Metalens for coaxial double wavelength focusing
Download: 730次
Tightly focused far-field spots have many applications in various fields such as laser precision processing, fluorescent microscopic imaging, lithography, optical data storage, and micro-particle manipulation. The spot size is usually measured with its full width at half-maximum (FWHM). However, the Abbe’s diffraction limit strongly restricts their minimum spot size. Fighting against the diffraction limit has always been the topic optical scientists make the effort to tackle. Mainly, there are two approaches to break through the diffraction limit. Conventionally, we usually obtain the far-field spot size below the diffraction limit by comprehensively modulating the amplitude, phase, and polarization distribution of the laser beam at the entrance pupil of the objective with a high numerical aperture. Because of its axial symmetry of polarization, the radially polarized beam is preferred to obtain a spot size smaller than the diffraction limit[1]. In 2003, Quabis’s group from Germany used the annular aperture to block the central part of the light beam in the focusing of radially polarized beams[2]. The central blocking of incoming light increased the ratio of the longitudinally polarized component to the transversely polarized component in the focal volume and made the size of the focusing spot smaller. In their work, the annular aperture acts as an amplitude filter. In 2008, Wang from the Singapore Data Storage Institute designed a multiple binary annular phase mask to modulate the incident radially polarized beam[3]. The FWHM of the focusing spot size is around
Another approach to break through Abbe’s diffraction limit is the so-called super-oscillatory lens. The super-oscillatory lens is first proposed theoretically, to the best of our knowledge, by Berry from the University of Bristol in 2006[4,5]. In 2012, Zheludev’s group from the University of Southampton designed and fabricated a super-oscillatory lens composed of a series of concentric binary amplitude rings[6,7]. They obtained a spot size of 185 nm
