基于双光子聚合3D打印的光纤法珀微波导腔制备及传感特性研究
Optical fiber Fabry-Perot microcavity sensor has the advantages of small size and good stability, and it is widely used in the measurement of temperature, magnetic field, refractive index, and other physical quantities. However, the traditional processing method has many drawbacks and limitations. For example, the fusion method of single-mode fibers and special fibers may cause the fusion area to collapse and form a conical structure, resulting in poor performance of the prepared sensor; the operation of the hollow-core fiber filling method is difficult, and the preparation repeatability is poor; the chemical reagents of chemical corrosion method are easy to cause harm to the human body. The femtosecond laser two-photon polymerization 3D printing technology adopted in this paper has the advantages of high processing precision, strong flexibility, and high repeatability and can cope with more complex conditions to achieve structure preparation. In addition, the optical fiber Fabry-Perot microcavity sensor is widely used in refractive index sensing, but due to the introduction of a section of air optical path, its light conduction ability is relatively insufficient, and the insertion loss is large. In this paper, the optical micro-waveguide is introduced to form the integrated sensing and guiding optical fiber Fabry-Perot micro-waveguide cavity. The Fabry-Pert microcavity can limit the light field to the micron range and support and protect the micro-waveguide structure. Meanwhile, micro-waveguides not only ensure good optical conductivity of the structure but also further enhance the refractive index sensitivity of the overall structure based on their strong evanescent field characteristics.
The micro-waveguide Fabry-Perot cavity structure is designed by COMSOL and simulated by finite difference time domain method and finite element method. The simulation results show that the refractive index sensitivity of the proposed structure can reach 555.14 nm/RIU in the range of 1.333-1.337 (Fig. 3). The structure is prepared by femtosecond laser two-photon polymerization 3D printing after setting the printing parameters. First, the fiber coating layer is removed; the flat single-mode fiber core end face with a cutter is cut out, and it is cleaned with a welding machine. Second, the optical fiber is fixed to the printer position, and the lens is focused on the optical fiber end face. Third, the optical fiber end face is adjusted to the center position of the printing equipment for printing. Fourth, the structure is cured using propylene glycol methyl ether acetate solution and isopropyl alcohol solution (Fig. 4).
The reflection spectrum of the printed structure in water is observed by the optical spectrum analyzer. It can be seen that the free spectrum range (FSR) of the structure formed in deionized water is 16.1 nm. The spectral Fourier transform results show that the structure forms a single peak with a good interference effect in the low-frequency band (Fig. 5). It is also confirmed that the micro-waveguide structure can play a good anti-interference role in the optical fiber structure and shield the external disturbance to a certain extent. The structures are placed in 12 sodium chloride solutions with different concentrations in the refractive index range of 1.3346-1.3764 for experiments, and the linear fitting results of the reflection spectra show that the sensing structure has good linearity and sensitivity of 525.81 nm/RIU in the refractive index range of 1.3346-1.3764 (Fig. 6 and Fig. 7). To verify the improvement effect of micro-waveguides on the sensing performance of Fabry-Perot microcavities, a waveguide free optical fiber Fabry-Perot microcavity with the same cavity length is prepared using 3D printing technology, and the structure is placed in different concentrations of sodium chloride solution in the refractive index range of 1.3346-1.3764; the linear fitting results show that the refractive index sensitivity of the microcavity structure without micro-waveguide is 115.31 nm/RIU. It is not difficult to see that with the support of a micro-waveguide, the refractive index sensitivity of the optical fiber Fabry-Perot microcavity has increased by nearly 4 times, and the peak interference spectrum has increased by 8.2 dB (Fig. 9).
Based on femtosecond laser two-photon polymerization 3D printing technology, a novel optical fiber Fabry-Perot micro-waveguides cavity integrated sensing structure is prepared in this paper. By combining the stability spectrum of fiber Fabry-Perot micro-cavity and the strong evanescent field characteristics of the optical micro-waveguide, the refractive index parameter detection with high sensitivity is realized. In the refractive index range of 1.3346-1.3764, the sensitivity is 525.81 nm/RIU, which is 3.56 times higher than that of the waveguide-free Fabry-Perot microcavity, and the peak value of the generated interference spectrum is increased by 8.2 dB. Such a fiber optic sensor with a small sample size, high sensitivity, and good repeatability will undoubtedly become a powerful booster for the rapidly developing fiber optic sensing technology and biomedical research and has a wide range of academic research value and application prospects in biodetection-related fields.
