Optimization of optical wireless power transfer using near-infrared laser diodes
Download: 665次
Wireless power transfer (WPT) is a technology where the energy is transmitted from a power source to a target device without any physical connections[1]. Because smart phones are connected to a wire only when they need to be charged, WPT may be the final technology that allows mobile devices to be completely free from the need to be wired[2]. Owing to its convenience and flexibility, WPT has gained much interest recently and its market is growing rapidly although the transfer efficiency of WPT is lower than that of conventional wired power transfer. The current WPT technologies can be broadly classified into nonradiative coupling-based transfer and radiative radio frequency (RF)-based transfer technologies[1]. The former includes inductive coupling[3], magnetic resonance coupling[4], and capacitive coupling[5], whereas the latter includes directive RF power beamforming[6] and nondirective RF power transfer[7], as illustrated in Fig.
The transfer efficiencies of nonradiative coupling-based transfer technologies are relatively high, and therefore they have been widely commercialized. Wireless smartphone chargers are among the applications of nonradiative coupling-based transfer technologies. However, the effective transfer distances of these technologies are less than several meters. Although radiative RF-based transfer technologies can transfer power over longer distances, the transfer efficiency of these technologies decreases in proportion to the square of the distance[8]. Although directive RF power beamforming technology has been proposed to increase the transfer distance more, the effective transfer distance is still limited. In addition, radiative RF-based technology causes electromagnetic interference (EMI) to other electronic devices.
For long-distance WPT, optical wireless power transfer (OWPT) can be a suitable solution[2]. OWPT is a WPT technology that uses light to deliver power[9]. In OWPT, a light source such as a laser is used at the transmitter site to convert electric power into optical power, and an optical power receiver such as a photovoltaic (PV) cell or a photodiode (PD) is used at the receiver site to convert the received optical power into electric power. If the power source is a form of optical power such as sunlight, then power conversion is not required at the transmitter site. Likewise, if the final usage form of power is optical power, such as illumination or a light-heated pot, then power conversion is not required at the receiver site.
OWPT has several advantages over radiative RF-based transfer technologies. First, OWPT can deliver large amounts of power to a distant small aperture because the laser beam size can be maintained even over long distances. Second, the effective transfer distance of OWPT is longer than that of radiative RF-based transfer technologies. The laser power does not decrease in proportion to the square of the distance, unlike radiative RF-based technologies. The laser power decreases as an exponential function. However, its attenuation coefficient is very low compared to the RF decrease. Third, OWPT does not cause EMI to the neighboring electronic devices. Fourth, an optical power receiver such as a PV cell can also be used to receive solar power. This means that the mobile device can receive power from both the intentional optical power source (i.e., laser) and the nonintentional optical power source (e.g., the sun or other illumination sources).
To date, several studies have been carried out on OWPT[10
In this Letter, we present an experimental investigation of the DC-to-DC transfer efficiency of OWPT using a near-infrared laser diode (LD) as the optical power transmitter. As for the optical power receiver, we consider two kinds of devices: (1) a PV cell and (2) a PD, whereas other researchers usually considered only the PV cell as the optical power receiver. We will compare the performances of the PV cell and the PD from the viewpoint of transfer efficiencies. Finally, we will demonstrate the optimization process of the OWPT system in order to maximize the DC-to-DC transfer efficiency using the given devices.
Figure
Figure
To maximize the E/O conversion efficiency of the LDs, an optimization process is required. To optimize the operation condition of LDs, we need to investigate the characteristics of the LDs. Figure

Fig. 4. E/O conversion efficiency and optical output power of (a) a 200 mW LD and (b) a 500 mW LD.
It can be observed that the point at which the E/O conversion efficiency was maximum was close to the starting point of the LD power saturation region. This tendency was also observed in a previous work[10]. In the linear LD power region, the E/O conversion efficiency increases with the increasing operating voltage. This is because when the operating voltage is lower than the voltage in the LD power saturation region, the stimulatively emitted photon can be absorbed again inside the gain medium because the gain medium is not fully excited. After the saturation region, the gain medium is fully excited so more electric energy (higher operating voltage) cannot make more photons. Therefore, the E/O conversion efficiency is maximum near the starting point of the LD power saturation region. The parameter values of the LDs at the maximum E/O conversion efficiency are summarized in Table
Table 1. Operating Condition of the LDs at the Maximum E/O Conversion Efficiency
|
Similar to the transmitter site, the optical power receiver also needs to be optimized in order to maximize the O/E conversion efficiency at the receiver site. In this Letter, we investigated and compared two kinds of optical power receiver devices: (1) a PV cell and (2) a PD. First, we measured the characteristics of the PV cell and PD as the optical power receivers when the 200 mW LD was used as the optical power transmitter. A silicon PV cell with a size of
Figures

Fig. 5. Voltage-current graphs of the (a) PV cell and (b) PD by changing the load resistance when the 200 mW LD is operating at the maximum E/O conversion condition.