In the field of scanning fluorescent microscopic imaging, the resolution is directly determined by the obtainable minimum focusing spot size. To circumvent this limit, Hell invented stimulated emission depletion microscopy (STED)[9,10]. He introduced a second laser beam with a different wavelength. It is imposed on a vortex phase modulation and is focused into a doughnut-shaped spot that acts as depleting stimulated fluorescent molecules. It in turn greatly reduces the size of the single pixel emitting fluorescent light. The STED system usually requires careful coaxial tuning of two laser beams along with a separate vortex phase plate and a high numerical objective. The misalignment of the optical elements will deform and damage the circular symmetry of the doughnut-shaped spot. It seriously compromises the resolution of the STED system.
In recent years, metasurfaces have attracted great attention attributed to their distinguished features, versatile functionality, easy integration, and relatively simple fabrication. Metasurfaces are usually composed of an ultrathin, space-variant, and nanostructured subwavelength cell array acting as local polarization, amplitude, or phase modulation pixels[11
In this study, we propose and demonstrate a metalens for coaxial double wavelength focusing, which can be used in the STED scheme. Due to the nature of the method, it guarantees the coaxial feature, the optical stability, and the circular symmetry of the doughnut-shaped spot.
We assume that the first laser with wavelength
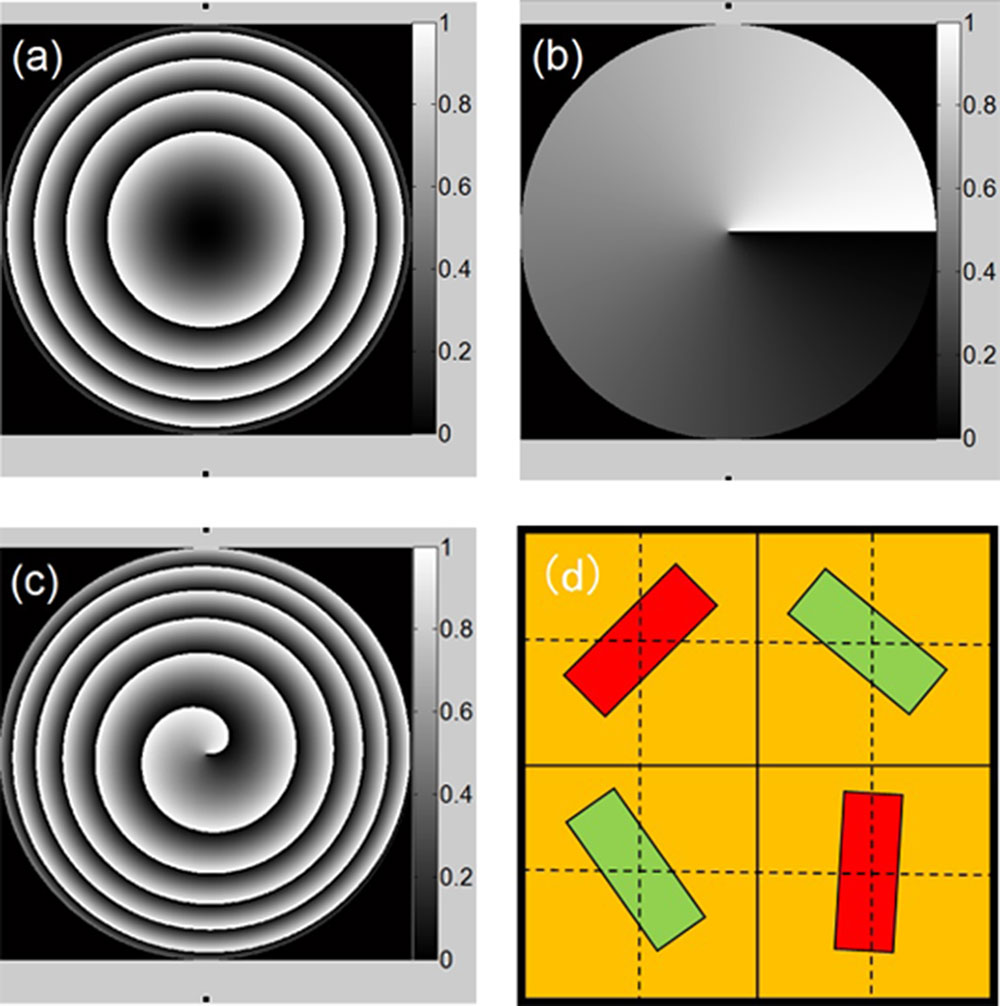
Fig. 1. Design scheme of the metalens for coaxial double wavelength focusing. (a) The phase modulation for the laser with a wavelength of ; (b) the phase modulation of a vortex with a topological charge of one; (c) the phase modulation combining (a) and (b) for the laser with a wavelength of ; (d) the meta-molecular structure unit.
The metalens is composed of a square cell array. Each square cell is further divided into four small squares, as shown in Fig.
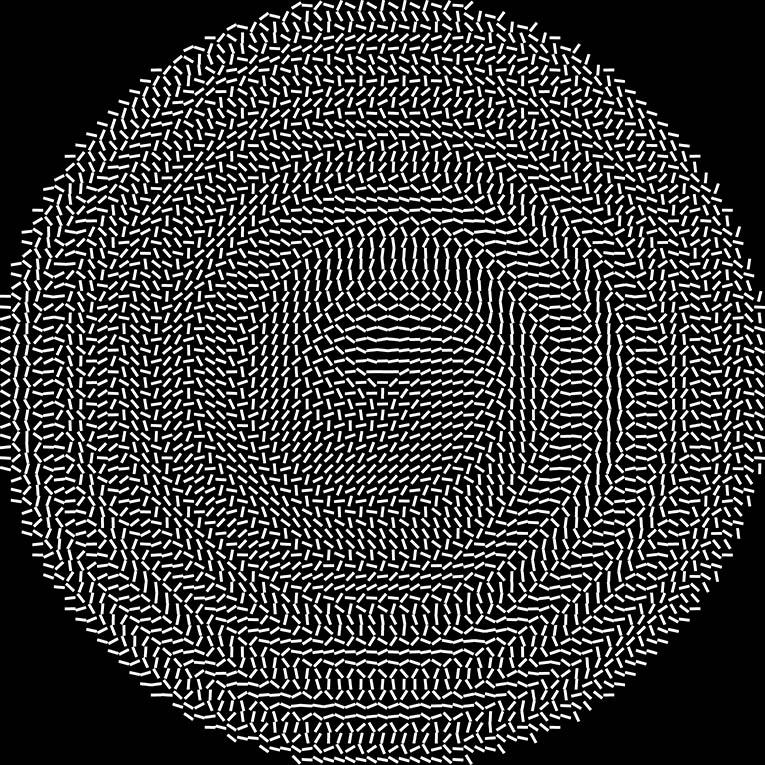
Fig. 2. Schematic diagram of the designed metalens for coaxial double wavelength focusing.