1 引言
光纤法布里-珀罗(法珀)微腔传感器因具有体积小、稳定性好等优点,被广泛应用于温度[1]、应力[2]、气压[3]、磁场[4]、折射率[5]等物理量的测量。传统的光纤法珀微腔传感器的制备方法主要有熔接法、空芯光纤填充法、化学腐蚀法。
熔接法是将单模光纤与其他光纤熔接形成一个法珀微腔[6-7]。2014年,Gao[8]在两根标准单模光纤中间熔接超细光纤,将两个单模光纤熔接端之间的开口空腔作为法珀微腔,从而构成传感器用于折射率检测。熔接法由于单模光纤与特种光纤材料的不同,熔接后容易产生大的损耗;且某些特种光纤结构强度相对较低,可能在熔接区域塌陷形成锥形结构,导致制备的传感器性能不佳。空芯光纤填充法是将聚合物或液体填充至空芯光纤腔内制成法珀传感器来实现检测[9]。2018年,Zhao等[10]利用毛细作用,在HCF内部镶嵌聚二甲基硅氧烷聚合物(PDMS)薄膜,形成带有空气微腔的光纤法珀微腔传感器用于低频声压的测量,在10~50 Hz的低频声压范围内,其灵敏度为0.427 mV/mPa。这种基于聚合物填充法制备的光学微腔灵敏度相对较高,但相比于其他制备方法,其操作相对困难,制备重复性较差。化学腐蚀通常是将光纤拼接后使用化学试剂有选择性地腐蚀光纤制成传感器[11]。2016年,Li等[12]将单模光纤与光子晶体光纤(PCF)熔接后,利用氢氟酸腐蚀PCF端面形成FP传感器并用于折射率检测,并将其应用到lambda噬菌体DNA溶液的浓度测量。化学腐蚀法具有制作难度低、成本低等优点,但化学腐蚀试剂通常易挥发,容易对人体造成伤害。
针对以上问题,本文在光纤法珀微腔干涉型传感器的微腔内引入微波导,形成集传感和导光为一体的光纤法珀微波导腔(MWC)结构。微腔可以将光场限制在微米量级的区域内,同时可以对腔内的微波导结构起支撑保护作用;微波导结构可以提升整体的导光能力,基于其强倏逝场特性可提升整体结构的折射率灵敏度。实验中,使用飞秒激光直写双光子聚合3D打印技术直接在SMF端面打印出光纤法珀MWC结构,其折射率灵敏度与仿真结果高度匹配。相比于传统的光纤法珀微腔结构,MWC结构不仅灵敏度得到提升,插入损耗也显著降低。此外,双光子聚合微纳加工技术凭借其超高的加工精度,可以保证光纤法珀MWC制备的重复性。
2 实验原理及仿真
2.1 光纤法珀微腔传感器
将光学法珀干涉仪应用到光纤传感领域,即形成了光纤法珀微腔传感器结构,通常以SMF的端面作为干涉系统的反射端面,且光束垂直入射,此时以反射光为例,其强度I可表示为