When the PD was used as the optical power receiver, the obtained maximum electric power was 24.0 mW at a load resistance of
Then we analyzed the characteristics of the PV cell and PD as the optical power receivers when the 500 mW LD was used as the optical power transmitter. Figures
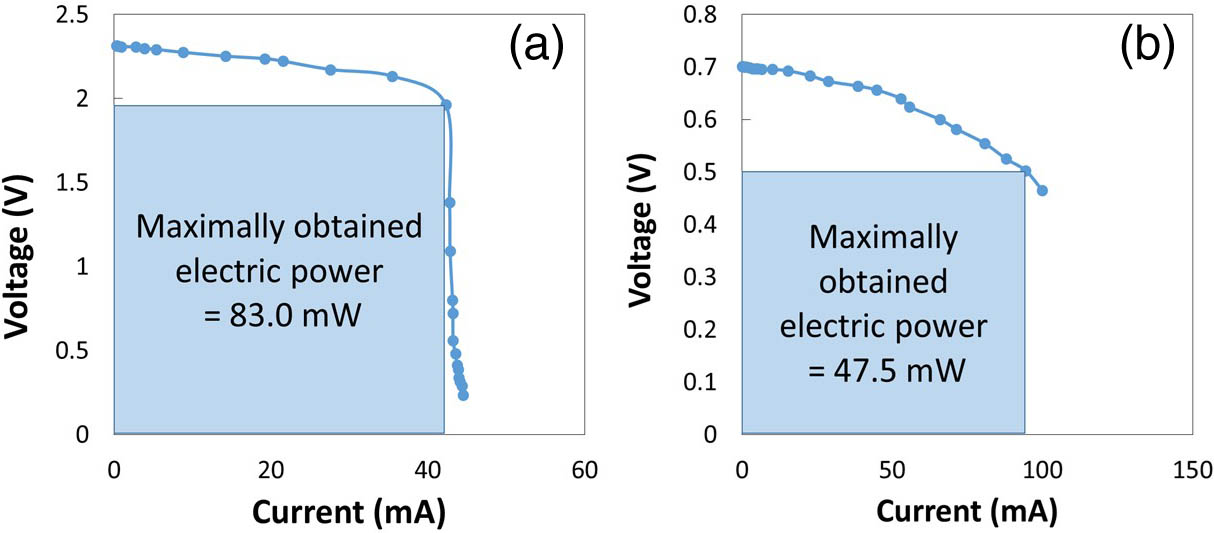
Fig. 6. Voltage-current graphs of the (a) PV cell and (b) PD by changing the load resistance when the 500 mW LD is operating at the maximum E/O conversion condition.
Based on the results presented in Table
The optimization process may look finalized. However, there are more chances for further optimization. The results in Table
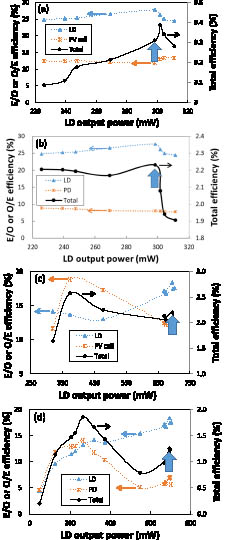
Fig. 7. E/O conversion efficiency of the LD, the O/E conversion efficiency of the optical power receiver, and the total OWPT efficiency as functions of the optical output power of the LD for the following OWPT systems: (a) 200 mW LD and PV cell, (b) 200 mW LD and PD, (c) 500 mW LD and PV cell, and (d) 500 mW LD and PD. The point marked by the arrow in each figure is the point where the E/O conversion efficiency of the LD is the maximum.
From Fig.
Table 2. OWPT Efficiency When the Optical Power Transmitter Was Operating at the Maximum E/O Conversion Efficiency
|
Table 3. Maximum OWPT Efficiency After the Whole System Optimization
|
As shown in Table
Here we remark that the OWPT efficiency can be further improved by using a more efficient LD, PD, and PV cell. In addition, alignment between the optical power transmitter and the optical power receiver and matching the receiver aperture to the LD beam shape are also important to improve the OWPT efficiency.
In summary, we have presented an OWPT system using near-infrared LDs as the optical power transmitters and have also presented an optimization process to maximize the transfer efficiency. We used a PV cell or a PD as the optical power receiver in the experiment. The maximum transfer efficiency was achieved by optimizing the load resistance of the optical power receiver while varying the operating condition of the LD. After the whole system optimization presented here, we found that the OWPT efficiencies improved from 3.32% to 3.39%, from 2.20% to 2.57%, and from 1.26% to 1.86% (increase by 48% from the original value), depending on the OWPT system cases. This optimization process is important to achieve a high transfer efficiency in OWPT.
[1]
[2]
[3]
[4]
[6]
[7]
[8]
[9]
[10]
[11]
[12]
[13]
[17]
[18]
Sung-Man Kim, Hanbit Park. Optimization of optical wireless power transfer using near-infrared laser diodes[J]. Chinese Optics Letters, 2020, 18(4): 042603.