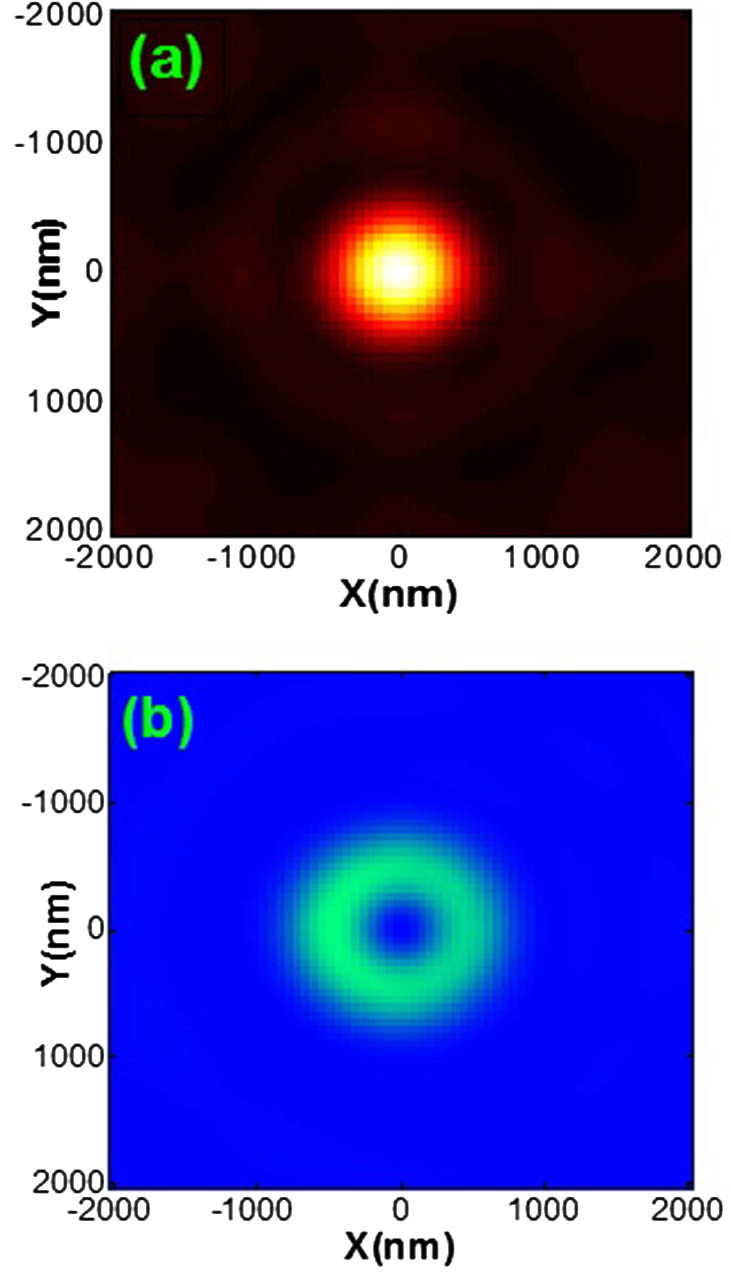
Fig. 3. FDTD-simulated focusing spot for designed metalens. (a) The circular solid spot for the first laser with ; (b) the doughnut-shaped focusing spot for second laser with .
To verify the design effectiveness of the metalens, we prepared a gold film on the substrate with the electron beam evaporation technique, and the thickness of the film is 150 nm. Then, the sample metalens is fabricated by a focused ion beam (FIB, EFI Nanolab600, Helios). The size of the experimental sample (
We have demonstrated the design scheme of a metalens for coaxial double wavelength focusing. Both the numerical simulation of the FDTD solution and experimental verification show that the design scheme is reasonable. The advantage of the metalens lies in that the vortex phase plate and conventional objective are combined into one integrated metalens. This insures the coaxial demand of two spots and the stability of the STED system. Another advantage of the metalens compared with the conventional objective made of glass and metal is that it has a very small size and weight. The small size may help to realize the portability and instrument minimization. The small weight is beneficial to the easy and precise scanning of the relevant STED system.
[1]
[2]
[3]
[4]
[5]
[6]
[7]
[8]
[9]
[10]
[11]
[12]
[13]
[15]
[16]
[17]
[18]
[19]
[20]
[21]
[22]
[23]
[24]
[25]
[26]
Jiannong Chen, Baodong Wang, Linwei Zhu, Jing Han, Qinfeng Xu. Metalens for coaxial double wavelength focusing[J]. Chinese Optics Letters, 2020, 18(4): 042401.