式中:I1和I2分别为微腔中的两个反射面的反射光强度;δ为I1和I2的相位差。
式中:n为光纤法珀微腔传感器中微腔内部介质的折射率;L为微腔的腔长;λ为入射光的波长;
式中:λ1、λ2分别为相邻两个干涉峰(或干涉谷)的峰值波长(或谷值波长)。从
2.2 光纤法珀MWC传输理论分析
光纤法珀MWC腔内介质均匀,可将介质视为该波导的包层。介质中的圆形微波导结构可视为结构尺寸不同的SMF,在理论上其特征方程与SMF基本一致。与普通单模光纤不同的是,微波导结构的直径与SMF纤芯直径相差较大,对折射率的变化更加敏感,其有效折射率将随着周围介质折射率的变化而改变。2020年,Zhang等[13]使用Rsoft仿真软件建立仿真模型,设置微波导直径从0.8 μm梯度增加到2.2 μm,并研究其在1.0~1.45折射率范围内的灵敏度变化,发现随着波导直径减小,光波导有效折射率对周围介质折射率变化的灵敏度明显提升[14]。在传统的光纤法珀微腔结构中引入一段空气光路或填充一段液体[15],使得光在微腔内的传输效率降低或传感灵敏度不高。为此,本文提出一种集传感和导光为一体的光纤法珀MWC结构,使得光在微腔内部沿着微波导进行传输,同时微波导的存在使得微腔对折射率的变化更为敏感。
2.3 飞秒激光双光子聚合3D打印技术
Goeppert-Mayer于1931年最先提出双光子聚合是指物质在发生双光子吸收后所引发的光聚合过程[16]。一般情况下,聚合反应只发生在入射光波长立方λ3的微小体积内,因此其加工精度可达到纳米量级。通常双光子聚合工艺应用于聚合物材料,在聚合物发生交联后再利用有机溶剂等显影液显影,得到所需要的结构。
飞秒激光双光子聚合3D打印技术(其原理图如
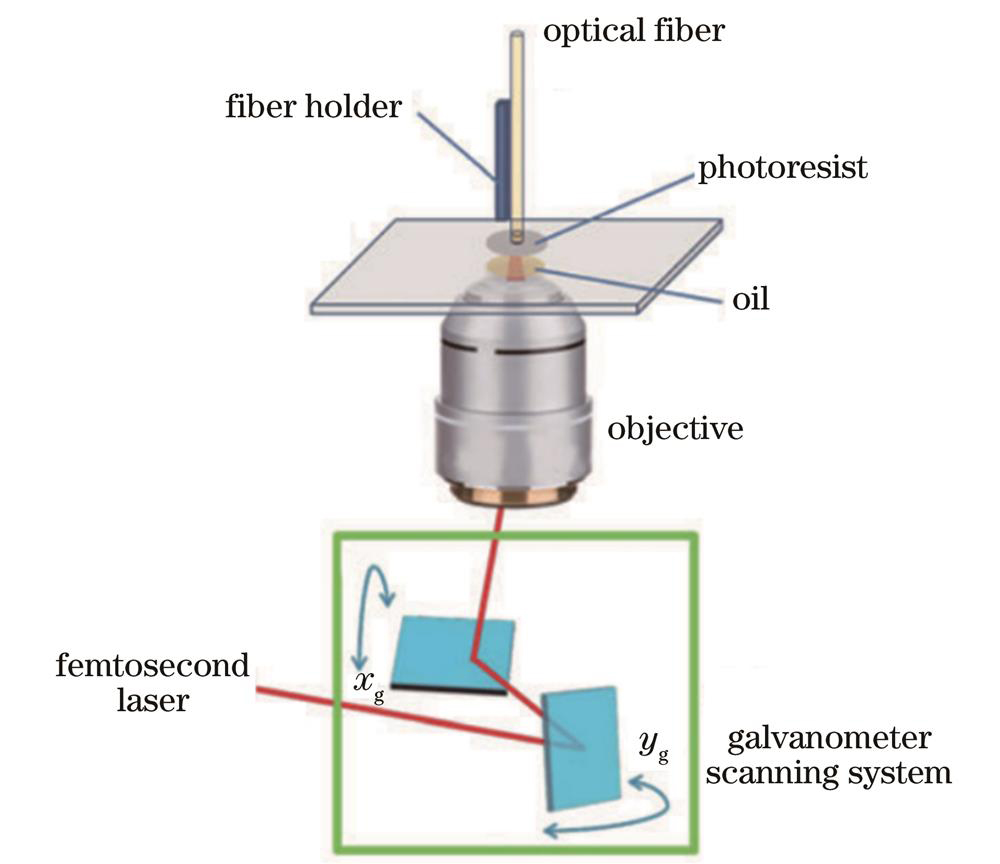
图 2. 飞秒激光双光子聚合3D打印技术原理图
Fig. 2. Schematic of femtosecond laser two-photon polymerization 3D printing technology
与传统的熔接、填充、腐蚀等工艺相比,使用双光子聚合3D打印技术制备光纤法珀微腔具有加工精度高、灵活性强、重复性好等优点,同时降低了复杂光纤传感结构对特种光纤的依赖性。此外,多样的可选择制备材料,也为基于飞秒激光双光子聚合3D打印制备不同应用场景的光纤法珀微腔提供便利。然而,双光子聚合3D打印微腔需要清洗内部光刻胶,所以微结构不能是封闭式的,这特点也可被巧妙应用于特殊场合的参数检测,比如2021年,Chen等[20]利用PVA材料的折射率随湿度变化来实现湿度检测,城堡型镂空壁增加湿敏材料与水分子的接触面积,提升传感器响应速度与灵敏度。
