency.

Fig. 7. (a) Spectra of seed source and pump source at different powers; (b) slope efficiency of double-cladding Tm/Al co-doped PCF amplifier.
Figure 8 shows the upconversion emission spectra of the double-cladding Tm/Al co-doped PCF under different power of 1064 nm fiber laser excitation. The pump source has an average power of 4 W, corresponding to 160 ps pulse duration, 1 MHz repetition frequency, and 25 kW peak power. Emission bands centered at 360, 456, 660, and 800 nm were observed, which was attributed to transitions of (353 nm), (450 nm), (620 nm), and (792 nm), respectively. As the pump intensity increases to a high excitation intensity, such as , four emission bands take place almost simultaneously. In our prepared glass, the 353 nm upconversion process is stronger than the other three bands, which might be caused by the intrinsic bi-stability characteristics of the level population concentration of the system[26]. This indicates the potential application in the ultraviolet region.
4. Conclusion
In this work, we present a double-cladding Tm/Al co-doped PCF fabricated by laser additive manufacturing and its all-fiber laser amplifier. A smooth cut end and low splicing loss (0.23 dB) of our Tm-doped PCF were achieved, providing an experimental basis for power amplification. Finally, a slope efficiency of 13% with 1.59 W laser power was obtained. In addition, the upconversion emission indicates the possibility of applying the double-cladding Tm/Al co-doped PCF to short-wavelength applications. Applying the all-fiber structure of Tm-doped PCF lays the foundation for the all-fiber application and integration of PCFs. The above results indicate that the laser amplification potential of the double-cladding Tm/Al co-doped PCF at 1948 nm is yet to be developed and that it also has great advantages in the short-wavelength direction, which will be greatly exploited in the future in the field of fiber lasers.
[7] C. Gaida, F. Stutzki, M. Gebhardt, F. Jansen, A. Wienke, U. D. Zeitner, F. Fuchs, C. Jauregui, D. Wandt, D. Kracht, J. Limpert, A. Tünnermann. Sub-700 fs pulses at 152 W average power from a Tm-doped fiber CPA system. Proc. SPIE, 2015, 9344: 93441K.
[15] C. Molardi, E. Coscelli, A. Cucinotta, S. Selleri. Thermo-optical effects in Tm-doped large mode area photonic crystal fibers. Proc. SPIE, 2014, 8961: 89612Q.
[26] L. Li, Q. Gong, X. Zhang, L. Chen, Y.-R. Shen. Bistable chromatic switching in 648 nm laser pumped Tm-doped crystal. Proc. SPIE, 2007, 6839: 68390R.
Yongwei Shi, Nan Zhao, Jiantao Liu, Jiaming Li, Zhiyun Hou, Guiyao Zhou. All-fiber laser amplification at 1948 nm based on double-cladding Tm/Al co-doped photonic crystal fiber fabricated by laser additive manufacturing[J]. Chinese Optics Letters, 2023, 21(12): 121401.