2.4 仿真建模分析
在本结构设计中,光纤法珀MWC是核心元件,承担着光信号的传输与获取任务,因此其光学传感特性将直接决定传感器的性能。为建立光纤法珀MWC理论模型,首先需要基于光学法珀干涉原理建立理论模型,同时为了更精确地验证结构的可行性,通过仿真软件建立仿真模型,使用有限时域差分(FDTD)法[21]与有限元分析法(FEM)[22]递推模拟波在时域中的传播过程以及场的分布,并通过模拟光纤法珀MWC的干涉光谱,研究当微波导的长度、直径、形状、有效折射率变化时,光纤法珀微腔干涉光谱的移动情况,从而优化光纤法珀MWC的结构设计。
微波导尺寸较小,对加工精度要求较高,因此利用传统的制备方式很难实现。基于飞秒激光双光子聚合的3D打印技术[23]可以灵活、高精度地制备各种传感器。打印所需材料为光刻胶。经双光子吸收后的光刻胶折射率为1.52~1.54,设置仿真结构的折射率为1.53,背景折射率的变化范围为1.333~1.337,设置光纤法珀MWC腔长为50 μm,锥形波导长度为20 μm,锥腰底直径为8.2 μm、顶直径为2 μm,微波导直径为2 μm,光纤法珀MWC干涉光谱的折射率响应和折射率灵敏度拟合结果分别如

图 3. 光纤法珀MWC光谱随折射率的变化
Fig. 3. Spectra of optical fiber Fabry-Perot MWC changed with refractive index
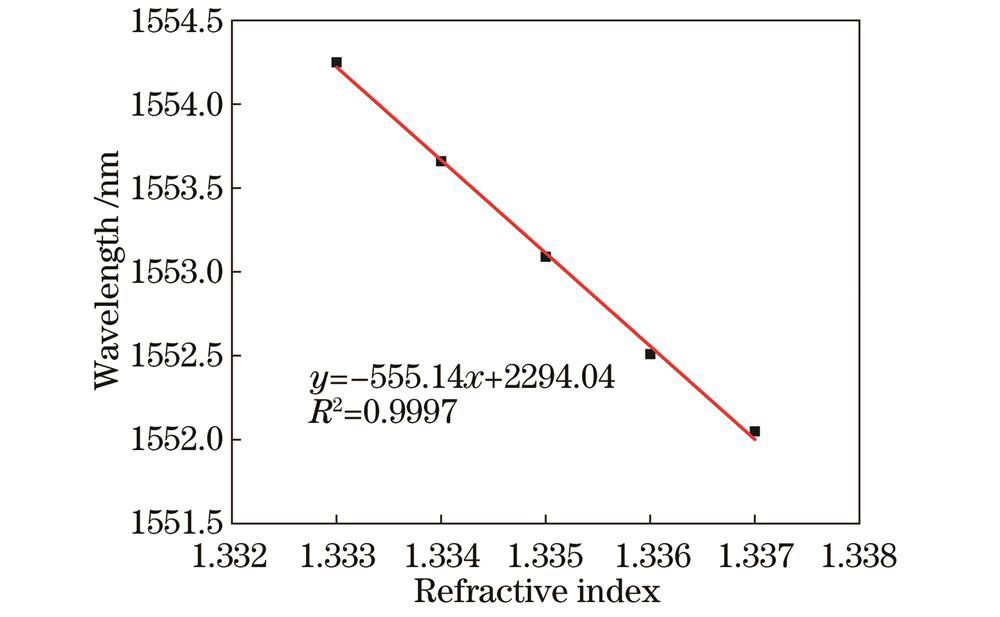
图 4. 光纤法珀MWC折射率灵敏度线性拟合结果
Fig. 4. Linear fitting results of refractive index sensitivity of optical fiber Fabry-Perot MWC
3 传感器制作及实验验证
3.1 光纤微波导腔结构制备及折射率测量
为研究波导结构对传感效果的提升,设计并制备光纤法珀MWC结构。为确保微波导能快速感知外界折射率的变化,微腔采用侧壁镂空的开放式结构,
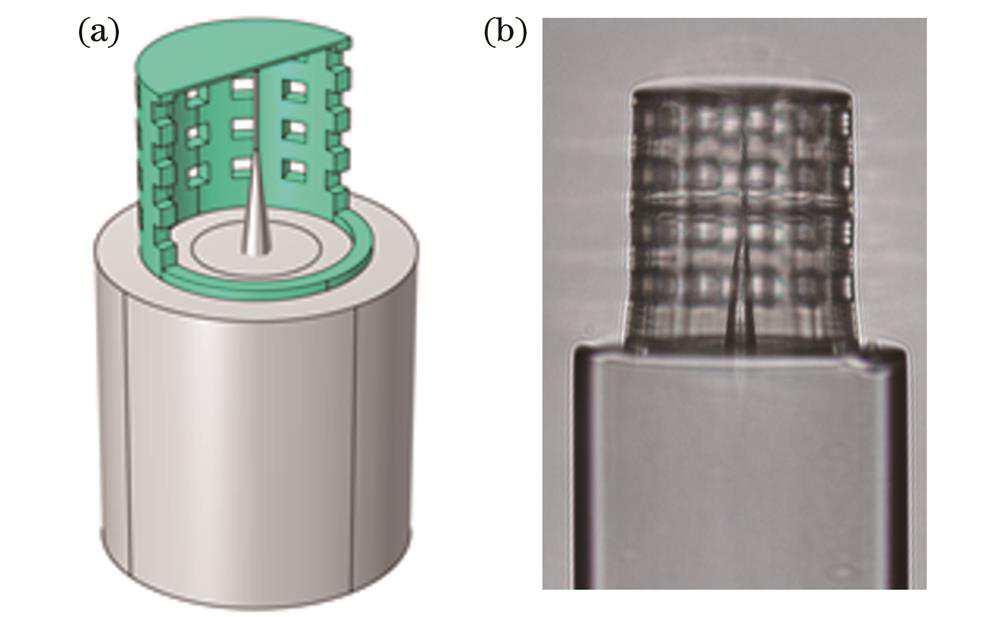
图 5. 光纤法珀MWC结构。(a)示意图;(b)实物图
Fig. 5. Structure of optical fiber Fabry-Perot MWC. (a) Schematic; (b) physical diagram
光纤法珀MWC结构制备过程主要分为以下三步:1)打印端面处理。取一根SMF,剥除涂覆层,使用乙醇溶液擦拭干净;使用切割刀将光纤端面切割平整,将切割后的SMF放置在熔接机中观察,并对SMF端面放电清洁;将处理后的SMF放置在夹具中,并将夹具固定在打印设备。2)镜头聚焦与纤芯位置确认。调整镜头位置,将镜头聚焦到光纤端面,使用650 nm可见光激光光源进行补光处理,并使用微位移平台摇杆调节光纤端面位置。所聚焦光斑的位置即为光纤纤芯的位置。调节微位移平台,将光纤端面调节至打印设备的中心位置。3)结构打印。调节激光强度、频率等参数,导入打印模型图并开始打印。打印时需要实时观察打印的结构是否处于光纤端面的几何中心,是否发生倾斜、断裂等。
打印方式为增材打印,光波导结构与微波导腔是同时从结构底部(单模光纤端面)向顶部打印,打印过程完全同步能保证结构更加稳定。打印结束后,将固定光纤的夹具从打印设备中取下,将打印结构固定到载玻片上。将光纤悬空放置在载玻片上并固定。将载玻片先后插入丙二醇甲醚醋酸酯溶液和异丙醇溶液中清洗。待结构干燥后,将其放置在显微镜下观察,实物图如
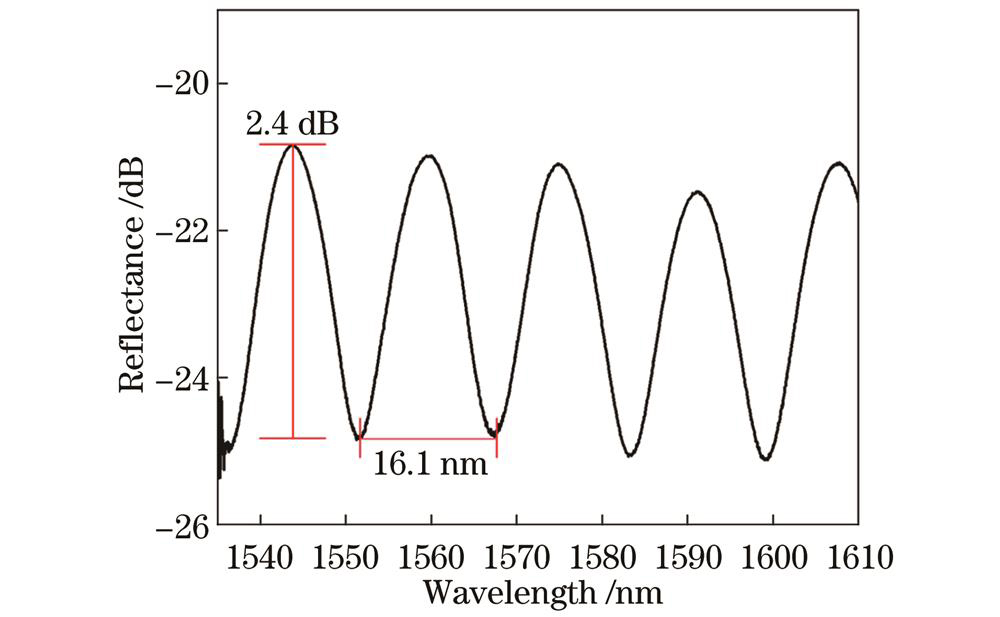
图 6. 光纤法珀MWC结构在去离子水中的反射光谱
Fig. 6. Reflection spectrum of optical fiber Fabry-Perot MWC in deionized water

图 7. 光纤法珀MWC结构反射光谱的傅里叶变换结果
Fig. 7. Fourier transform result of reflection spectrum of optical fiber Fabry-Perot MWC
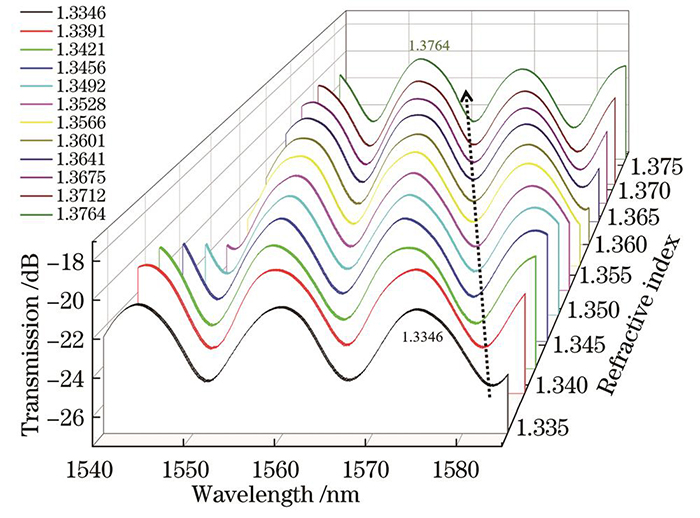
图 8. 1.3346~1.3764折射率范围内的干涉光谱变化情况
Fig. 8. Interference spectrum shifts within refractive index range of 1.3346-1.3764
将MWC结构分别放在折射率在1.3346~1.3764范围的12种不同浓度的氯化钠溶液中进行实验,
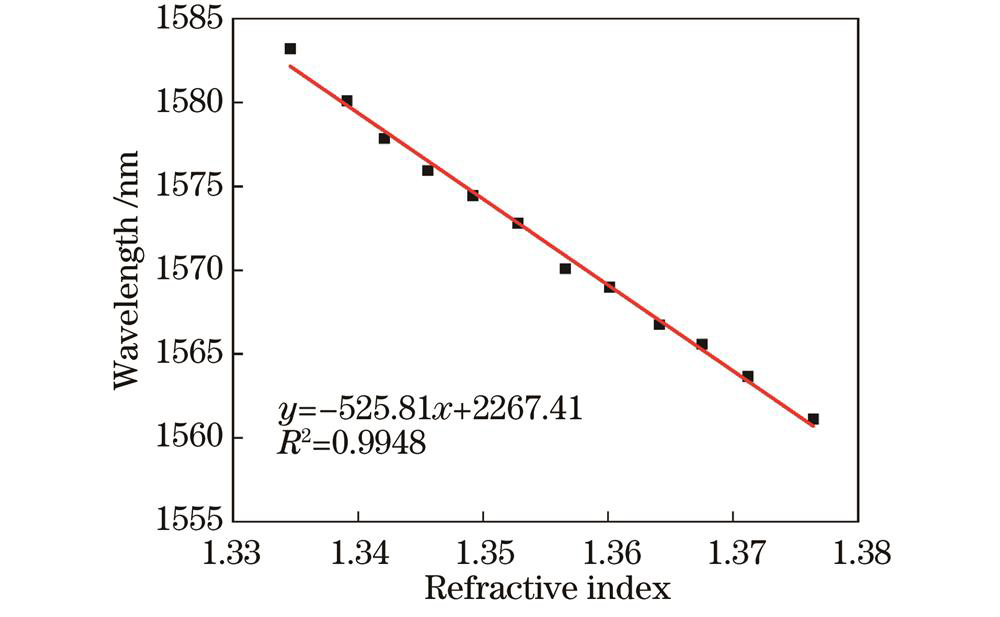
图 9. 基于双光子聚合技术制备的光纤法珀MWC结构在不同折射率溶液下的反射光谱线性拟合结果
Fig. 9. Linear fitting results of reflection spectra of optical fiber Fabry-Perot MWC prepared by two-photon polymerization technology under different refractive index solutions

图 10. 可重复性实验所用光纤法珀MWC结构在不同折射率溶液下的反射光谱线性拟合结果
Fig. 10. Linear fitting results of reflection spectra of optical fiber Fabry-Perot MWC structure used in repeatability experiments under different refractive index solutions
3.2 无微波导法珀微腔结构的折射率传感特性
为了验证光纤法珀MWC对传感结构的性能提升效果,使用双光子聚合3D打印技术制备了腔长同样是50 μm的无微波导法珀微腔结构。将该结构放置在折射率为1.3346~1.3764的5种不同浓度的氯化钠溶液中,并进行传感测量。

图 11. 无微波导法珀微腔结构在不同折射率溶液下反射光谱,插图为无微波导法珀微腔结构示意图
Fig. 11. Reflection spectra of waveguide-free optical fiber Fabry-Perot microcavity structure in different refractive index solutions,and the illustration shows microcavity structure without micro-waveguide

图 12. 无微波导法珀微腔结构的折射率灵敏度线性拟合结果
Fig. 12. Linear fitting results of refractive index sensitivity for Fabry-Perot microcavity structure without micro-waveguide
4 结论
基于飞秒激光双光子聚合的3D打印技术制备了一种新型光纤法珀微波导腔一体化传感结构,结合光纤法珀微腔的稳定性光谱与微波导的强倏逝场特性,实现了高灵敏度的折射率参数检测,在1.3346~1.3764折射率范围内灵敏度为525.81 nm/RIU,与无波导法珀微腔相比,灵敏度提升了3.56倍,生成的干涉光谱峰值提升了8.2 dB。这样一种样本量需求小、灵敏度高、可重复性好的光纤传感器,无疑将成为正在迅速发展的光纤传感技术、生物医学研究的强力助推剂,在生物检测相关领域具有较大的学术研究价值和良好的应用前景。
[1] 谢佳一, 苏国帅, 李明宇, 等. 反射型法布里-珀罗腔和微环谐振腔级联的温度传感器[J]. 光学学报, 2022, 42(23): 2328002.
[2] 王旗, 邹辉, 韦玮. 基于偏芯熔接光纤的应力与折射率传感器[J]. 光学学报, 2017, 37(10): 1006005.
[3] Li Z, Zhang Y X, Zhang W G, et al. High-sensitivity gas pressure Fabry-Perot fiber probe with micro-channel based on vernier effect[J]. Journal of Lightwave Technology, 2019, 37(14): 3444-3451.
[4] Sun H Z, Jiang C Q, Tang J R, et al. High sensitivity optical fiber magnetic field sensor based on semi fixed extrinsic Fabry-Perot interferometer[J]. Optical Fiber Technology, 2022, 70: 102890.
[5] 武洁雅, 康娟, 董洁, 等. 基于PDMS水滴型光纤挥发性有机化合物传感器[J]. 光学学报, 2022, 42(21): 2128001.
[6] 徐廷廷, 杨玉强, 杨文龙, 等. 基于PDMS膜封装空芯光纤的级联双腔温度传感器[J]. 光学学报, 2022, 42(8): 0806004.
[7] Lü R Q, Li S Q, Wang W, et al. Temperature characterization of thin-walled-microsphere air-cavity fiber sensing structures[J]. Sensors and Actuators A, 2023, 349: 114081.
[8] Gao S C, Zhang W G, Bai Z Y, et al. Microfiber-enabled in-line Fabry-Pérot interferometer for high-sensitive force and refractive index sensing[J]. Journal of Lightwave Technology, 2014, 32(9): 1682-1688.
[9] 王超, 冯国英, 陈晓旭, 等. 空芯光子晶体光纤的塌缩及选择性液体填充[J]. 激光与红外, 2019, 49(9): 1119-1123.
Wang C, Feng G Y, Chen X X, et al. Fabrication of selective injection microstructured optical fibers[J]. Laser & Infrared, 2019, 49(9): 1119-1123.
[10] Zhao Y, Chen M Q, Xia F, et al. Small in-fiber Fabry-Perot low-frequency acoustic pressure sensor with PDMS diaphragm embedded in hollow-core fiber[J]. Sensors and Actuators A, 2018, 270: 162-169.
[11] Wu S N, Yan G F, Lian Z G, et al. An open-cavity Fabry-Perot interferometer with PVA coating for simultaneous measurement of relative humidity and temperature[J]. Sensors and Actuators B, 2016, 225: 50-56.
[12] Li J C, Qiao X G, Wang R H, et al. Temperature-independent refractometer based on fiber-optic Fabry-Perot interferometer[J]. Optics and Lasers in Engineering, 2016, 79: 16-21.
[13] Zhang D W, Wei H M, Hu H Z, et al. Highly sensitive magnetic field microsensor based on direct laser writing of fiber-tip optofluidic Fabry-Pérot cavity[J]. APL Photonics, 2020, 5(7): 076112.
[14] Reghuprasad A E, Colombero C, Godio A. Serially connected cantilever beam-based FBG accelerometers: design, optimization and testing[J]. Sensors, 2023, 23(6): 3188.
[15] Wang J, Liu Z Y, Gao S R, et al. Fiber-optic anemometer based on Bragg grating inscribed in metal-filled microstructured optical fiber[J]. Journal of Lightwave Technology, 2016, 34(21): 4884-4889.
[16] Göppert-Mayer M. Elementary processes with two quantum transitions[J]. Annalen Der Physik, 2009, 18(7/8): 466-479.
[17] Chen M Q, He T Y, Zhao Y. Review of femtosecond laser machining technologies for optical fiber microstructures fabrication[J]. Optics & Laser Technology, 2022, 147: 107628.
[18] Chen Q D, Wu D, Niu L G, et al. Phase lenses and mirrors created by laser micronanofabrication via two-photon photopolymerization[J]. Applied Physics Letters, 2007, 91(17): 171105.
[19] 赵圆圆, 罗海超, 梁紫鑫, 等. 光聚合微纳3D打印技术的发展现状与趋势[J]. 中国激光, 2022, 49(10): 1002703.
[20] Chen M Q, Zhao Y, Wei H M, et al. 3D printed castle style Fabry-Perot microcavity on optical fiber tip as a highly sensitive humidity sensor[J]. Sensors and Actuators B, 2021, 328: 128981.
[21] Singh L, Agrawal N, Saha C, et al. A plus shaped cavity in optical fiber based refractive index sensor[J]. IEEE Transactions on NanoBioscience, 2022, 21(2): 199-205.
[22] Zhang P, Wang S, Jiang J F, et al. A fiber-optic extrinsic Fabry-Perot hydrophone based on Archimedes spiral-type sensitive diaphragm[J]. IEEE Sensors Journal, 2022, 22(23): 22654-22660.
[23] Hadibrata W, Wei H M, Krishnaswamy S, et al. Inverse design and 3D printing of a metalens on an optical fiber tip for direct laser lithography[J]. Nano Letters, 2021, 21(6): 2422-2428.
Article Outline
陈茂庆, 刘思源, 蔡露, 刘强, 赵勇. 基于双光子聚合3D打印的光纤法珀微波导腔制备及传感特性研究[J]. 光学学报, 2024, 44(2): 0206002. Maoqing Chen, Siyuan Liu, Lu Cai, Qiang Liu, Yong Zhao. Fabrication and Sensing Characteristics of Optical Fiber Fabry-Perot Micro-Waveguide Cavity Based on Two-Photon Polymerization 3D Printing[J]. Acta Optica Sinica, 2024, 44(2): 0206002.